Cucumber production in Florida in 2007–2008 was divided between the spring season, 58% of the total, and the fall season, 42% of the total (Florida Agriculture Statistical Bulletin 2009). Crop value for 2007–2008 was $94,433,000. Acreage planted to cucumber increased from 9,400 acres in 1997 to 15,700 in 2008. While cucumber acreage increased during this 11-year period, the recorded average yield per acre decreased from 560 to 509 55-lb bushels/acre.
Fertilizer is a major part of the crop production expenses for cucumber, and it is critical for successful crop yields and high fruit quality. This document is not meant to present new fertilization recommendations but rather to update the previously published research review by the same title, including new research conducted in the last decade. Current cucumber fertilization recommendations are based on published field research. The compilation of literature contained in this document will assist in making valid fertilizer recommendations that are commercially viable while also reducing the risk of environmental consequences in adjacent water bodies. As environmental regulation becomes more commonplace, it becomes more important that fertilization recommendations are based on published research.
This publication documents the previous written literature, revisions of which now appear only in updated, modern electronic format. All publications, including the reviewed older paper-format publications, will be placed in PDF on the website https://bmp.ifas.ufl.edu for future reference.
Fertilizer recommendations include the recommended rate of fertilizer and also management strategies needed to maximize the fertilizer investment while protecting the environment. These principles for fertilization of vegetables are summarized by Hochmuth and Hanlon (2010a). Rate is only a part of the modern fertilizer recommendation. Sound fertilizer recommendations also consider, among other aspects, fertilizer materials, irrigation, placement, and timing of application (Hochmuth and Hanlon 2010b).
More than 40 years worth of cucumber fertilization research has been conducted in Florida. During this time, many changes have occurred in cucumber production practices, including changes in cultivars and the introduction of new cultural systems like polyethylene mulch and drip irrigation. Cucumber crop and fertilizer management recommendations, such as plant and row spacing, have changed with time in keeping with new developments in research (Hochmuth and Maynard 1996; Montelaro 1978; Hochmuth 1988a, b; 1995; 1996a: 1996b; Hochmuth and Hanlon 1989, 1995a; 1995b; 2000; Hochmuth and Maynard 1995; Hochmuth and Smajstrla 1997; Kidder et al. 1989; Kostewicz and Cantliffe 1975; Marlowe and Montelaro 1977; Olson and Santos 2010; Olson et al. 2010; Simonne and Hochmuth 2010). The most current recommendations for nutrient management in cucumber production are presented in the Commercial Vegetable Production Handbook for Florida (Olson and Santos 2010). The University of Florida's Institute of Food and Agricultural Sciences (IFAS) recommends a target of 150 lb/acre N, plus 120 lb/acre P2O5 and 120 lb/acre K2O (only when soil concentrations of P or K are "very low" based on results of Mehlich-1 (M-1) soil testing) (Hochmuth and Hanlon 1995a; 2000; Mylavarapu 2009; Olson et al. 2010). More N can be applied to replace leached N or for an extended harvest season (Simonne and Hochmuth 2010). These recommendations have been in effect for the last decade, after being revised from 90-120-120 in 1977 (Marlowe and Montelaro 1977; Montelaro 1978) to 120-120-120 N-P2O5 in 1989 and then to 150-120-120 in 1997 (Hochmuth and Hanlon 1989; 1995b; Hochmuth and Cordasco 1999). Despite the recommendations, commercial growers often apply higher rates to reduce the risk of yield reductions due to unfavorable production conditions.
Early research focused largely on yield and fruit quality (size and shape) in response to fertilization. Since the early research was conducted, and since the first review of the literature in 1999, there have been increasingly strong interests in water quality, and this component has been included in fertilizer research. The state (Fla. Dept. of Agr. and Cons. Serv. 2005; Simonne and Hochmuth 2010) has been formalizing Best Management Practices (BMPs) and encouraging growers to implement them. The definition of a BMP includes both economic and environmental components and also states that BMPs must embody the best available science.
This literature review includes all available published documentation concerning cucumber yield and fertilizer use in Florida since 1960. We chose to present all the research without being selective, as that would introduce bias. Inclusion of all Florida literature shows the history of commercial production system development with respect to fertilizer use. Commercial yields have been increasing primarily due to selection of new cultivars with traits that resist disease and pest pressures and more intensive production practices like plastic mulched beds. Since the basic plant has not changed, plant nutrient requirements have also been slow to change. In fact, nutrient use efficiency has increased while commercial fertilizer rates have not. Fertilizer recommendations have, however, been increased to reflect the increasing productivity of the cucumber crop.
Since nutrient and water management are linked, fertilization research is summarized by irrigation method. The fertilization recommendation addresses commercial yield and quality, the economics of crop production, and protection of the environment. Equally important is to have a mechanism available to the grower to adjust fertilization practices during the season due to leaching rains and extension of the growing season for additional harvests when market conditions are favorable. To address all of these concerns, UF/IFAS vegetable recommendations are given as a single target fertilizer rate that is projected to be sufficient for meeting the crop fertilizer needs for most growing seasons. This single target fertilizer rate is a recommended starting point and has been used historically in all vegetable fertilizer recommendations in Florida, as well as around the country. The target recommendation approach with footnotes was used by Montelaro (1978) and confirmed by Hochmuth and Hanlon (1995; 2000). The target value was derived from numerous fertilizer studies and represents a reasonable fertilizer rate that reflects the average maximum crop responses from all of the fertilizer research, not the extremes in responses. However, the recommendation process recognizes some growing seasons are different, due to more leaching rains or increases in crop value that can lead to prolonged harvest windows. Hence, the target fertilizer amount is accompanied by a series of footnotes that explain the addition of supplemental fertilizer during the growing season, which includes addressing leaching rains and additional harvests near the end of the season. It is logical to select a single target rate based on research that avoids excessive fertilizer applications that often reduce crop nutrient efficiency and increase the potential for environmental degradation.
This publication is an updated version of a previous research literature review by Hochmuth and Cordasco (1999; reviewed 2009) that covered fertilizer research through 1996. This new document adds the research reports conducted since 1996 and expands on the role of irrigation in managing fertilizer for crop production efficiency and environmental protection. The audience for this publication includes educators (e.g., Extension specialists and agents), commercial vegetable producers, consultants, and governmental agencies.
Data Summary Method
Most authors chose to express fertilizer rates on a per-acre basis, irrespective of variations in bed spacing among reports or experiments. Authors of a few reports chose to use the linear-bed-foot system to standardize fertilizer-rate expressions across experiments and planting patterns. In this report, we attempt to specify planting patterns and fertilizer rates for each experiment as far as we could determine. Current fertilizer recommendations for cucumber are based on a 6-foot bed spacing with two plant rows per bed.
Evaluation of cucumber yield response to varying rates of applied fertilizer required a standardized method of summarizing statewide yields, which were expressed variably as kg/ha, lb/acre, Mg/ha, tons/acre, cwt/acre, and cartons or boxes per acre (55 lb box). In addition, vegetable yields vary depending on season, cultivar, and location in the state. Relative yield (RY), a calculated percentage, was chosen as the unit to express cucumber yield response to fertilization. Relative yield is an accepted scientific method for summarizing and presenting data across wide sources and reports (Brown 1987).
In his book, C. Black (1992) summarized the advantages and disadvantages of the RY approach. There are valid statistical concerns about RY, but he concluded that, when applied properly and cautiously, the RY approach can be useful in displaying general relationships. Black demonstrated examples in his book where RY yield was helpful and where it was not. Plotting absolute yield data in original units obviously would result in a scatter of data, rendering any general interpretation impossible. Black (1992) pointed out that decisions can always be improved with further research, but the data on hand are the best we have at the time. The highest yield for each fertilizer experiment was assigned a 100% value, and other yields were expressed as a percentage of the highest yield. The actual yield corresponding to 100% RY was presented in the number of 55-lb boxes/acre, or the original units were given. The RYs were plotted against rates of nutrient to determine how cucumber yields responded to fertilizer in Florida. The RY presentation allowed data from a variety of experiments to be included in the graphical summary of yield responses without making arbitrary decisions about what data to include and what data not to include. For most studies, RYs of 90 to 100% were not significantly different.
Sometimes the argument is made that growers have expectations of greater yields than those obtained in research projects. It should be pointed out that realistic, regularly obtainable yields by growers are often considerably less than expected yields or "yield goals." Research on this subject has documented that 20% of growers actually reached their stated yield goals, and only 50% reached 80% of their stated yield goals (Schepers et al. 1986). Therefore, growers rarely achieve their stated yield goal, which means fertilizer might be wasted. Therefore, fertilizer rates should not be set on yield goals but rather on realistic goals based on research and actual farm records. The practice of using yield goals to set fertilizer rates is not recommended in Florida, so the effect of overfertilization has been avoided by grounding expectations in measurement of observed yields. In effect, the current Florida fertilizer recommendation includes a component that addresses yield expectation versus actual production capability concern expressed by growers.
Scientists throughout the years have conducted many replicated demonstration studies using true commercial production practices in growers' fields, and those studies are included in this review. Excessive fertilizer was justified in the past when fertilizer was viewed as inexpensive insurance against yield loss. Research with numerous crops has shown that nutrient use efficiency declines as nutrient rate, especially N, increases. Even given the best of production systems, N use efficiency rarely exceeds 70% of the applied N. Concepts and practices for managing nutrients in vegetable production were summarized by Hochmuth (1992a, b; 2000). Further, it has been suggested that yields from older studies were much lower than yields obtained today. However, there are older research reports in the last two decades where yields were as high as yields achieved today.
In this review, the highest yield for each fertilizer experiment was designated as 100%, and other yields were expressed as a percentage of the highest yield. The commercial weight of a box (1 and 1/9 bu) of cucumber (typically 55 lb) was used, with all data presented for the actual treatment corresponding to 100% RY. While fertilizer rate is reported in pounds per acre (lb/acre), the reader should keep in mind that the bed system used in each field may vary and require adjustments to the fertilizer rate. To address this issue, the linear bed foot concept can be used to convert among the various bed designs with and without spray rows or ditches (Hanlon and Hochmuth 1989; Hochmuth and Hanlon 2009). Additional cultural practices for commercial cucumber production can be found in Chapter 2 of the Vegetable Production Guide (Simonne and Hochmuth 2010).
Keeping available nutrients in the soil profile for cucumber plant uptake requires proper irrigation management. Because of the critical nature of irrigation and drainage management with respect to nutrient uptake and possible loss of nutrients to the environment, information is regularly updated (Simonne et al. 2010).
Another useful tool to measure nutrients and that contributes to appropriate management of fertilizer is plant tissue and/or plant sap sampling. For interpretation of plant and petiole sap testing values, see Hochmuth (1994 a, 1994b) and Hochmuth et al. (1991b; 2009).
Mixed-Fertilizer Trials
Early cucumber research established crop yield responses to rates of applied nutrient and to fertilizer application methods through the use of mixed N-P-K fertilizers (Figure 1). Attributing crop response to a single nutrient, however, could not be done with certainty from a blended N-P-K material. Since N was usually the most limiting nutrient in sandy soils, yield responses in mixed studies are considered here as responses to N.
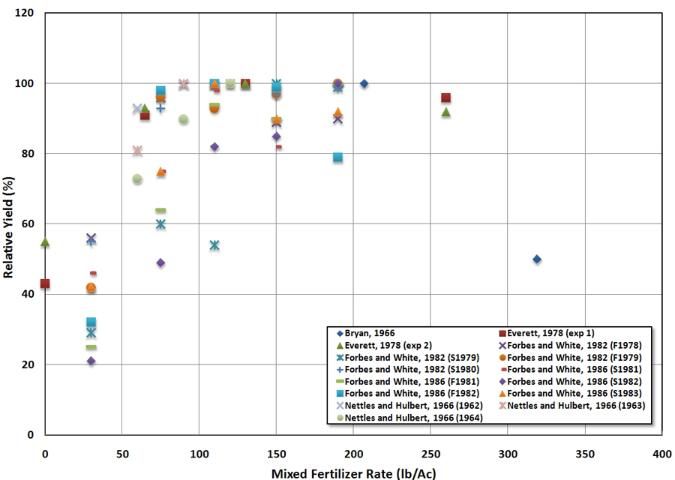
Cucumbers treated with a 30% organic 4-8-8 (N-P2O5-K2O) fertilizer produced higher yields in all three seasons of an Immokalee experiment compared with cucumbers fertilized with inorganic, 4-8-8 (N-P2O5-K2O) fertilizers with or without added minor elements (Everett 1963). Subsurface irrigation was used in all experiments. Benefits associated with organic fertilizer include its ability to stabilize soil pH, act as a microbial medium, act as a source of soluble organic compounds and minor elements, improve water and nutrient holding capacity, and resist N leaching. With application of minor elements and chelating agents, researchers sought to increase yields of plants fertilized with inorganic fertilizers to those of plants fertilized with the 30% organic fertilizer. These comparison studies resulted in unexpected responses from plants fertilized with the mixed organic fertilizer and raised additional questions as inorganic fertilizer sources became more widely used.
As a base of comparison, an inorganic fertilizer containing NH4NO3 and a 30% organic fertilizer containing Peruvian guano and biosolids as 30% of the total N (a 4% N product) were applied without minor elements in 1960 (Everett 1963). Three N applications of 40 lb/acre each were made from either the inorganic or organic source, and an additional 86 lb/acre NO3-N were applied in six 14 lb/acre top-dress applications to all base fertilizer treatments. Plants were thinned to one per hill with 18 inches between hills, and subsurface irrigation was applied as needed. Beds were spaced 5 feet apart on centers. Yield from plants fertilized with the inorganic N source was 111 bushels/acre while yield with the organic N source was 760 bushels/acre; the bushel weight was not specified. Differences in yield were traced to concentrations of Fe, Cu, Mn, B, and Al in the leaf tissue. Although soil concentrations of N, P, K, Ca, and total soluble salts were similar with both treatments, plants fertilized with inorganic fertilizer had six times more Al and lower concentrations of Fe, Cu, Mn, and B compared with tissue from organic-N-fertilized plants. Based on high Al concentrations in the leaf tissue, marginal leaf burn, and stunted plants observed at three weeks and throughout the season, aluminum toxicity was suspected in the inorganic-fertilized plants. Researchers theorized that other micronutrients present in the organic fertilizer provided sufficient competition to prevent Al absorption, or a natural chelating agent present in the organic mix decreased Al absorption.
In 1961, the same organic and inorganic 4-8-8 fertilizer sources were used plus a third 4-8-8 formulation of 30% N from NH4NO3 and 70% N from NH4NO3, (NH4)2SO4, and ammonium superphosphate (Everett 1963). These nutrients were applied as before with four additional top-dress applications each of 14 lb/acre of NO3-N. Fritted (molten glass) FN-501 micronutrients were applied with half of each fertilizer treatment at the time of the first 40 lb/acre N application. Cucumber plants were thinned to two per hill. Similar yields resulted, with or without application of minor elements, for plants fertilized with the organic source (1,005 bushels/acre) or the inorganic, NH4NO3-N source (812 bushels/acre). Yields increased significantly (1% probability) with addition of micronutrients to the third fertilizer formulation, from 788 bushels/acre without micronutrients to 908 bushels/acre with micronutrients. Leaf-tissue concentrations of Cu and Fe were highest in plants fertilized with the organic source, were unaffected by micronutrients in NH4NO3-fertilized plants, and were increased when micronutrients were applied with the third fertilizer formulation (Mn leaf-tissue concentration increased also). A change in plot location with this experimental season appeared to eliminate Al-toxicity symptoms noted in the previous season.
The mixed organic and NH4NO3 fertilizers were applied in three 40 lb/acre N applications as before, with an additional 40 lb/acre applied due to heavy rain and two 14 lb/acre NO3-N side-dress applications (Everett 1963). An interaction effect on marketable yield occurred this season between the addition of a chelating agent (a solution of 0.12 g Na-EDTA/ml), added micronutrients Fe or Cu applied in chelated or soluble form, and fertilizer sources. All treatments were applied as solutions in an 18-inch-wide band sprayed on top of the first fertilizer application and bedded to a depth of 3 inches. Plants fertilized with the organic fertilizer source and the chelating agent alone or chelated Fe or Cu resulted in an average yield of 1,004 bushels/acre. This yield was significantly higher than where organic fertilizers were applied without the chelating-agent solution or where Fe or Cu was applied in a soluble form (918 bushels/acre). Yields of plants fertilized with the inorganic NH4NO3 fertilizer, however, were not affected by chelated or nonchelated treatments, which averaged 686 bushels/acre. The stimulating effect of the chelating agent among plants fertilized with organic fertilizers was not explained by the authors, and more research was recommended. Nitrogen and K leaching were not a factor in any season, as leaf-tissue concentrations of these nutrients were comparable for plants with either fertilizer treatment.
Application of 15 tons/acre of fresh poultry manure on Norfolk loamy fine sand in Quincy contained sufficient N to sustain high cucumber yields in the spring of 1960 (Robertson and Young 1964). Cucumber yield with the base manure application and 0 lb/acre of applied soluble N did not differ compared with yields in which plants received 30, 60, 90, 120, or 150 lb/acre N applied in a single side-dress application. Bed centers were spaced 4 feet apart. The average marketable yield of 326 bushels/acre was considered good based on an average-to-good yield range of 135 to 436 bushels/acre for cucumbers grown in the U.S. at the time. Irrigation was applied at this site, but the type of irrigation was not cited.
Fertilizer treatments of 207-118-236 lb/acre N-P2O5-K2O and 319-187-366 lb/acre N-P2O5-K2O were used in an early study (Bryan 1966). Fertilizers were applied to Ruston loamy fine sand soils at the North Florida Experiment Station in Quincy in bands 8 inches to each side of the bed center; covered with soil; and mulched with clear, aluminum (black polyethylene sprayed with aluminum paint), or black polyethylene mulch. Beds were spaced on standard 6-foot centers. Yields with these treatments were compared with yields of nonmulched plants fertilized with a base and subsequent side-dress fertilizer applications. The nonmulched beds and beds mulched with clear polyethylene received herbicide application. Polyethylene mulch had not been widely used in North Florida when this 1965 experiment was conducted. Irrigation was not described in this study.
Plants fertilized with 207 lb/acre N yielded 307 bushels/acre (100% RY) compared with 50% RY from plants fertilized with 319 lb/acre N (Bryan 1966). Yields were highest from plants mulched with clear polyethylene (324 bushels/acre), followed by those mulched with aluminum (282 bushels/acre), black polyethylene (171 bushels/acre), and nonmulched plants (142 bushels/acre).
Improvements in equipment used to apply fertilizer prompted fertilizer placement studies on nonmulched cucumbers in Gainesville from 1962 to 1966 (Nettles and Hulburt 1966). Applied N treatments from 6-8-8 (N-P2O5-K2O) were 60 or 90 lb/acre in 1962 and 60, 90, or 120 lb/acre in 1963 and 1964. Cucumbers were grown as a fall crop on Arredondo fine sand soil in 1962 and 1964 and on Kanapaha fine sand in 1963. There were five fertilizer placement methods. High-band fertilizer placements were 2.5 inches to one or both sides of the row and 2.5 inches below the seed level. Low-band placements were 2.5 inches to the side and 6 inches below the seed. Where high- and low-band placements were used together, the bands were placed on opposite sides of the plant row. Bed spacing and the type of irrigation used were not specified.
In 1962, half of the total fertilizer was applied in the various placement methods and half was applied as a side-dressing 30 days after planting (Nettles and Hulburt 1966). Plants fertilized with 90 lb/acre N yielded 223 bushels/acre (100% RY), and similar yields resulted from 60 lb/acre N (93% RY). Marketable yields did not differ when the fertilizer was halved and applied in single or double high bands, broadcast and incorporated in an 18-inch band and in double high bands, split evenly between a high and a low band, or when a fourth was applied in a high band and three-fourths in a low band. Marketable yields with these placement methods averaged 225 bushels/acre.
Cucumber plants in the 1963 experiment season received all of the applied fertilizer in the various placement methods at planting (Nettles and Hulburt 1966). Marketable yields with 90 lb/acre N were 491 bushels/acre (100% RY), a significant increase from 81% RY with 60 lb/acre N. Yield remained at 100% RY with the 120 lb/acre N treatment. Fertilizer placement method had an effect on marketable yield this season. Higher yield resulted when plants received fertilizer in double high bands, broadcast and in double high bands, or half in high and half in double low bands. These treatments resulted in an average marketable yield of 511 bushels/acre compared to 423 bushels/acre from plants fertilized in a single high band or plants that received one-fourth of the fertilizer in a high band and three-fourths in a low band.
In 1964, fertilizer was applied either all at planting or 80% at planting plus 20% side-dressed (Nettles and Hulburt 1966). N application timing interacted with N rate this season. Marketable yields increased through 120 lb/acre N (140 bushels/acre, 100% RY) when fertilizer was split-applied but did not increase with N when all of the fertilizer was applied at planting; similar yields resulted with 90 and 120 lb/acre N (99 bushels/acre, 99 and 100% RY, respectively). Heavy rains between planting and side-dress fertilizer application resulted in erosion and loss of one of the four experimental replications, likely reducing overall yield that season.
Two spring experiments were conducted on previously mulched beds following a fall tomato crop in order to evaluate fertilizer rates and placement methods in a two-crop system (Everett 1978). Tomatoes grown in the fall (first planting) were nonstaked "ground" tomatoes. Fertilizer was placed in a hole punched through the polyethylene at rates of 0, 0.5, 1.0, 2.0, or 4.0 ounces per hole. Fertilizer holes were 8 inches to one side of the plant, 8 inches to both sides of the plant, or halfway between the plants (in the "drill"). Nitrogen treatments of 0, 65, 130, or 260 lb/acre (18-0-25, N-P2O5-K2O) were calculated based on 5,808 plants/acre (5-foot row spacing). Fields were subsurface irrigated to maintain soil moisture at field capacity before planting the spring cucumber crop (3 weeks after removing fall-season plants).
Cucumber yield increased significantly with the first increment of applied fertilizer to 91% and 93% RY each season. Yield increases were not significant with N treatments greater than 65 lb/acre. Each season, 100% RY occurred with 130 lb/acre N (780 and 801 bushels/acre). Fertilizer placement did not affect cucumber yields in either experiment.
Researchers chose old vegetable-production lands in Sanford, Florida, (Central Florida Research and Education Center [CFREC], now the Mid-Florida Research and Education Center) for several successional, spring and fall experimental seasons to trace residual nutrient concentrations and establish the optimum side-dress N rate for pickling cucumber (Forbes and White 1982; Forbes and White 1986). The Myakka or Immokalee fine sand soils chosen for these experiments were typical of those used in vegetable production in the Sanford area for many years. All experimental sites were tile drained and received an initial preplant application of 30 lb/acre N from a 5-5-8 (N-P2O5-K2O), 40% organic N fertilizer. Side-dressed fertilizer was applied to the base 30 lb/acre N treatment at rates of 0, 40, 80, 120, or 160 lb/acre (NH4NO3-N). Single-row beds were 5 x 26 feet. Residual soil nitrate concentrations were measured before fertilizer was side-dressed and at final harvest. Sprinkler irrigation was applied to nonmulched cucumbers in all experimental seasons.
A significant yield increase occurred with the first side-dress N application or 70 lb/acre total N (203 bushels/acre, 96% RY) in the fall 1978 season (Forbes and White 1982). Poor plant stands and reduced yield resulted from root-knot nematode damage in the spring and fall seasons of 1979. Yields were maximized each season with 150 lb/acre total N (184 and 110 bushels/acre, 100 and 97% RY). On fumigated soils the following spring (1980), highest yield occurred with 110 lb/acre N (352 bushels/acre, 100% RY). Side-dressed N increased soil NO3 concentration measured at final harvest from less than 3 parts per million (ppm)—where no N was side-dressed—to more than 20 ppm with the 120 lb/acre side-dress N treatment. Increased soil NO3 concentration late in the season resulted in fewer culled fruits and higher yields compared to plants that received no additional side-dressed N. Researchers concluded that 30 lb/acre preplant-applied N combined with a side-dress application of 80 to 120 lb/acre N was sufficient to produce optimum cucumber yield.
These experiments were continued in the spring and fall of 1981 and 1982 and in the spring of 1983 with the slicer cucumber 'Poinsett' (single-row beds, 5 x 26 feet) (Forbes and White 1986). The fall harvests were generally limited by cooler weather, shorter days, and disease problems typical of that time of year. The highest spring harvests ranged from 550 to 816 bushels/acre while highest fall harvests ranged from 240 to 287 bushels/acre. A preplant-incorporated N application of 30 lb/acre was applied as in the previous experiments. Cucumber yield generally did not increase with side-dress N application greater than 80 lb/acre. Relative yields with 80 lb/acre side-dressed N (110 lb/acre total N) were 98% (spring 1981), 94% (fall 1981), 100% (fall 1982), and 100% (spring 1983). A side-dressed N application of 150 lb/acre was required in spring 1982 to produce the maximum yield of 593 bushels/acre (100% RY) compared with 82% RY with 80 lb/acre side-dressed N. Researchers concluded that slicing and pickling cucumbers required from 80 to 120 lb/acre of side-dressed N (110 to 150 lb/acre total N) for high yield in both spring and fall seasons. Residual soil NO3 concentrations from 7 to 14 ppm were measured at final harvest sampling each season where 80 lb/acre N were side-dressed. These NO3 concentrations were sufficient to sustain late-season yields without leaving excessive N to leach through the wet summer season.
Summary of Mixed-Fertilizer Experiments
Cucumber yield responses to increased N applied in mixed fertilizers are presented in Figure 1. Plants fertilized with a 30% organic 4-8-8 (N-P2O5-K2O) formulation consistently produced greater yields compared with two inorganic 4-8-8 sources. Yield approached that of plants receiving organic mixed fertilizer when minor elements were added to an inorganic N mix of NH4NO3, (NH4)2SO4, and ammonium superphosphate. High yield occurred with 90 lb/acre N when all of the fertilizer was applied preplant in two experiments, but yield increased through 120 lb/acre N when fertilizer was split (80% preplant and 20% side-dressed). By reversing the application ratio to 20% preplant and 80% side-dressed, late-season soil NO3 concentrations were increased, the formation of cull-grade cucumbers late in the season decreased, and total yields subsequently increased. Cucumbers fertilized in double bands 2.5 inches beside and beneath the seed or 2.5 inches and 6 inches beneath the seed or broadcast and double banded resulted in higher yields in one of three experimental seasons compared with yield trials in which fertilizer was placed in a single band. Yields were higher in these early studies when cucumbers were mulched with clear polyethylene compared to those mulched with aluminum-painted polyethylene, black polyethylene, or unmulched cucumbers. Yield in most of these experiments was optimized by applying 130 to 150 lb/acre N.
Nitrogen
Responses of cucumber to N fertilizer are presented in Figure 2. Early fertilizer recommendations for cucumbers grown on irrigated mineral soils called for a base N fertilizer application of 90 lb/acre to which an additional one to four side-dress applications of 30 lb/acre could be added if leaching conditions occurred (Marlowe and Montelaro 1977; Marvel and Montelero 1967). Researchers in 1977 sought to test cucumber yield response to the recommended N rate and to excess N applied to Kanapaha fine sand soils in Gainesville (Cantliffe and Omran 1977). A base fertilization of 0, 90, or 180 lb/acre N from NH4NO3 was applied to the bed surface and tilled in preplant. Beds were spaced on 4-foot centers. As the vines began to form, 30 lb/acre N was side-dressed to all plots, raising the total base N rates to 30, 120, and 210 lb/acre. A simultaneous experiment was conducted using ethephon to chemically induce female flowering in cucumber. The effects of these treatments were observed on 'Poinsett' and 'Gemini' slicing cucumber cultivars, irrigated with 2 inches of water per week and spaced 6 inches apart. Similar yields resulted with N treatments of 30 or 120 lb/acre. Average yields produced by both N treatments were 829 bushels/acre with 'Poinsett' cucumbers and 653 bushels/acre with 'Gemini' cucumbers (Cantliffe and Omran 1977). Yields were greater in this experiment compared with previous experiments by Everett (1963), most likely due to increased plant populations compared to the earlier hill planting method, where cucumbers were planted in hills spaced 18 inches apart and thinned to one to two plants per hill.
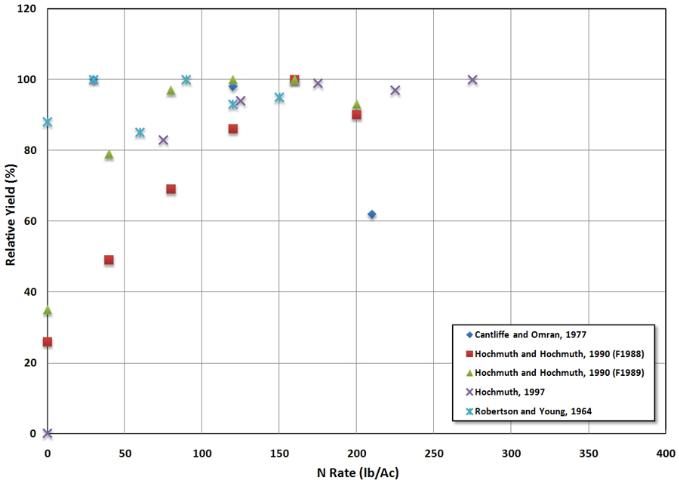
A cultivar x rate interaction was observed. 'Poinsett' cucumber yield decreased significantly to 516 bushels/acre with N at 210 lb/acre, but a lesser yield reduction resulted with 'Gemini' cucumbers (600 bushels/acre). Overall, 'Poinsett' cucumbers produced higher yield, 40% fewer cull fruit, and better fruit color than 'Gemini.' With ethephon treatments from 125 to 250 ppm, more fruits were produced, but the fruits were smaller and of lower color quality. Similar yields resulted with both the ethephon-treated and the untreated cucumbers.
Evolution of cultural practices with the use of polyethylene mulch and drip irrigation led to reevaluation of N recommendations, which were based on older, nonmulched cucumber experiments (Hochmuth and Hochmuth 1990). Nitrogen treatments of 0, 40, 80, 120, 160, and 200 lb/acre from NH4NO3 (calculated on 8,712 linear bed feet of crop per acre on 5-foot bed centers) were hand-applied and incorporated with a tiller on Klej fine sand at the Suwannee Valley Research and Education Center near Live Oak. The beds were mulched with white-on-black polyethylene in experiments conducted in the fall of 1988 and 1989. Drip irrigation was applied to maintain soil moisture tension at -12 centibars as monitored by tensiometers placed at a 12-inch soil depth. Plants were thinned to one per hill, and hills were 12 inches apart in two rows per bed.
During two September days in 1988, 4.7 inches of rain fell during an overall wet season in which 16 inches of rain likely leached nutrients through planting holes and affected cucumber more than other mulched crops (Hochmuth and Hochmuth 1990). Peak early and total yields, as determined by a linear response and plateau procedure, occurred with 107 and 136 lb/acre N, respectively, for an early yield of 226 bushels/acre and a total yield of 700 bushels/acre. The total crop value was increased by these early yields, which were largely Fancy and No. 1 grade fruit harvested when market values were high. Significant yield differences (1%) occurred with N treatments from 0 to 200 lb/acre, resulting in optimum yield with 160 lb/acre N (735 bushels/acre, 100% RY). These optimum N rates were greater than the 90 lb/acre rate recommended at that time, and this finding indicated more N was needed during a wet season. In the fall of 1989, rainfall was limited to 4.5 inches for the season. Total yield leveled off at 57 lb/acre N (694 bushels/acre) as determined by a linear response and linear plateau procedure. Significant (1%) differences occurred between yields with increased N, and optimum yield occurred with 120 lb/acre N (713 bushels/acre, 100% RY), 97% RY with 80 lb/acre N. These optimum N rates in a drier season were nearer the 90 lb/acre recommended rate compared to 69% RY in the previous wet season achieved with 80 lb/acre N. Leaf-tissue N concentrations sampled at early fruiting were between 3.5% and 4.0%, with the optimum N rates each season. Petiole sap NO3-N concentrations were approximately 500 ppm when sampled at early fruiting.
Despite the polyethylene mulch and controlled water use with drip irrigation, cucumbers in the above experiment responded to greater rates of applied N during a wet season. Given similar experiment conditions (white-on-black polyethylene mulch and drip irrigation), cucumbers grown in Gainesville were fertilized with polymer-coated Meister fertilizers (Helena Chemical Co.) in an attempt to further control the loss of N to leaching (Hochmuth 1997). Two Meister fertilizers formulated as a 15-5-15 and a 19-5-14 (N-P2O5-K2O) were applied in a 10-inch band on the bed surface at 75, 125, 175, 225, and 275 lb/acre N. Three additional treatments were compared: a zero fertilizer check treatment, 175 lb/acre NH4NO3-N, and 175 lb/acre N Meister 15-5-15. These treatments were incorporated in the bed. Fertilizer-rate calculations were based on a 6-foot-bed-center spacing, although beds were spaced 4-feet on center. 'Dasher II' seeds were planted in fall 1996 in two rows per bed with 12 inches between plants. Irrigation was applied to maintain soil moisture tension at 10 centibars as monitored by tensiometers.
Cucumber yields responded quadratically to rates of both Meister fertilizers with average yields leveling off at rates greater than 125 lb/acre N (1,340 bushels/acre, 94% RY) (Hochmuth 1997). Yields reached 99%, 97%, and 100% RY (1,427 bushels/acre) with N treatments to 275 lb/acre. These yields were high for a fall crop compared with the state average of 457 bushels/acre for fall 1996 (Fla. Dept. Agri. Consumer Serv. 1998). Yield of US No. 1 grade fruits, shorter or curved fruit, represented 10 to 15% of the total fruit yield, and cull fruits represented approximately 5%. Cull-fruit yields were unaffected by N treatment. Quadratic increases in leaf-tissue N concentration were within the sufficient range and reflected yield increases to applied N.
Where 175 lb/acre N of Meister 15-5-15 was incorporated in the bed, cucumber yields were 1,484 bushels/acre, a yield increase of 12% from yields where the same fertilizer was applied in a 10-inch band on the bed surface (1,325 bushels/acre) (Hochmuth 1997). Cucumber plants fertilized with 175 lb/acre NH4NO3-N applied broadcast and incorporated resulted in similar yields compared with plants fertilized with the incorporated Meister fertilizer. These results showed that yields with the controlled-release fertilizer leveled off after 120 lb/acre N (expressed on a 6-foot bed spacing). In this experiment, the equivalent pounds of fertilizer per acre rate (Hochmuth and Hanlon 2009) of N was 180 lbs/acre for 4-foot bed spacing.
Irrigation management is critical to successfully managing mobile nutrients such as nitrogen. Optimal management of water is important to keep the water and nutrients in the root zone. Irrigation systems vary throughout the state for cucumber production (Smajstrla and Haman 1998). Irrigation systems include subirrigation (seepage) in Southern Florida, sprinkler irrigation and drip irrigation in Miami-Dade County, and drip irrigation in Central, Southwestern, and Northern Florida. Dukes et al. (2010) provided recommendations for irrigation management for vegetable crops. Where drip irrigation is used, N and K can be applied through the drip system (fertigation). Recommendations have been provided for injection of N and K through the drip irrigation system (Hartz and Hochmuth 1996; Hochmuth and Smajstrla 1997; Hochmuth and Hartz 1996).
Summary of Nitrogen Fertilizer Trials
Where significant residual soil N concentrations occurred, maximum cucumber yield on Kanapaha fine sand soils in a spring 1977 experiment was produced with no more than 30 lb/acre N fertilizer. In most experiments, however, cucumber yields responded to N ranging from 60 to 160 lb/acre. Relative yield responses to N fertilization are presented in Figure 2. Changes in cultural practices, drip irrigation, and white-on-black polyethylene mulch resulted in higher yield of fall-grown cucumbers. Mulched and drip-irrigated beds fertilized with 120 or 160 lb/acre NH4NO3-N broadcast and incorporated produced 713 and 735 bushels/acre, respectively. These yields exceeded the state average of 460 bushels/acre (1997) for a fall cucumber crop. Further yield increases resulted when two formulations of polymer-coated fertilizer were applied in 10-inch surface bands and combined with mulch and drip irrigation. Fruit yield responded quadratically to both fertilizer formulations, leveling off at 125 lb/acre N with an average yield of 1,336 bushels/acre. Broadcast incorporation of Meister 15-5-15 (N-P2O5-K2O) polymer-coated fertilizer increased yield to 1,632 bushels/acre with 175 lb/acre N compared to 1,458 bushels/acre when the same fertilizer was applied in a 10-inch surface band in a four-foot bed spacing system. Researchers changed the 1989 recommendation of 90 lb/acre N (Kidder et al. 1989) to 150 lb/acre (Hochmuth and Hanlon 1995a) based on greater optimum yield results from mulched and drip-irrigated cucumbers fertilized with NH4NO3. Application of the recommended N rate provided some insurance against NH4NO3-N leaching through planting holes in the polyethylene during wet seasons, though high yields were possible with 125 lb/acre N when polymer-coated fertilizers were used. Plant-spacing recommendations were changed from 18 inches between hills with one and two seeds per hill to between-plant spacings of 6 to 12 inches (Hochmuth 1988a; Olson et al. 2010).
Soil Testing
Phosphorus
Adjacent sites on newly cleared land were selected for experiments near Gainesville in 1971 and 1972 (Navarro and Locascio 1979). The St. Johns fine sand soil had a pH of 3.9, 1 ppm Cu, and 5.5% organic-matter content. Soil P concentrations were not given. Application of CaCO3 elevated the pH to 5.5, and before planting, 120 lb/acre N and 150 lb/acre K2O were applied either in a band 2 inches beside the bed center and 2 inches deep or broadcast and incorporated. Phosphorus from three P sources was applied factorially at four rates with four rates of Cu added to either the band or broadcast fertilizer. 'Poinsett' cucumbers were planted at bed center and thinned to 2 feet between plants on beds spaced 4-feet apart. An additional 30 lb/acre N were applied as a side-dress.
Cucumber yield increased significantly with application of 0 to 60 lb/acre P2O5 (172 bushels/acre, 97% RY) in the 1971 experiment (Navarro and Locascio 1979). A quadratic yield response occurred that season with 0 to 230 lb/acre P2O5. Yields from plants fertilized with superphosphate (SP) were 1.6 times greater than yields from plants fertilized with either diammonium phosphate (DAP) or concentrated superphosphate (CSP). Linear yield increases resulted with increased SP, but yields decreased with rates of CSP or DAP greater than 115 lb/acre P2O5. In 1972, Cu and P interacted in their effects on total yield. Yield did not differ with P sources that season. Leaf-tissue concentrations were sufficient (0.4% P and 3.2 ppm Cu) during fruiting. Phosphorus was taken up by the plant equally when placed in a band or when broadcast, but plant uptake of Cu was more efficient with broadcast placement. Increased P application resulted in lower concentrations of Cu in plant tissue during both seasons at the 30-day sampling date. Researchers noted that excess P fertilizer resulted in Cu deficiency symptoms on soils with low Cu concentrations.
Soil P concentrations at various experimental sites on successively used vegetable-production land in Sanford at the CFREC were very high, as determined by M-1 (double-acid) extraction (Forbes and White 1982). Spring and fall cucumber crops did little to reduce soil P concentration, which was sampled before 34 lb/acre P2O5 from SP was incorporated and sampled again after the final harvest. The lowest soil P concentration was 320 ppm, and the highest was 540 ppm in the fall/spring experiment seasons from 1978 to 1980. Higher soil P concentrations extracted in spring and fall experiments from 1981 to 1983 were in the range of 500 to 633 ppm of extracted P (Forbes and White 1986). These soil P concentrations had no apparent effect on cucumber yields in any season.
Where polymer-coated (Meister) fertilizers were applied from formulations of 15-5-15 or 19-5-14 (N-P2O5-K2O) in a Gainesville 1996 fall experiment, leaf-tissue P concentrations were similar with treatments of 25 to 90 lb/acre P2O5 (Hochmuth 1997). Leaf-tissue P concentrations of 1.0% and 1.1% with P fertilizer and 0.7% with the unfertilized check treatment exceeded the sufficient range of 0.3 to 0.6% at early flower sampling.
Summary of Phosphorus Experiments
Yield increased quadratically in two Gainesville experiments to 60 lb/acre P2O5; additional P resulted in reduced tissue Cu concentrations on soils with low Cu concentrations. On these same soils, added Cu reduced yield when P was applied at low rates. Plants fertilized with SP resulted in yields 1.6 times higher than plants fertilized with either DAP or CSP. Addition of P was likely not needed on old vegetable-production soils in Sanford, Florida, where soil P concentrations ranged from 320 to 633 ppm. Current P recommendations for cucumber specify no P fertilizer application where soils test more than 31 ppm (Hochmuth and Hanlon 1995a). No research was conducted on P fertilization of cucumber on the east coast or in Southwest Florida—regions that represented 58% of the state cucumber production area. For a comprehensive review of P fertilization of cucumber, additional research is needed in these unrepresented areas.
Potassium
Research with K fertilization of cucumber plants has not been reported. References to K in research with cucumber were limited to comparisons of end-of-the-season soil K concentrations for plants receiving side-dress K applications and to leaf-tissue K concentrations as a response to increased K from mixed fertilizers or from specific K sources. This research is summarized here in lieu of yield responses to increased K fertilization.
Experiments were conducted during four seasons on different field locations at Sanford, CFREC (Forbes and White 1982). Soils were tile-drained Immokalee and Myakka fine sands, which were typically low in residual K despite long-term use for vegetable production. Soil samples were taken after 30-30-50 lb/acre (N-P2O5-K2O) was incorporated preplant and again at final harvest. At the first soil sampling, K concentrations, determined by M-1 (double-acid) extraction, were 67, 122, 50, and 68 ppm in fall 1978, spring 1979, fall 1979, and spring 1980, respectively. Where no K was side-dressed during the planting season, soil K concentrations taken at the final harvest were depleted to 35, 85, 30, and 13 ppm. These concentrations were generally low compared with the first extracted concentrations in each respective season. Very high soil K concentrations, greater than 125 ppm, occurred where 200 lb/acre K2O was side-dressed in fall 1978 and spring 1979. Where side-dress applications of 50 to 200 lb/acre K2O were applied, soil K concentrations at final harvest were generally in the medium to high range in all seasons except spring 1980. In this season, larger harvests (326 to 349 bushels/acre) likely depleted final harvest soil samples to low K concentrations. Residual soil K concentrations at harvest were higher where 150 lb/acre K2O was side-dressed from KCl instead of K2SO4.
Experiments continued at the Sanford CFREC, conducted as before on Immokalee or Myakka fine sand soils and fertilized with 30-30-50 lb/acre (N-P2O5-K2O) incorporated preplant (Forbes and White 1986). Soil K concentrations were determined by M-1 soil-test extractant from samples taken after preplant fertilization and at final harvest. Spring soil test results for 1981, 1982, and 1983 seasons averaged 83 ppm K with the first sampling and 52 ppm K—a medium soil K concentration—with the final harvest sampling from sites where K was not side-dressed. With side-dressed K applications through 200 lb/acre K2O, soil K concentrations increased to 108 ppm—a high soil K concentration. In 1983, residual soil K concentrations sampled after 32 inches of rainfall from April through September were depleted to medium (38 to 45 ppm) concentrations from an average high range of spring harvest concentrations of 52 to 108 ppm. Average soil K concentrations for fall seasons 1981 and 1982 were 93 ppm after preplant fertilization, 62 ppm after harvest where no K was side-dressed, and 116 ppm where K was side-dressed with 200 lb/acre K2O. Soil K concentrations did not differ after a side-dress application of 150 lb/acre K2O from KCl or K2SO4.
Cucumber plants mulched with white-on-black polyethylene and drip irrigation were evaluated for yield response to fertilizer rates and placement methods in a fall 1996 Gainesville experiment (Hochmuth 1997). Leaf-tissue K concentration was compared for plants that received no K fertilizer, plants fertilized with a blended polymer-coated 15-5-15 or 19-5-14 (N-P2O5-K2O) fertilizer applied to the bed surface in a 10-inch band or broadcast and incorporated, and plants fertilized with soluble KNO3 applied at 175 lb/acre K2O. Leaf-tissue K concentration sampled at early flowering and harvest did not differ with increased K from the polymer-coated fertilizers applied from 75 to 275 lb/acre K2O, with either the band or incorporated placement methods, or from the soluble K fertilizer source incorporated in the bed at 175 lb/acre K2O. Average leaf-tissue K concentrations of 4.3% at early-flower sampling and 3.0% at late harvest sampling were high (sufficient concentrations for these stages were 1.6 to 3.0%).
Summary of Potassium Trials
Side-dress K applications from 50 to 150 lb/acre K2O were shown to hold soil K at medium to high concentrations through harvest, compared with low concentrations where no K was side-dressed in fall and spring experiments from 1978 to 1983. In continued fall and spring experiments from 1981 to 1983, end-of-season K concentrations were medium where no K was side-dressed and high where 50 to 200 lb/acre K2O was side-dressed. Residual soil K concentrations were higher with KCl fertilizer compared with K2SO4 fertilizer in some experiments but not in others. Mulched and drip-irrigated cucumber plants had similar and above-sufficient leaf-tissue K concentrations when fertilized with either of two polymer-coated Meister fertilizers applied from 75 to 275 lb/acre K2O and placed in a band or incorporated, or from incorporated KNO3 fertilizer applied at 175 lb/acre K2O.
Overall Summary
A substantial amount of research with N fertilization of cucumber has been conducted in Florida, and in most cases, the current recommendation of 150 lb/acre N was supported for cucumbers grown in double-row patterns on beds with centers spaced 6 feet apart. No research has been reported that addresses management of N and irrigation in relation to nutrient leaching. More research is needed, and this research should also study P and K fertilization of cucumber.
Some general conclusions from the research:
- Cucumber has been studied intensively for N requirements.
- The majority of the results show that maximum yield was obtained at 150 to 175 lbs/acre N. In several studies, less N was required for maximum yields.
- In two studies, yield was reduced with 200 lbs N/acre or more.
- Not enough research has been conducted with P or K to draw conclusions about crop nutrient requirements for those two nutrients.
Literature Cited
Black, C. A. 1992. Soil fertility evaluation and control. Lewis Publishers, Boca Raton, FL. 746 pp.
Brown, J. R. 1987. Soil testing: sampling, correlation, calibration, and interpretation. Soil Science Society of Amer. Special Publ. No. 21. Soil Science Society of America, Inc. Madison, Wisc.
Bryan, H. H. 1966. Effect of plastic mulch on the yield of several vegetable crops in north Florida. Proc. Fla. State Hort. Soc. 79:139-146.
Cantliffe, D. J., and A. F. Omran. 1977. Nitrogen fertilization rates for slicing cucumbers treated with ethephon. Proc. Fla. State Hort. Soc. 90:373-376.
Dukes, M. D., L. Zotarelli, and K. T. Morgan. 2010. Use of irrigation technologies for vegetable crops in Florida. HortTechnology 20:133-142.
Everett, P. H. 1963. Minor element and nitrogen studies with cucumbers. Proc. Fla. State Hort. Soc. 76:143-149.
Everett, P. H. 1978. Fertilizing tomatoes or cucumbers as second crops on plastic mulched beds. Proc. Fla. State Hort. Soc. 91: 317-319.
Florida Dept. of Agriculture and Consumer Services. 1998. Florida Agricultural Statistics, Vegetable Summary 1996-1997. 70 pp. Fla. Agric. Stat. Serv., Orlando, FL.
Florida Agriculture Statistical Bulletin. 2009. Season vegetables highlights, https://www.nass.usda.gov/Statistics_by_State/Florida/Publications/Annual_Statistical_Bulletin/2009/E1thru20Veg-2009.pdf [Accessed October 2022].
Florida Dept. of Agriculture and Consumer Services. 2005. Water quality/quantity best management practices for Florida vegetable and agronomic crops. http://www.floridaagwaterpolicy.com/PDF/Bmps/Bmp_VeggieAgroCrops2005.pdf.
Forbes, R. B., and J. M. White. 1982. Nitrogen and potassium side-dressing of pickling cucumbers. Soil Crop Sci. Soc. Fla. Proc. 41:182-185.
Forbes, R. B., and J. M. White. 1986. Fertilization of slicer cucumbers in central Florida. Soil Crop Sci. Soc. Fla. Proc. 45:87-90.
Hanlon, E. A., and G. J. Hochmuth. 1989. Calculating fertilizer rates for vegetable crops grown in raised-bed cultural systems in Florida. Fla. Coop. Ext. Serv. Spec. Series SS-SOS-901.
Hartz, T. K., and G. J. Hochmuth. 1996. Fertility management of drip-irrigated vegetables. HortTechnology 6:168-172.
Hochmuth, G. J. 1988a. Cucumber production guide for Florida. Fla. Coop. Ext. Serv. Circ. 101E.
Hochmuth, G. J. 1988b. Commercial Vegetable Fertilization guide. Fla. Coop. Ext. Serv. CIR 225C.
Hochmuth, G. J. 1992a. Fertilizer management for drip-irrigated vegetables in Florida. HortTechnology 2:27-32.
Hochmuth, G. 1992b. Concepts and practices for improving nitrogen management for vegetables. HortTechnology. 2 (1): 121-125.
Hochmuth, G. J. 1994a. Plant petiole sap-testing guide for vegetable crops. Fla. Coop. Ext. Serv. Circ. 1144.
Hochmuth, G. J. 1994b. Sufficiency ranges for nitrate-nitrogen and potassium for vegetable petiole sap quick tests. HortTechnology 4:218-222.
Hochmuth, G. J. 1995. Commercial vegetable fertilization guide. Fla. Coop. Ext. Serv. Circ. 225D.
Hochmuth, G. J. 1996a. Vegetable fertilization pp. 3-17. IN: G. Hochmuth and D. Maynard (eds.) Vegetable production guide for Florida. Fla. Coop. Ext. Serv. Circ. SP 170.
Hochmuth, G. J. 1996b. Commercial Vegetable Fertilization guide. Fla. Coop. Ext. Serv. CIR 225D.
Hochmuth, G. J. 1997. Response of cucumber to Meister controlled-release fertilizers. Fla. Agr. Expt. Sta. Research Report. Suwannee Valley REC 97-01.
Hochmuth, G. J. 2000. Management of nutrients in vegetable production systems in Florida. Soil and Crop Sci. Soc. Fla. Proc. 59:11-13.
Hochmuth, G. J., and K. Cordasco. 1999 (reviewed in 2009). A summary of N, P, and K Research with cucumber in Florida. Fla. Coop. Ext. Serv. HS 749.
Hochmuth, G. J., and E. A. Hanlon. 1989. Commercial vegetable crop nutrient requirements. Fla. Coop. Ext. Serv. Circular 806.
Hochmuth, G. J., and E. A. Hanlon. 1995a. IFAS standardized fertilization recommendations for vegetable crops. Fla. Coop. Ext. Serv. Circ. 1152.
Hochmuth, G. J., and E. A. Hanlon. 1995b. Commercial vegetable crop nutrient requirements in Florida. Fla. Coop. Ext. Serv. SP 177.
Hochmuth, G. J., and E. A. Hanlon. 2000. IFAS standardized fertilization recommendations for vegetable crops. Fla. Coop. Ext. Serv. Circ. 1152.
Hochmuth, G. J., and E. A. Hanlon. 2009. Calculating recommended fertilizer rates for vegetable crops grown in raised-bed mulched cultural systems. Fla. Coop. Extension Serv. Circ. SL 303. https://edis.ifas.ufl.edu/pdffiles/ss/ss51600.pdf.
Hochmuth, G. J., and E. A. Hanlon. 2010a. Commercial vegetable fertilization principles. Fla. Coop. Extension Serv. Cir. SL 319. https://edis.ifas.ufl.edu/pdffiles/cv/cv00900.pdf.
Hochmuth, G. J., and E. A. Hanlon. 2010b. Principles of sound fertilizer recommendations. Fla. Coop. Extension Serv. Cir. SL 315.
Hochmuth, G. J., and T. K. Hartz. 1996. Fertility management of drip-irrigated vegetables. HortTechnology 6:168-172.
Hochmuth, R. C., and G. J. Hochmuth. 1990. Nitrogen requirement for mulched slicing cucumbers. Soil Crop Sci. Soc. Fla. Proc. 50:130-133.
Hochmuth, G. J., and D. N. Maynard. 1996. Vegetable production guide for Florida. Fla. Coop. Ext. Serv. Circ. SP 170.
Hochmuth, G. J., D. N. Maynard, C. Vavrina, E. A. Hanlon, and E. H. Simonne. 2009. Plant Tissue Analysis and Interpretation for Vegetable Crops in Florida. 55 pg. https://edis.ifas.ufl.edu/publication/ep081.
Hochmuth, G., D. N. Maynard, C. Vavrina, and E. A. Hanlon. 1991b. Plant-tissue analysis and interpretation for vegetable crops in Florida. Fla. Coop. Ext. Serv. Spec. Ser. SS-VEC-42.
Hochmuth, G. J., and A. G. Smajstrla. 1997. Fertilizer application and management for micro (drip)-irrigated vegetables in Florida. Fla. Coop. Ext. Serv. Circ. 1181.
Kidder, G., E. A. Hanlon, and G. J. Hochmuth. 1989. IFAS standardized fertilization recommendations for vegetable crops. Fla. Coop. Ext. Serv. Spec. Ser. SS-SOS-907.
Montelaro, J. 1978. Commercial vegetable fertilization guide. Fla. Coop. Ext. Serv. Circ. 225-B.
Mylavarapu, R. S. 2009. UF/IFAS Extension Soil Testing Laboratory (ESTL) Analytical Procedures and Training Manual. Circular 1248, 19 pg. https://doi.org/10.32473/edis-ss312-2009.
Kidder, G., E. A. Hanlon, and G. J. Hochmuth. 1989. IFAS standardized fertilization recommendations for vegetable crops. Fla. Coop. Ext. Serv. Spec. Ser. SS-SOS-907.
Kostewicz, S. R., and D. J. Cantliffe. 1975. Growing pickling cucumbers in Florida. Extension Vegetable Crops Mimeo report 75-3. Vegetable Crops Dept. University of Florida, Gainesville, Fla.
Marlowe, G. A., and J. Montelaro. 1977. Cucumber production guide. Fla. Coop. Ext Serv. Cir 101D.
Marvel, M., and J. Montelaro. 1967. Cucumber Production guide. Fla. Coop. Ext Serv. Cir 101D.
Navarro, A. A., and S. J. Locascio. 1979. Copper nutrition of cucumber (Curcumis sativus L.) as influenced by fertilizer placement, phosphorus rate, and phosphorus source. Soil Crop Sci. Soc. Fla. Proc. 39:16-19.
Nettles, V. F., and W. C. Hulburt. 1966. Effect of placements and levels of fertilizer on the yield of vegetables. Proc. Fla. State Hort. Soc. 101:185-191.
Olson, S. M., and B. Santos. 2010. Vegetable production handbook of Florida. Univ. of Fla. IFAS Extension. https://ufdcimages.uflib.ufl.edu/UF/00/09/91/59/00001/Binder1.pdf [Accessed October 19, 2022].
Olson, S. M., E. H. Simonne, W. M. Stall, P. D. Roberts, S. E. Webb, and S. A. Smith. 2010. Cucurbit production in Florida. Fla. Coop. Ext. Serv. Cir HS 725. https://edis.ifas.ufl.edu/pdffiles/cv/cv12300.pdf.
Robertson, W. K., and H. W. Young. 1964. Response of vegetables grown in the Quincy area on Norfolk loamy fine sand to fertilizer and soil amendments. Proc. Fla. State Hort. Soc. 77:198-204.
Schepers, J. S., K. D. Frank, and C. Bourg. 1986. Effect of yield goal and residual nitrogen considerations on nitrogen fertilizer recommendations for irrigated maize in Nebraska. J. Fertilizer Issues 3:133-139.
Simonne, E. H., M. D. Dukes, and L. Zotarelli. 2010. Principles and practices for irrigation management for vegetables. Fla. Coop. Ext. Serv. AE 260. https://edis.ifas.ufl.edu/pdffiles/cv/cv10700.pdf.
Simonne, E. H., and G. J. Hochmuth. 2010. Chapter 2. Soil and Fertilizer Management for Vegetable Production in Florida. Vegetable Production Handbook. HS711. 3-15. https://edis.ifas.ufl.edu/publication/cv101.
Smajstrla, A. G., and D. Haman. 1998. Irrigated acreage in Florida: A summary through 1998. Fla. Coop. Ext Serv. Cir 1220. https://edis.ifas.ufl.edu/ae150.