Introduction
Millions of boxes of fruit and thousands of acres of citrus trees have been lost in freezes and frosts. Oranges are usually damaged when the fruit are exposed to temperatures of 28°F or lower for 4 hours or more. As the temperature gets colder or durations below 28°F get longer, damage to fruit, leaves, twigs, and eventually large branches increases. More than nearly any other factor, freezes have caused some of the most dramatic changes in fruit supply, availability, and price. Thus, any method that provides some cold protection can be of major importance to citrus growers.
Many cold protection methods have been used over the years. These methods include heaters, wind machines, fog generators, high volume over-tree irrigation, and low volume undertree microsprinkler irrigation.
High fuel cost has made grove heating during freeze nights prohibitively expensive except for high value crops. Wind machines are effective, but they require maintenance and need a strong temperature inversion for optimum effectiveness. Fog can provide cold protection, but light winds can blow the fog away from the grove and obscure nearby roadways. In south Florida where temperatures do not normally go far below freezing, high volume over-tree sprinkler irrigation has been used effectively on limes and avocados. In central and north Florida, temperatures are usually colder, and over-tree sprinklers should not be used on large citrus trees because the weight of the ice formed can break off limbs and cause tree collapse. With overhead systems, all leaves are wetted and susceptible to damaging evaporative cooling during low humidity or windy freezes. Many trees were killed in the windy 1962 freeze when overhead sprinklers were used because of evaporative cooling of wetted leaves.
Low volume undertree microsprinkler irrigation is an alternative method for partial frost protection and can be more affordable than other methods (Figure 1). Microsprinklers have proven effective during several freeze nights in central Florida tests. In addition to frost protection, microsprinklers can provide effective year-round irrigation. Microsprinklers, or spray jets, are small, low volume irrigation sprinklers that discharge 5 to 50 gallons/hour. In citrus groves, the most commonly used spray jets discharge from 5 to 25 gallons/hour and cover a diameter of 5 to 21 feet. Usually 1 or 2 microsprinklers per tree are installed at the ground level or on short risers. Unlike overhead sprinklers, microsprinklers do not commonly wet leaves and branches above a height of about 3 feet and do not usually cause serious limb damage.
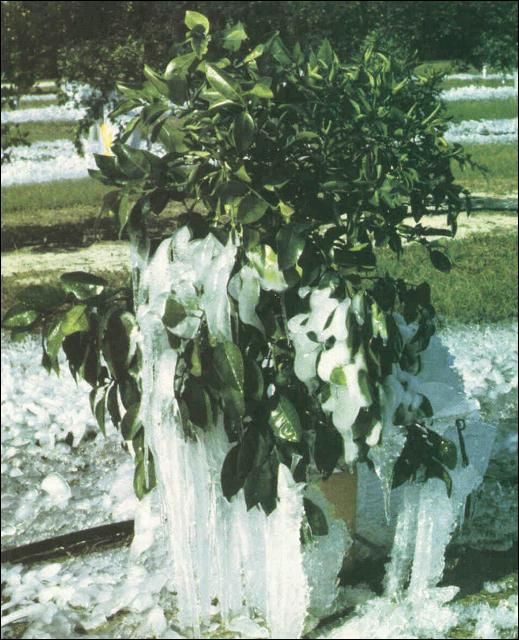
How Irrigation Works for Cold Protection
Various forms of irrigation have been used for frost and freeze protection for many years. When used properly, water can provide partial or complete cold protection for a number of crops. On the other hand, improper use of water can increase cooling or ice loading and cause greater damage than if no water were used at all. Because water can provide protection in one situation and cause damage in another, it is important to know what principles are involved in cold protection. To better understand what can happen when using water during a freeze, several commonly used terms need to be understood. With a knowledge of these terms, one can better evaluate the risks and benefits and successfully use irrigation for cold protection.
Heat of Fusion
The heat that is released when liquid water freezes to solid ice is called the heat of fusion. The amount of heat generated when water freezes is 1200 BTUs/gallon or 80 calories/gram of water frozen. As long as enough water is continuously applied to a plant, the heat generated when water freezes can keep the plant at or near 32°F (0°C). This is the principle used by strawberry, fern, or citrus nursery growers when they apply high volumes of water by sprinkler irrigation to protect their plants. At least 0.25 inch/hour or more is required for cold protection. With very low temperatures, low humidity, or high winds, more water must be applied to get adequate protection. Many citrus nurserymen need to apply water at rates of 0.40 inches/hour or higher.
Heat of Vaporization
The heat lost when water changes from a liquid to water vapor is called the heat of vaporization. At 32°F, the heat of vaporization is about 8950 BTUs/gallon or 596 calories/gram of water evaporated. Note that the heat of vaporization is about 7.5 times greater than the heat of fusion. This means that to maintain a stable situation when both freezing and evaporation occur, for every gallon of water that evaporates, 7.5 gallons of water need to be frozen to balance out the heat in the grove. Anything that promotes evaporation, such as low humidity and high wind speed, will promote overall cooling.
If the water application rate is high enough on the trunk of a young tree, it will be protected by the ice formation. However, on the edge of and outside of the iced zone, temperatures will not be maintained at 32°F, and those parts will probably be damaged or killed. Therefore, usually the tops of young trees or branches above the iced zone are more severely damaged after a freeze.
Dry Bulb Temperature (Tdb)
The dry bulb temperature is the temperature of the ambient air, which is the same thing as the normal air temperature read with a grove thermometer.
Wet Bulb Temperature (Twb)
The wet bulb temperature is defined as the lowest temperature to which air can be cooled solely by the addition of water. An example of wet bulb temperature is the temperature one feels when coming out of a swimming pool on a windy day. As long as a surface is wet while in the wind, its temperature will drop to the prevailing wet bulb temperature of the air.
The wet bulb temperature is between the dew point and dry bulb temperatures and normally closer to dry bulb than the dew point temperature. When the air is saturated with water vapor, the relative humidity is 100%, and all three temperatures (dew point, wet bulb, and dry bulb) are equal (Figure 2).
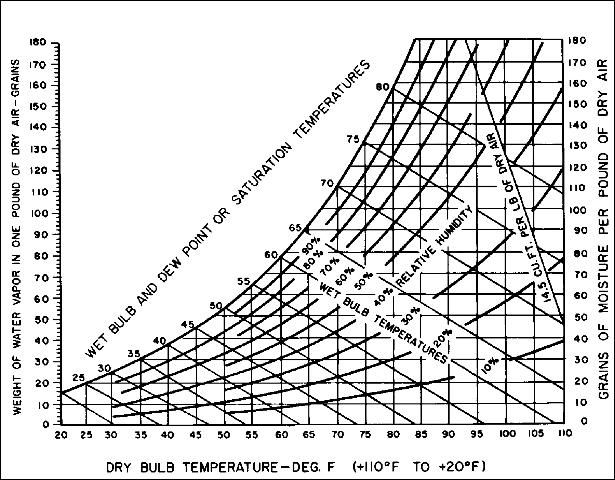
Humidity
Humidity refers to the amount of water vapor in the air. There are various ways to express humidity, but the most commonly used terms are relative humidity and dew point temperature.
Relative humidity (RH)
Relative humidity is the percentage (or ratio) of water vapor in the air in relation to the amount needed to saturate the air at the same temperature. Although commonly used, relative humidity is not the best measure of humidity because it depends on the air temperature. Warm air holds more water vapor than cool air.
Example:
Compare the amount of water vapor in air at 40°F and 70% RH to air at 90°F and 70% RH.
Using Figure 2, enter the graph at 40°F on the horizontal axis and go straight up until midway between the 60% and 80% RH curves. Then read the weight of water vapor in 1 lb of dry air on the left vertical axis—about 25 grains.
Following the same procedure for 90°F and 70% RH, the weight of water vapor in 1 lb of dry air is about 150 grains, or 6 times as much. Hence, the warmer air in this example holds about 6 times more water vapor than the cooler air even though both have the same RH.
Dew Point Temperature (Tdp)
The dew point temperature is the temperature at which dew begins to form or the temperature at which water vapor condenses to liquid water. It is also the temperature at which air reaches water vapor saturation. A common example of condensation is the water that forms on the outside of a glass of ice water. This happens because the temperature of the glass surface is lower than the dew point temperature of the ambient air in the room. Thus, some of the water vapor in the surrounding air condenses on the outside of the cold glass.
When referring to cold protection, dew point is one of the better ways to describe the humidity or amount of water vapor in the air. When the dew point is below 32°F, it is often called the frost point because frost can form when the temperature is below freezing. The dew point is important on freeze nights because water vapor in the air can slow the rate of temperature fall. With a relatively high dew point on a cool night, radiant heat losses from a grove are reduced, and the temperature may be expected to fall slowly. But if the dew point is quite low, the temperature can be expected to fall rapidly. Water vapor absorbs infrared radiation. Water droplets or fog are even more effective radiation absorbers than water vapor. Therefore, fog can reduce the rate of temperature drop on a frost night.
In addition to affecting the rate of radiation loss, the dew point is often a "basement" temperature, and the air temperature will not go much below it unless drier air moves in. The reason for this is that when dew condenses or ice forms, heat is given off. The amount of heat from condensation is the same as the heat of vaporization (about 8950 BTUs per gallon or 596 calories per gram of water) because vapor is changing to liquid water. This heat release during condensation slows the rate at which the air temperature drops. If dew forms, water vapor is condensed from the air, and the humidity or dew point of that air is lowered. This is how the evaporation coil in an air conditioner removes water vapor and dehumidifies the air.
Dew point temperatures are commonly higher on the coasts than they are inland. In the central Florida citrus belt (e.g. near Lake Alfred), dew point temperatures on a moderate frost night can be in the vicinity of 20 to 30°F. On more severe freeze nights, dew point temperatures can be 10°F or lower. For example, in the damaging Christmas 1983 and January 1985 freezes, dew point temperatures in Lake Alfred approached 5°F, which is exceedingly low for central Florida.
When wet bulb and dry bulb temperatures are known, Figure 3 and Figure 4 can be used. (Figure 4 for Twb < 40°F and Fig. 5 Twb 40–60°F). Locate Tdb on the bottom axis and go up to the curve with the measured Twb. The dew point temperature (Tdp) is read from the vertical axis value from the intersection of the Tdb and Twb lines.
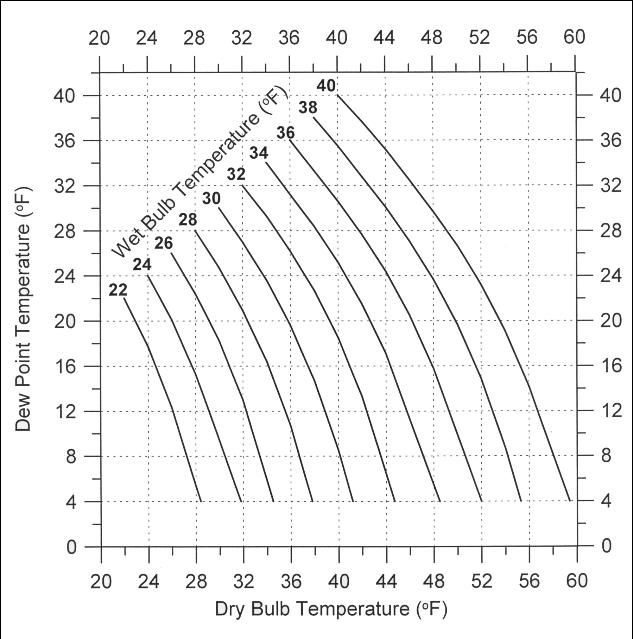
Example:
For the following temperatures, determine the dew point temperature.
Tdb = 60°F
Twb = 46°F
Using Figure 4, locate 60°F on the bottom axis and go up to the Twb = 46°F curve. The value of Tdp can be read from the vertical axis as 30°F.
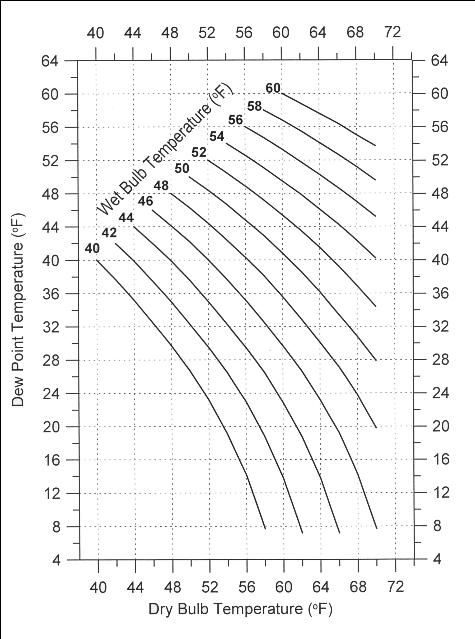
If Tdb and the relative humidity (RH) are known, Tdp can be determined from Figure. 5. Locate Tdb on the bottom axis and go up to the line that represents the measured relative humidity. Tdp is read from the vertical axis value from the intersection of the Tdb and RH lines.
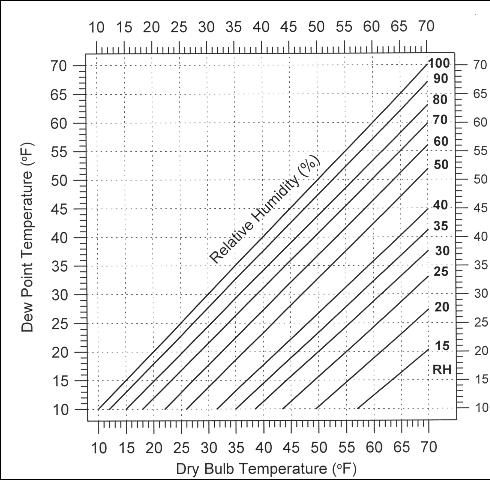
Example:
For a dry bulb temperature of 45°F with a relative humidity of 50%, determine the dew point temperature.
Select Figure 5 (for relationships between Tdb, RH, and Tdp). Enter the figure on the bottom axis at Tdb = of 45°F and go straight up until the RH = 50% line is intersected. Read the Tdp from the vertical axis as Tdp= 32°F.
Wind Chill
Wind chill refers to the cooling effect of moving air on a warm body and is expressed in terms of the amount of heat lost per unit area per unit of time. Wind chill was developed to estimate heat loss rate from humans and other warm blooded organisms. It does not apply to plants or vegetation. Even though wind chill does not apply to plants, wind can remove heat from a grove rapidly. In a windy freeze, temperature of a dry leaf is usually fairly close to air temperature. If the leaf is wet and water is not freezing on it, the leaf can theoretically cool to the wet bulb temperature. Thus, the length of time a grove will be at low temperatures can be longer on a windy night than on a calm one. Thus, more damage can potentially occur during a windy freeze.
Psychrometer
A psychrometer is a device used to determine atmospheric humidity by reading the wet bulb and dry bulb temperatures. The wet bulb thermometer is kept wet by a moistened cotton wick or sleeve. With a psychrometer, one determines how much cooler the wet bulb is than the dry bulb and then calculates humidity by using appropriate graphs or tables. "Psychros" comes from the Greek work meaning "cold," and hence a psychrometer measures humidity by determining how much colder the wet bulb thermometer is than the dry bulb thermometer.
For an accurate reading, the wet bulb thermometer must have air moving over it. With a sling psychrometer, air flow is created by rotating the two thermometers through the air by hand (Figure 6). The sling psychrometer consists of two liquid-in-glass thermometers. One thermometer measures the air (dry bulb) temperature while the other one measures the wet-bulb temperature. After the wick is dipped in distilled water, the sling psychrometer is whirled around using the handle.
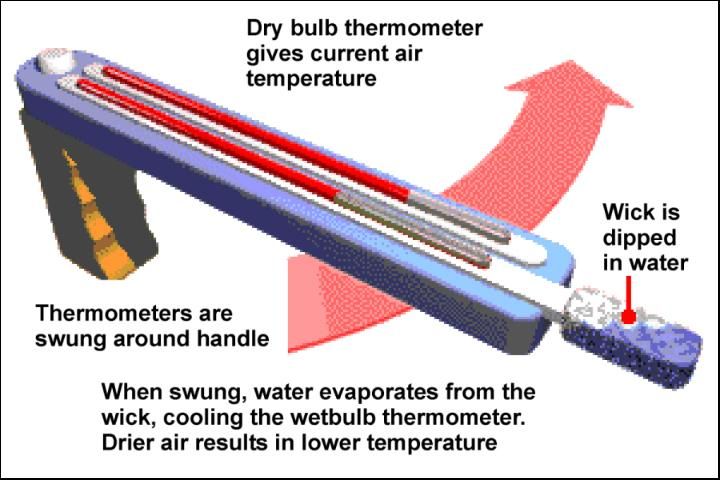
Water evaporates from the wick on the wet-bulb thermometer and cools the thermometer due to the latent heat of vaporization. The wet-bulb thermometer is cooled to the lowest value possible in a few minutes. This value is known as the wet-bulb temperature. The drier the air is, the more the thermometer cools and thus, the lower the wet-bulb temperature becomes. With a fan ventilated psychrometer, a fan blows air across the two thermometers. Fan ventilated psychrometers cost more than sling psychrometers, but they are more convenient to operate on freeze nights.
Sling psychrometers work well at temperatures above freezing, but are more difficult to operate at temperatures below freezing. The reason for this is that at temperatures much below freezing, the water on the wet bulb freezes, releases its heat of fusion, and raises the wet bulb temperature to around 32°F. Eventually, it is possible to get a "frost" wet bulb temperature if one rotates the sling psychrometer long enough. A battery powered fan psychrometer avoids some of the problems of a sling psychrometer but it may take 20 minutes or more to get a valid wet bulb temperature when the air is below freezing. A slightly different chart is used for humidity calculations when the wet bulb sleeve has ice on it.
Effectiveness of Microsprinklers
Microsprinkler irrigation is more effective for cold protection when high volumes of water are used. A system that delivers the maximum amount of water per acre and is practical or affordable is best for frost protection. Irrigation rates of 2000 gallons/acre/hour or 33.3 gallons/acre/minute are recommended. This can be accomplished with one 20 gallon/hour jet or two 10 gallon/hour jets per tree in a grove with 100 trees/acre (Figure 7). If there are 200 trees per acre, then one 10 gallon/hour jet is adequate. Rates below this level will provide some protection but not as much as higher rates. Application rates of 3000 gallons/acre/hour or more are more effective at lower temperatures.

At high application rates, average warming with spray jets at a 4-foot height is only 2 to 3°F. Spot readings have occasionally shown temperature increases of 4°F or more, but 1°F or less is also common during freeze nights. At heights greater than 8 feet, warming is usually less than 1°F. When compared to a non-irrigated area, lower volume systems provide slight warming, but higher volume systems (2000 gallons/acre/hour) provide more warming. Low irrigation rates can provide some, although not much, protection on calm frost nights. While a small amount of water can provide a little protection, it is generally best not to go below 10 gallons/hour/tree. Emitters that put out less than 10 gallons/hour usually have small orifices that can plug easily. If a jet next to a tree is plugged, that tree will suffer more damage or may be killed.
Example:
Determine the gallons per hour per acre available for freeze protection for a block that has 1 microsprinkler/tree that discharges 12 gph. Trees are planted at a 12 ft in-row and 24 ft across-row spacing.
Determine the number of trees/acre
trees/ac = 43,560 ft2/ac x 1 tree/(12 ft x 24 ft) = 151 trees/ac
Calculate gal/hr/ac
gal/hr/ac = 151 trees/ac x 12 gal/hr/tree = 1812 gal/hr/ac
or, use Figure 7
Enter the figure on the bottom axis at a density of 151 trees/ac and go straight up until the 12 gph line is intersected. Read the per acre application rate from the vertical axis as 1800 gal/hr/ac.
Microsprinklers can provide some protection to leaves and wood, particularly on the lower and inner part of the canopy. A dense canopy tends to retain heat from the soil and provide better protection than a thin canopy. Damage will commonly be seen on the outer and upper parts of the tree after severe freezes. Since fruit is more sensitive to cold temperatures than leaves or wood, microsprinklers generally do not protect the fruit. At higher volumes, spray jets will help protect fruit a little better than no irrigation, but generally microsprinkler irrigation is best for tree protection rather than fruit protection.
There is a limit to the effectiveness of microsprinklers. Factors such as tree health, rootstock, and cold acclimation affect tree survival. Depending on volume of water applied, the lower limit of effectiveness for microsprinkler irrigation is around 17°F. The lower parts of young trees have been protected to even colder temperatures, but damage usually increases as it gets colder.
Young Tree Protection with Microsprinklers
Microsprinklers have been effective in protecting the bud union and lower portion of young trees. In young trees, the microsprinkler protects the lower trunk by the direct application of water. When water freezes, it releases heat. If the application rate is high enough, the freezing water will maintain the trunk at a temperature near 32°F. The spray jet must be close enough to the young tree so that water sprays directly on the trunk and lower part of the tree. Recommended distances between the trunk and the jet are 1 to 2.5 feet. If the jet is too far away from the young tree, wind can blow the water away. If the water freezes before it hits the tree, milky white ice can form on the tree. Protection under milky ice is usually not as good as under clear ice. During most Florida freeze nights, the wind comes from the north or northwest making it best to put the jet on the north or northwest (upwind) side of the tree. In this position, the wind will carry the water into the tree and not blow it away from the tree.
It is common for protection to be seen only in the iced zone on young trees. Damage commonly occurs, particularly in severe freezes, above the iced zone or where no water was run. Figure 8 shows a row of green trees where microsprinklers were run during a freeze and a freeze-damaged brown tree where no water was run during the freeze. Young trees are usually more sensitive to cold and do not retain heat as well as mature trees. Therefore, protection down to 17°F cannot always be assured even if the tree is in good health.
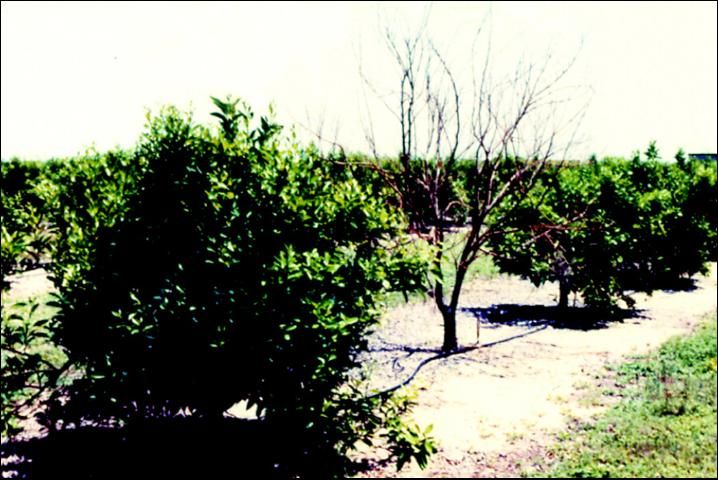
Insulating tree wraps placed around the trunks of young trees slow the rate of temperature fall. Tree wraps alone provide some trunk protection. Tree wraps in combination with microsprinkler irrigation provide even better cold protection insurance. If the irrigation system fails during the night, the tree wrap (particularly if it is a good insulator or has enclosed water pouches) can slow the temperature drop and protect the tree longer.
Operation of Microsprinklers on Freeze Nights
For cold protection, a microsprinkler system must be designed to provide water to the entire block or grove all at once. An irrigation system that can apply water only to smaller zones is not satisfactory for freeze protection. Hence, a system designed for freeze protection is initially more expensive to install because it handles a larger volume of water.
Compared to higher pressure overhead systems, microsprinklers operate at relatively low pressures of 20 to 25 psi. Because of this low pressure and the small openings in the emitters, spray jets can freeze up if they drop below 32°F before the water is turned on. Hence, the water should be turned on before the temperature reaches 32°F so the jets do not freeze. On frost nights, it is recommended that microsprinklers be turned on when the air temperature reaches 36°F. Be careful of thermometer placement, because in low-lying cold pockets, the ground surface can be below 32°F when it is 36°F at the thermometer location. When spray jets ice up, they are very difficult to thaw and usually the emitters must be replaced in the field.
Once the microsprinklers are turned on, the system must keep running all night. If the system stops or fails when the temperature is below 32°F, it will be very difficult to restart the system because the emitters can rapidly freeze up. The situation becomes especially critical for young trees if the system fails when the temperature is below freezing. Shortly after the water stops spraying on a young tree, the trunk temperature can drop to the wet bulb temperature.
If the irrigation system stops, the trunk can rapidly drop below the actual air temperature by evaporative cooling. Thus, more tree damage can occur than if the jets were never turned on. Even if the system has only stopped for a short time, many of the emitters probably will have frozen and it will be difficult to get the entire system fully operational again. Because of evaporative cooling, damage can be greater to trees in the area where a system stopped during a freeze night than where the system kept operating continuously. Do not turn off a system when the temperature is near the critical tree killing temperature. If the pumping system is unreliable or if the pump is electrically driven in an area that commonly has electrical interruptions on freeze nights, it may be wise to convert to a more reliable system.
In the morning, when the temperature warms up, it is not necessary to wait until all the ice has melted before turning off the system. When the wet bulb temperature is above 33°F, the system can be safely turned off with no damage to the trees. At temperatures below freezing, it is possible for the wet bulb to read 32°F and actually be warmer than the dry bulb thermometer. This occurs because the water in the wick freezes, releases the heat of fusion, and raises the wet bulb temperature to 32°F. Therefore, one should wait until the wet bulb temperature reaches 33°F before turning off the irrigation system.
Generally speaking, if the air temperature (dry bulb) has risen to 40 or 45°F, the irrigation system can be turned off safely. If the grove contains only mature hardened off trees, the system can be turned off at 40°F. Under the most adverse conditions of low dew point and high wind, the grower may want to wait until the temperature is above 45°F. If it is a two-night freeze and the daytime temperature never gets above 40°F, then the system should be run continuously throughout the day and into the second night. If drainage or water conservation is of major concern, the system can possibly be turned off slightly before 40°F under less severe non-windy conditions, but that increases the freeze damage risk.
Why Microsprinklers Provide Cold Protection
Several factors contribute to the cold protection effectiveness of microsprinkler systems. Most well water in Florida is around 68 to 70°F. This warm water contributes a small amount of sensible heat to the grove at it drops from the initial temperature to 32°F. When temperatures drop below freezing, the latent heat of fusion is released when the water freezes. Depending on the amount of ice that forms, the heat released can raise temperatures in the lower part of the canopy. Water has a high heat capacity and can store a fair amount of heat. Therefore, a moist soil can hold more heat than a dry soil.
Microsprinklers can also raise the dew point or frost point temperature in the grove. When the temperature drops to the frost point, heat is released as the water vapor is converted to ice crystals. When the grove air temperature reaches the dew point temperature, the rate of cooling slows down because heat is released as the water vapor in the air condenses. It has been suggested that microsprinklers can provide some protection above the spray zone because moist air rises and condenses higher up in the canopy. The heat of condensation (8950 BTUs/gallon) may help warm the upper canopy and protect more of the tree.
Depending on the dew point temperature, microsprinklers can sometimes create fog on cold, calm nights. Fog is beneficial for frost protection and if the fog is dense enough and the droplets are of the proper size, the rate of cooling can be slowed since fog can act like a blanket and reduce the rate of radiation loss. Similarly, clouds (which consist of water droplets) can act like a blanket and slow radiation loss.
Operation on Windy Nights
Like other methods used for citrus cold protection, microsprinklers are less effective during windy or advective freeze nights. They provide little or no protection to mature trees. There is a risk when using water during windy or low humidity freezes. When dew point temperatures are low and winds are high, high evaporation rates can occur and cool the wetted part of a tree below the air temperature. This happened in the windy 1962 freeze. Where overhead sprinklers were used, evaporative cooling occurred and trees were killed. The heat lost by evaporative cooling is approximately 7.5 times greater than the heat gained by ice formation.
The irrigation application rate on the wetted area influences the level of protection. A higher application rate can protect trees to lower temperatures. One way to increase the application rate is to reduce the spray pattern size. This can be done by changing a 360° full circle spray pattern to a half circle (180°) or quarter circle (90°) pattern. This essentially doubles or quadruples the application rate by concentrating the amount of water on a smaller area. With a higher application rate, the protection level is better. Because of changing winds, a half circle cap may do a better job of directing water into the young tree than a quarter circle cap.
Summary
Undertree microsprinkler irrigation is an affordable alternative to other forms of cold protection. It does not provide complete protection and generally will not protect fruit. Weak trees will receive little or no protection. On calm nights, microsprinklers have given partial protection to healthy and well-hardened trees down to 17°F. On windy severe freeze nights, little if any protection will be provided for mature trees, and only higher volume systems will provide protection for the lower portion of young trees.
References
Buchanan, D. W., F. S. Davies, and D. S. Harrison. (1982). High and low volume under-tree irrigation for citrus cold protection. Proc. Fla. State Hort. Soc. 95:23-26.
Parsons, L. R., T. A. Wheaton, N. D. Faryna, and J. L. Jackson. (1991). Elevated microsprinklers improve protection of citrus trees in an advective freeze. HortScience 26(9):1149-1151.
Parsons, L. R. and T. A. Wheaton. (1987). Microsprinkler irrigation for freeze protection: Evaporative cooling and extent of protection in an advective freeze. J. Amer. Soc. Hort. Sci. 112:897-902.
Parsons, L. R., T. A. Wheaton, D.P.H. Tucker, and J. D. Whitney. (1982). Low volume microsprinkler irrigation for citrus cold protection. Proc. Fla. State Hort. Soc. 95:20-23.