Purpose
This manual has been designed as a reference source for UF/IFAS Extension office laboratories offering soil pH and/or electrical conductivity tests to their clients. This manual, if followed, will assist county faculty in assuring that these laboratory measurements are done correctly with high-quality assurance.
Soil pH and Its Uses
Soil pH measurement is useful because it is a predictor of various chemical activities within the soil. As such, it is also a useful tool in making management decisions concerning the type of plants suitable for location, the possible need to modify soil pH (either up or down), and a rough indicator of the plant availability of nutrients in the soil.
Aluminum
Aluminum in the soil can adversely affect plants if aluminum occurs in certain forms and its activity is elevated sufficiently. As the activity of aluminum increases, the soil becomes more acidic and soil pH decreases. If the pH is low enough and aluminum is present in sufficient quantity, plants may be stunted or lost due to aluminum toxicity. In most Florida sandy soils, there is danger of aluminum toxicity when soil pH is below 5.0.
The occurrence of aluminum toxicity (at acidic pHs) decreases somewhat as one travels from the Florida Panhandle and down the peninsula. The reason for this apparent change is found in changes in the soil constituents. Aluminum levels tend to decrease, and the aluminum is found in different minerals in the soil as one travels south in Florida.
Organic soils have low total aluminum. Low soil pH in these soils does not pose a threat from aluminum toxicity in Florida.
Nitrogen-Fixing Microbes
Legume plants have a helpful relationship with selected soil microbes. These microbes convert nitrogen gas from the atmosphere to forms useful to the plant for growth and improved yield. In turn, the microbes are supplied nutrients and carbohydrates from the plant. This mutual, beneficial existence is termed symbiosis. Agronomic examples of this symbiotic relationship are alfalfa, peanut, and soybean.
Soil pH directly affects the activity of these microbes. Research conducted in the absence of aluminum toxicity has shown that once the soil pH has decreased to 4.7 or lower, the ability of the microbes to convert nitrogen is greatly reduced. If aluminum is present, then both the microbial symbiotic activity and the normal metabolism of the plant are adversely affected.
Solubility of Plant Nutrients
Soil pH directly affects the solubility of many of the nutrients in the soil needed for proper plant growth and development. These chemical reactions are complex and have often been generalized with charts that oversimplify chemical conditions in specific soils. One should be careful in using these pH nutrient charts when dealing with soils in Florida, because they may be misleading.
As soil pH decreases, nutrients, such as phosphorus, usually decrease in plant availability because of precipitate reactions with iron and aluminum. However, plants can affect their micro-environment and are often found to grow well over a range of soil pH. This range of successful growth is often as great as 1 to 2 pH units. In general, many plants will do well in a soil pH range of 5.5 to 7.5. Specific plants, such as azalea or pine seedlings, actually require low soil pH. Such plants are often iron inefficient, meaning that they require low soil pH to aid in the uptake of iron from the soil.
As soil pH increases above 6.5, manganese, a micronutrient, may become limiting to plant growth. Phosphorus and micronutrients such as copper and zinc also decrease in their plant availability at high pH. Soils composed of limestone (such as those in the Dade County area) have a high native soil pH of about 8.3. Plants grow in these high pH soils, but nutrient deficiencies are common.
Optimum pH Ranges for Plants
As stated above, most plants do well over a range of soil pH values. This point bears repeating because the best management of soil pH is often to do nothing. Because many plants directly modify the chemical environment around their roots, nutrient limitations are not found, plant production is not adversely affected, and visual stress symptoms are not observed.
The nutrient recommendations from the UF/IFAS Extension Soil Testing Laboratory (ESTL) are based on the IFAS Standardized Fertilization Recommendation process (Mylavarapu et al. 2000). Nutrient recommendations for agronomic and horticultural crops in Florida are detailed in EDIS documents SL129 (https://edis.ifas.ufl.edu/ss440) and CIR1152 (https://edis.ifas.ufl.edu/cv002) (Mylavarapu et al. 2015; Mylavarapu et al. 2017).
Specific pH values are cited in this system as target pHs. A target pH is a soil pH, within the optimum pH range, that is used for the calculation of lime rates. A target pH is usually selected such that the adverse effects of aluminum toxicity are avoided and so that nutrient availability for that crop will be adequate. A target pH is not the only pH at which the crop will do well.
Liming of Soils
If the soil pH is greater than 0.2 units below the target pH, the ESTL will complete an additional test, the Adams-Evans Buffer. This test has been specifically designed for the sandy soils found in the southern United States. Furthermore, current lime recommendations have been calibrated for Florida conditions using both the soil pH and the Adams- Evans Buffer.
Soil pH is a measure of the active acidity, that portion of the hydrogen ions that is active in the soil solution. Soil pH does not measure the reserve acidity. Reserve acidity is that portion of hydrogen and other acid-contributing ions that are sorbed on soil particles. Usually, the reserve acidity is much greater than the active acidity. The Adams-Evans test is designed to measure this reserve acidity. Together, the target pH, the soil pH, and the Adams-Evans test can be used to determine the amount of lime required to adjust the soil pH from its current reading to the target pH.
Unfortunately, the Adams-Evans test is rather complex and contains chemicals that must be handled and disposed of as hazardous waste. For this reason, UF/IFAS Extension office laboratories should not offer the Adams-Evans test. However, local knowledge of the county and its soils can help considerably with local lime recommendations. It is recommended that liming recommendations made at the county laboratory be verified by submitting a small percentage (e.g., 5%) of soil samples to the ESTL. Comparison of the local recommendations with those from the ESTL will allow UF/IFAS Extension faculty to calibrate their recommendations.
Acidifying Soil
In some situations, it may be desirable to acidify the soil, that is, to lower soil pH. If the high soil pH is a natural condition, there is little that can be done to lower soil pH permanently. Treatment with sulfur will lower the pH for a few weeks, but the pH will eventually increase. In landscaping, it is often better to select plants that are adapted to the natural soil pH range rather than to use plants that will need constant soil pH maintenance and usually look unhealthy even after this extra effort.
In situations where the high soil pH condition was created by human activity, for instance overliming, it is often feasible to lower the soil pH with one or two applications of sulfur. In such cases, the amount of overliming is relatively small, say 1 ton/acre (46 lb/1000 sq ft) and can be treated successfully. In naturally occurring high pH soils, the effective lime equivalent will usually be over 100 tons/acre.
Unfortunately, there is no soil test available to assist in determining the amounts of sulfur (S) needed to reduce soil pH. If plants are actively growing, agricultural sulfur treatment should be restricted to a maximum of 300 lb S/acre (7 lb S/1000 sq ft). Damage may still occur at this rate if the S is allowed to remain in contact with the foliage.
Multiple treatments during the growing season should be done with caution—that is, allowing enough time (usually about 1 month) for the previous S treatment to react with the soil. Sulfur added to the soil must undergo oxidation by soil microbes, resulting in the production of hydrogen ions and sulfate. Because microbial action controls the effectiveness of the treatment, warm moist soil conditions are preferable to dry or cool conditions. Treatment of the soil with gypsum (calcium sulfate) will not change soil pH because gypsum does not contribute any acidity to the soil. It is a pH-neutral salt. Reasons for increases in soil and options for lowering soil pH are detailed in SL437 (https://edis.ifas.ufl.edu/ss651) (Mylavarapu et al. 2019).
Combination pH Electrodes
The pH electrodes shown in Figures 1 and 2 are examples of combination pH electrodes, which contain a fibrous or ceramic chip that allows the internal solution to slowly flow out from the body of the electrode into the external solution of the sample being measured.
This leaking results in a completed electrical circuit. Inspection should include checking that the chip is in place and is relatively clean of debris from past use. Often the chip will be white or light gray in color, indicating that it is still functioning. If the chip is a different (darker) color, the electrode may not function properly. Use of a small amount of abrasion may restore the chip, but too much abrasion may damage the electrode.
Lastly, the barrel of the electrode often contains a filler port near the top of the electrode. Most manufacturers recommend that the covering be removed from this port so that pressures do not develop within the operating electrode.
The solution, often a saturated solution of potassium chloride (see specifications for your specific electrode), should be kept within 1 inch of the filler port. This level is enough to ensure that the internal solution level is higher than the sample solution being analyzed. This difference permits flow out from the electrode through the chip.
A relatively new type of electrode is constructed with an internal fluid that is a gel. This type of electrode does not contain a filler port, because it cannot be serviced by the user.
These combination pH electrodes should be replaced if broken or if they generate erroneous readings not resolved by recalibration or replacement of potassium chloride solution in probes.
Contact the manufacturer if the electrode or the ball has dried out. Rejuvenation often calls for strong acids, which present a personal safety hazard. County faculty may wish to replace electrodes rather than attempting rejuvenation.
Inspection of the electrode wire should not reveal any loose connections or breaks in the insulation. There are no serviceable parts on the pH electrode.
pH Meter
The pH meter is a sensitive electronic device, often designed with printed circuits making it quite reliable. However, improper handling (bumps, hot dashboard storage, or solution spills) can damage the meter. The meter is designed to measure changes of millivolts between the reference and pH electrodes. To understand the sensitivity needed to measure millivolts, human neck pains are often induced by 7 to 20 millivolts.
Most problems associated with reading pH usually originate with faulty electrodes. Additionally, if the meter contains batteries, they should be checked before each use. Low or inadequate power supply will result in inaccurate pH readings.
In any case, unless the instrument completely fails, it will always give a pH reading whether it is functioning correctly or not. It is the responsibility of the operator to detect problems and to report accurate pH results.
Electrical Conductivity and Its Uses
The Electrical Conductivity (EC) of a solution is a measure of the ability of the solution to conduct electricity. The EC is reported in either meter. When ions (salts) are present, the EC of the solution increases. If no salts are present, then the EC is low, indicating that the solution does not conduct electricity well.
The EC indicates the presence or absence of salts but does not indicate which salts might be present. For example, the EC of a soil sample might be considered relatively high. No indication from the EC test is available to determine if this condition was from irrigation with salty water or if the field had been recently fertilized and the elevated EC is from the soluble fertilizer salts. To determine the source of the salts in a sample, further chemical tests must be performed.
Soluble Salts vs. EC
Prior to 1989, the ESTL reported soluble salts values. "Soluble salts" is an older term and is derived from EC measurements. Unfortunately, there are a number of assumptions that must be made before soluble salts can be calculated. Conversion factors (EC to soluble salts) were different across the United States, ranging from 600 to 700. Florida used a conversion factor of 700.
These assumptions, and their introduced errors, are avoided by reporting the actual EC measurement. The EC can also be directly used in the body of literature, which is continuously growing, concerning plant productivity under the effects of salinity. The older term soluble salts should be avoided by UF/IFAS Extension laboratories.
Interpretation of Electrical Conductivity of Irrigation Water
Frequent use of irrigation water will directly influence the salts in the soil profile. Salts are influenced by factors such as rainfall content and timing, internal soil drainage, and irrigation practices. Usually, rainfall contains low amounts of salts and acts to dilute salts that are present in the soil. If the rainfall is of sufficient volume or duration, and the soil has internal drainage, the added rainfall is enough to leach salts from the soil. During drying conditions, water is lost from the soil due to evaporation, and salts are effectively concentrated.
If irrigation water contains appreciable salts, then intensive management is required to produce healthy plants. Therefore, EC measurement of the irrigation water source is an excellent management decision. Table 1 has been developed to assist in making a decision to use a water source.
Interpretation of Electrical Conductivity of Soils
In actuality, the interpretation of EC of a soil or media must be made considering the plant(s) to be grown. The EC of the soil has little direct detrimental effect on sandy mineral soils or on media. However, EC directly affects plants growing in the soil or media. The impact of EC on plants is also directly affected by water management.
Salt Index Use and Calculation
As EC increases, more attention to water management is needed to prevent salinity from adversely affecting plants. The UF/IFAS Extension Soil Testing Laboratory uses a 2:1 solution:soil ratio with which to determine EC. Many states use a saturated paste extract. This saturated paste method is more time consuming than the 2:1 extraction, and results in inadequate amounts of solution in Florida's sandy soils. The conversion from the 2:1 extraction result to the saturated paste result, termed salt index, is easy and accurate.
EC (salt index) = EC (2:1) × 8
In general, when the soil EC (2:1, water:soil) exceeds 0.25 dS/m (or 0.25 × 8 = 2.0 dS/m salt index), many plants experience stress due to salts. Other plants (e.g., bermudagrass) are quite tolerant to salts. Due to this species-dependent effect, reference data for EC of soil related to specific plant species is published in UF/IFAS Circular 1092, Soil and Container Media Electrical Conductivity Interpretations (https://edis.ifas.ufl.edu/ss117) (Hanlon et al. 2017).
EC Probe and Meter
There are a wide variety of EC probes and meters marketed. Because EC is a measurement of the conductivity of the soil solution, the measurement should be made under a controlled mixture of solution to soil (2:1). These conditions usually do not exist when direct reading instruments are used.
Electrical Conductivity Probe
The probe consists of a tube, usually plastic, into which electrodes have been installed. Two common electrode arrangements are: 1) two plates; or 2) a rod located con- centrically in a ring. In either case, the electrodes are held a specific distance apart. The gap between the electrodes is filled with the water sample or filtered solution from a soil sample, either by filling a reservoir or by placing the probe in the solution. The ESTL currently uses a 4-electrode graphite cell sealed in epoxy for its EC measurements (Figure 3). This type of probe is virtually indestructible and can be used without filtering the soil solution.
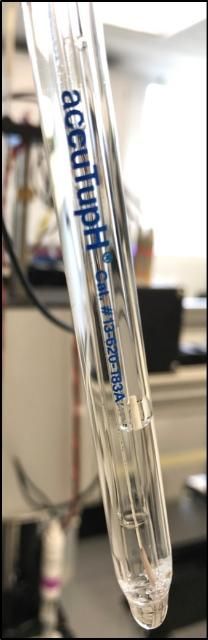
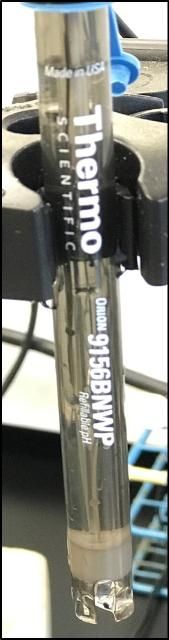
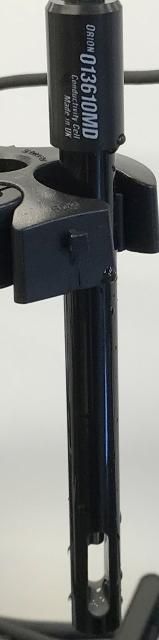
When the probe is immersed in the solution, ions contained in the solution will permit electrical flow from one electrode to another. If a large number of ions are present (salty conditions), then the EC of the sample will be higher than a sample with a low number of ions (low salts).
Inspection of the probe should include ensuring that the probe is clean and free of debris, and that all electrical connections are in place. Some probes may experience a buildup of corrosion on the electrodes with time. Indication of corrosion, if not directly visible, is indicated by the constant need to reset the instrument because of drifting, often in one direction as corrosion progresses.
Electrical Conductivity Meter
Meters for EC are extremely reliable. Meters from the 1950s that use a "cat's eye" tube are still in use. Newer meters using digital displays are often susceptible to failure of one or more segments of the digital display, usually related to corrosion within the instrument.
As with failures associated with pH meters, EC meters will always provide an EC, unless the problem results in total instrument failure. It falls upon the operator to ensure that the reported EC is an accurate measurement of the conductivity of the sample.
Laboratory Procedures
The following procedures are used at the ESTL and are based on good laboratory procedures with sufficient quality control measures to ensure that pH and EC readings are accurate and reliable. While equipment may vary among UF/IFAS Extension Laboratories, procedures should be developed that directly parallel those used by the ESTL.
I. Soil Scooping Technique
The ESTL uses a scoop (that is, a volume/measurement) for both pH and EC determinations. The scoop is a plastic and metal device that may be obtained from a commercial manufacturer. Alternately, the scoop may be constructed from locally available materials, such as measuring spoons or coffee scoops. The intent is to use a consistent volume of soil and water.
The soil scooping technique requires practice, despite its unsophisticated appearance. The technique depends upon uniform actions by the technician from sample to sample to produce consistent packing of soil into the scoop. This consistency can be directly measured by repeatedly scooping the same soil and weighing each scoop. Weights should be uniform within each scoop (volume) and soil-sample combination. Weights will vary from soil to soil, especially when there is a noticeable difference in soil texture.
A. Sample Handling and Preparation
The sample should be air-dried and passed through a 2 mm sieve before scooping.
B. Procedure
-
Dip the scoop into the center of the soil sample and fill the scoop with a twisting motion so that extra soil is mounded above the rim of the scoop. Do not press the scoop or force the soil against the side of the container (Mylavarapu and Miller 2014).
-
Strike the handle near the scoop three times with a plastic rod to settle soil particles.
-
Level the scoop with the plastic rod. Strike off all excess soil above the rim of the scoop in a single stroke so that the soil is not compacted into the scoop.
II. Estimation of Soil Texture
Knowledge of soil texture is useful in the recommendation of lime. Local soil conditions may be quite uniform so that little differences may be found throughout the county. However, such uniformity is expected to be rare in most counties. After the soil has been air-dried and sieved, a small amount of dry soil should be moistened and rubbed between the forefinger and thumb. An estimation of the texture is made by comparing the "feel" of the sample to that of a set of soil samples of known texture (Table 2).
III. Soil pH (2:1 V/V)
This procedure uses a 20 cc (~25 g) soil scoop and 40 mL of pure water to obtain a 2:1 water-to-soil ratio. Most problems with this procedure are associated with older glass or calomel electrodes. However, sample pH may also be affected by contaminated water, by microbial activity if samples are allowed to sit for several hours before determining pH, or by improper scooping techniques.
A. Standard Solutions
Obtain commercial standard solutions of pH 4.00, 7.00, and 10.00.
B. Sample Handling and Preparation
The soil sample should be air-dried and passed through a 2 mm sieve. Irrigation water samples require no preparation.
C. Procedure
-
One scoop (see Soil Scooping Technique) of soil to a 3 oz plastic cup using a 20 cc (~25 g) scoop.
-
Add 40 mL of pure water to each cup using an automatic pipette or suitable volumetric container. Stir with a glass rod and let the sample stand for 30 minutes.
-
Standardize the pH meter (see following section, pH Meter).
-
Stir the sample again immediately before measuring the soil pH. Do not place the electrode(s) directly in the sand layer at the bottom of the container. The electrodes should be positioned in the solution just above the sand layer.
-
Record pH to the nearest 0.1 pH unit (suggested format of XX.X).
IV. Electrical Conductivity
This test, often called "Soluble Salts," requires that 20 cc (~25 g) of soil be mixed with 40 mL of pure water, resulting in a water:soil ratio of 2:1. The 4-hour equilibration period provides time for some slowly soluble constituents to approach solution equilibrium. Little error results from much longer equilibration times, but shorter time periods might introduce inconsistent results for some samples.
A. Standards
A 0.01 M solution of potassium chloride, prepared by dissolving 0.7456 g KCl in 1 L of deionized water has an EC of 0.1413 dS/m at 25°C.
Potassium chloride (KCl), 0.1 M: Dissolve 7.456 g KCl in 1 L of deionized water. The EC for this solution is 12.9 dS/m at 25°C.
Optionally, use a commercially prepared solution as the reference solution.
B. Sample Handling and Preparation
The soil sample should be air-dried and passed through a 2 mm sieve. Irrigation water samples require no preparation.
C. Procedure
-
Place 20 cc (~25 g) of soil in a plastic 3 oz cup.
-
Add 40 mL of pure water, stir, and allow to stand for 4 hours.
-
Standardize the conductivity meter (see the following section on the Conductivity Meter) just before reading EC in samples.
-
Insert probe into water above the soil to obtain EC measurement.
-
Move the probe up and down in the solution several times to dislodge any bubbles on the electrode surfaces. Measure the electrical conductivity of the extract contained in the funnel tube.
-
Rinse the interior and exterior of the probe with pure water between samples. Remove any excess water from the exterior of the probe by blotting with a tissue.
-
Record all meter readings as displayed. Note that some meters use a floating-point display while others use a reading that depends upon a switch setting.
-
If an older probe is used for this measurement, take the time to filter the solution as not to damage the probe.
-
If required, filter the solution through a Whatman No. 41 (11 cm) paper and collect the extract in a funnel tube or other suitable container. The intent is to remove the soil and other debris from the solution so as not to damage the probe.
V. pH Meter
Instructions for the proper use of a pH meter, including electrode care and instrument-specific settings, are given in this subsection. While instrument settings may vary among meters, daily operation of pH meters is relatively standard.
Most problems with pH instruments originate within the electrode(s) or in the electrical connections to the instrument. Prevention of early electrode deterioration is best accomplished by following the information in sections B and C that follow.
Use of only one standard buffer solution is not adequate for proper calibration. Do not reuse a buffer solution that has been left out overnight or has been used to "store" electrode(s). It is advisable to maintain a supply of a reference soil sample (see Appendix for more information) to be used as a check sample with every set of samples. Early detection of problems is the best method of avoiding inaccurate results.
A. Calibration of Electrode(s)
-
Rinse electrode(s) with pure water.
-
Using commercially prepared buffer solutions of pH 4.00, 7.00, and 10.0, pour approximately 30 mL into labeled, 3 oz plastic cups. Do not reuse buffer solutions in which electrode(s) have been immersed for daily storage.
-
Calibrate the pH meter according to manufacturer specifications.
-
Check calibration against buffer solutions that bracket the range of use for the pH meter. These standards should be within 0.05 pH units of their true value.
-
Using a reference soil sample (see Appendix), read the pH and determine if the pH reading agrees with the "known" reading of the reference soil. Use of a reference soil sample, a soil that can be analyzed with every sample set, is strongly recommended. Use of a reference soil is an excellent quality-assurance measure and verifies that the electrodes and meter are functioning correctly in a soil solution and not just in standard buffers. The reference soil sample should be read once for each 20 samples analyzed. If the reading drifts by more than ±0.2, then restandardize the meter.
-
Standardize the instrument according to the above procedure. If the instrument has shown drift of more than 0.1 pH units, reread the last few samples to verify the accuracy of the recorded readings.
B. Daily Electrode Storage
-
Refer to manufacturer's instructions regarding proper storage of the pH probes.
-
Most manufacturers provide an electrode storage solution for long-term storage of pH probes.
-
A 4.0 buffer solution can be used for short-term storage of pH probes.
C. Electrode Servicing
-
If the pH reading drifts by more than ±0.1 pH units, the meter should be recalibrated. Usually, new electrodes should be kept in pH 4.00 buffer solution, or an electrode storage solution before use.
-
After use, wash the electrodes with pure water.
-
Immerse the electrodes in pH 4.00 buffer solution for short-term storage or an electrode storage solution for long-term storage.
VI. Electrical Conductivity Meter
Conductivity measurements must be made on soil solution samples only and not used directly in soil when taking measurements.
The most common problem with older-style conductivity meters concerns failure of the electrodes (mounted within the hollow plastic probe) to make proper contact with the solution. These probes must be kept clean by adequately flushing the interior of the probe with pure water. Newer-style probes are designed to negate these concerns and are typically sealed within epoxy resin to prevent damage. When analyzing a solution, ensure that the solution covers the bottom 3 to 5 cm (1 to 1.5 inches) of the probe.
Agitation of the probe in the unknown and standard solutions is required when using older-style probes to obtain reproducible results. Agitation ensures complete wetting of the internal electrodes by removing any air bubbles using the solution of interest. Newer-style probes have contacts that are sealed in epoxy resin to negate these concerns. Newer-style probes can be agitated to ensure probe contact with solution, but agitation is not required during measurements.
A. Calibration
-
Turn on the power supply or power switch.
-
Calibrate EC probe as directed by the manufacturer instructions.
-
Most meters and probes require specific calibration solutions and routines for proper calibration. Solutions of 100 µS, 1413 µS, and 12.9 mS are typically used for calibrating probes and meters.
-
Record the entire meter reading, including location of the decimal point and units of measure.
-
Many meters and probes will automatically adjust their range of measurement from microsiemens to millisiemens during operation.
-
EC readings obtained in microsiemens convert easily to millisiemens by multiplying result by 1000. Millisiemens readings are equivalent to millimhos per centimeter and decisiemens per meter.
-
Consult the manufacturer calibration instructions regarding specific meters and probes used at different facilities.
Assistance from the UF/IFAS Extension Soil Testing Laboratory
The ESTL is available with the following forms of assistance. Operation of county instrumentation can be checked at the ESTL. Instrument inspection by the manager of the ESTL should be done by appointment. Specific questions from UF/IFAS Extension faculty regarding pH and EC should be directed to the director.
Literature
Hanlon, E. A., B. L. McNeal, and G. Kidder. 1993. Soil and Container Media Electrical Conductivity Interpretations. CIR 1092. Gainesville: University of Florida Institute of Food and Agricultural Sciences. https://edis.ifas.ufl.edu/ss117
Mylavarapu. R. S. 2019. UF/IFAS Nutrient Management Series: UF/IFAS Standardized Nutrient Recommendation Development Process. SL189. Gainesville: University of Florida Institute of Food and Agricultural Sciences. https://edis.ifas.ufl.edu/ss401
Mylavarapu, R., G. Hochmuth, and G. Liu. 2017. UF/IFAS Standardized Nutrient Recommendations for Vegetable Crop Production in Florida. CIR1152. Gainesville: University of Florida Institute of Food and Agricultural Sciences. https://edis.ifas.ufl.edu/cv002
Mylavarapu, R. S., G. Hochmuth, C. Mackowiak, A. Wright, and M. Silveira. 2019. Lowering Soil pH to Optimize Nutrient Management and Crop Production. SL437. Gainesville: University of Florida Institute of Food and Agricultural Sciences. https://edis.ifas.ufl.edu/ss651
Mylavarapu, R. S., D. Wright, and G. Kidder. 2015. UF/IFAS Standardized Fertilization Recommendations for Agronomic Crops. SL129. Gainesville: University of Florida Institute of Food and Agricultural Sciences. https://edis.ifas.ufl.edu/ss163
Naumann, R., Ch. Alexander-Weber, R. Eberhardt, J. Giera, and P. Spitzer. 2002. "Traceability of pH Measurements by Glass Electrode Cells: Performance Characteristic of pH Electrodes by Multi-point Calibration." Anal. Bioanal. Chem. 374: 778–786. doi 10.1007/s00216-002-1506-5
Appendix
Soil pH Definition
The test for soil pH measures the acidity (pH less than 7.0) or alkalinity (pH greater than 7.0). If the pH is equal to 7.0, then it is called neutral. The pH scale ranges from 1 to 14; however, most soils in Florida fall between 4 and 8.5.
The following equation defines pH: Soil pH = -log(H+)
In words, soil pH is equal to the negative logarithm of the hydrogen ion activity. Activity is related to concentration and activity is usually somewhat less than concentration in soil systems. From a horticultural or agronomic viewpoint, plant roots are "experiencing" the activity, not the concentration, effects of the hydrogen ion.
Reference Soil Sample
A reference soil sample is a sample that is analyzed each time pH and/or EC are analyzed. The source of the sample should be such that sufficient volumes can be taken for use by the county laboratory. A sample of 3 to 5 pounds of air-dried soil can be used for several months by most county laboratories.
The sample should be completely air-dried and then sieved to pass a 2 mm screen. The sample should be thoroughly mixed and stored in a dry location. A working subsample may be kept in the laboratory in a cylindrical cardboard container for convenience (e.g., an ice cream container). When the subsample has been used to the point where the remainder occupies less than about 1/3 of the container, it should be refilled from the main sample. The subsample should be mixed before each use by inverting the container several times. Mixing prevents separation of the finer particles from the coarser particles.
Reference soil samples are also available for purchase by many reputable reference standard providers, NIST, or other sources on the internet.