Introduction
The purpose of this publication is to summarize the historical Florida research literature on nitrogen, phosphorus, and potassium fertilization of watermelon. This document is not meant to present new fertilization recommendations. The intent of this document is to review literature from all sources that pertains to commercial watermelon fertilization in Florida's growing conditions. Many of these experiments were conducted on grower fields to measure crop response to fertilizer in "real-world" commercial production systems. For watermelon, as many as a fourth of the most recent studies were done on commercial farms.
This publication documents the previous written literature, some of which now appears only in updated modern electronic format. All publications, including the reviewed, older, paper-format papers, will be placed in PDF on the website https://bmp.ifas.ufl.edu for future reference. The research publications over the years have provided the scientific basis for the Florida Extension watermelon fertilization recommendations that further formed the basis for best management practices (BMP) that address water quality protection. Watermelon fertilization recommendations have been based on actual field measurements, and a compilation of the literature assists with making valid fertilizer recommendations that are both commercially viable and reduce the risk of environmental consequences in adjacent water bodies. As environmental regulation becomes more commonplace, it becomes more important that fertilization recommendations be based on published research.
Fertilizer recommendations not only contain the recommended rate of fertilizer but also the management strategies for getting the most out of the fertilizer investment while protecting the environment. These principles for fertilization of vegetables are summarized by Hochmuth and Hanlon (2010a). Rate is only a part of the modern fertilizer recommendation. Sound fertilizer recommendations also consider fertilizer materials, placement, and timing, among other aspects (Hochmuth and Hanlon 2010b).
The fertilization recommendation addresses commercial yield and quality, the economics of crop production, and protection of the environment. Equally important is to have a mechanism available to the grower to adjust fertilization practices during the season due to leaching rains and extension of the growing season for additional harvests when market conditions are favorable. To address all of these concerns, UF/IFAS vegetable recommendations are given as a single target fertilizer rate that is projected to be sufficient for meeting the crop fertilizer needs for most growing seasons. This single target fertilizer rate is a recommended starting point and has been used historically in all vegetable fertilizer recommendations in Florida, as well as around the country. The target recommendation approach with footnotes was used by Montelaro (1978) and confirmed by Hochmuth and Hanlon (1995; 2000). The target value was derived from numerous fertilizer studies and represents a reasonable fertilizer rate that reflects the average maximum crop responses from all of the fertilizer research, not the extremes in responses. However the recommendation process recognizes some growing seasons are different, due to more leaching rains or increases in crop value than can lead to prolonged harvest windows. Hence the target fertilizer amount is accompanied by a series of footnotes that explain the addition of supplemental fertilizer during the growing season, which includes addressing leaching rains and additional harvests near the end of the season. It is logical to select a single target rate based on research that avoids excessive fertilizer applications that often reduce crop nutrient efficiency and increase the potential for environmental degradation.
This literature review includes all available published documentation concerning watermelon yield and fertilizer use in Florida. We chose to present all the research without being selective, as that would introduce bias to the presentation. Most of the older literature with mixed N-P-K fertilizers is captured in separate figures in the review document. Inclusion of all Florida literature documents the development of the commercial production system and the fertilizer recommendations. Average commercial yields have been increasing, primarily due to the proper selection of new cultivars with traits that resist disease and pest pressures and more intensive farming practices. Since the basic plant has not changed, nutrient requirements of the plant have also been slow to change. In fact, nutrient use efficiency has increased while fertilizer rates have not. This gain in nutrient efficiency (plastic mulch, drip irrigation) also avoids adverse environmental consequences.
This publication is an updated version of a previous research literature review by the same title (Hochmuth and Cordasco 1999, reviewed 2008) that covered research through 1996. This new review document adds the research reports conducted since 1996 and expands on the role of irrigation in managing fertilizer for crop production efficiency and environmental protection. The audience for this publication includes educators, such as Extension specialists and agents, and the commercial vegetable producers, consultants, and governmental agencies.
Watermelon is one of the ten most important vegetable crops in Florida. Florida watermelon production in the 2007–2008 season totaled 8,613,000 hundred weight (cwt). Crop value was $140,392,000 from 26,700 planted acres (26,100 harvested acres) in Florida (Fla. Dept. of Agr. and Cons. Serv. 2009). The average yield was 330 cwt per acre.
More than sixty years of watermelon fertilization research has been conducted in Florida. During this time many changes have occurred in watermelon production practices, including changes in cultivars and the introduction of new cultural systems such as polyethylene mulch and drip irrigation. Watermelon crop and fertilizer management recommendations, such as plant and row spacing, have changed with time in keeping with new developments in research (Montelaro 1978; Hanlon and Hochmuth 1992; Hochmuth 1988; 1996a; 1996b; Hochmuth et al. 1988; Hochmuth and Hanlon 1989, 1995a; 1995b; 2000; Kidder et al. 1989; Maynard 1992; Olson et al. 2010; Simonne and Hochmuth 2010; Stall and Showalter 1985). Fertilizer is a significant cost input. The most current recommendations for nutrient management in watermelon production are presented in the Commercial Vegetable Production Handbook for Florida (Olson and Simonne 2010). These recommendations have undergone several revisions over the years (Montelaro 1978; Kidder et al. 1989; Hochmuth and Hanlon 1995; 2000).
The early research focused largely on yield and fruit quality (size and shape) in response to fertilization. Since the early research was conducted, and since the first review of the literature in 1999, there has been increasingly strong interest in the incorporation of more environmental impact (water quality) study into the fertilizer research. The state (Fla. Dept. of Agr. and Cons. Serv. 2005) has been formalizing BMPs and encouraging growers to implement BMPs. Part of the definition of a BMP includes references to the consideration of both economics and environmental components and that a BMP embodies the best available science.
Data Summary Method
To evaluate watermelon yield responses to variable rates of fertilizer, a method was needed to standardize the numerous units used for quantifying statewide yield results such as pounds/acre, tons/acre, metric tons/hectare, or hundred-weight (100-lb units)/acre (cwt/acre). In addition, vegetable yields varied over years and season, cultivar, and location in the state. Relative yield (RY), a calculated percentage, was chosen as the unit to express watermelon yield responses to fertilization. Relative yield is an accepted scientific method for summarizing and presenting data across wide sources and reports (Brown 1987).
This paper uses percent relative yield to take into account the changing conditions associated with season, irrigation, pest pressures, and other cultural practices that directly affect yield. No single study is favored by the use of relative yield in the summaries of data. Percent relative yield has been critically reviewed and included in all modern books and papers dealing with plant yield and added nutrition completed by researchers in different locations and spanning large periods of time.
In his book, C. Black (1992) summarized the advantages and disadvantages of the RY approach. There are valid statistical concerns about RY, but he concluded that, when applied properly and cautiously, the RY approach can be useful in displaying general relationships. Black demonstrates examples in his book where RY is helpful and where it is not. We chose the RY approach because we wanted to display the historical data without making biased decisions about what to include and what not to include in the presentation. Plotting absolute yield data in original units obviously would result in a scattering of data rendering any general interpretation impossible. Black (1992) points out that decisions can always be improved with further research, but the data on hand are the best we have at the time. The highest yield for each fertilizer experiment was assigned a 100% value, and other yields were expressed as a percentage of the highest yield. The actual yield corresponding to 100% RY was presented in cwt/acre units. The RYs were plotted against rates of nutrient to determine how watermelon yields responded to fertilizer in Florida. The RY presentation allowed data from a variety of experiments to be included in the graphical summary of yield responses. For most studies, RYs of 90 to 100% were not significantly different.
Sometimes the argument is made that growers have expectations of greater yields than those obtained in research projects. It should be pointed out that realistic, regularly obtainable yields might be different from expected yields or "yield goals." Research on this subject has documented that 20% of growers actually reached their yield goals, and only 50% reached 80% of their yield goals (Schepers et al.1986). Fertilizer rates should not be set on yield goals but rather on realistic goals based on research. This practice is not recommended in Florida, so the effect of overfertilization has been avoided by grounding expectations in measurement of observed yields. Scientists throughout the years have conducted many replicated demonstration studies in growers' fields, with true commercial production practices, and those studies are included in this review. Excessive fertilizer was justified in times past when fertilizer was viewed as inexpensive insurance against yield loss. Research with numerous crops has shown that nutrient use efficiency declines as nutrient rate, especially N, increases. Even given the best of production systems, N use efficiency rarely exceeds 70% of the applied N. Concepts and practices for managing nutrients in vegetable production were summarized by Hochmuth (1992; 2000). Further, it has been suggested that the yields in older studies were much lower than yields obtained today. However there are older research reports from the 1950s and 1960s where yields were as high as yields achieved today.
Fertilizer rates are expressed on a per-acre basis (amount of fertilizer used on a crop growing in an area of 43,560 sq ft). Changes in bed spacing often lead to needed changes in fertilizer amounts. For example, to maintain the same amount of fertilizer in the plant bed for a crop on 6-foot bed spacing as a crop with 4-foot bed spacing would mean an increase by a factor of 1.5 in the "per acre" rate of fertilizer for the crop growing in beds spaced 4-foot on center. The important aspect is to have the same amount of fertilizer per linear-bed-foot of bed because it is the bedded area where most of the roots are contained. This linear-bed-foot system is used by the University of Florida Extension Soil Testing Laboratory to express fertilizer rates. The concept is explained by Hanlon and Hochmuth (1989) and by Hochmuth and Hanlon (2009). Fertilizer rate expressions used in this review summary and its figures are those rates presented by the various authors in their research papers. Most authors express rates on a per-acre basis, irrespective of variations in bed spacings among reports or experiments. Authors of a few reports chose to use the linear-bed-foot system (standard bed spacing for watermelon is 8 ft) to standardize fertilizer rate expressions across experiments and planting patterns. We attempt to specify planting patterns and fertilizer rates for each experiment as far as we can determine from each report. Watermelon production systems have varied with time largely due to differences in irrigation systems. Hence the irrigation method is referenced for the fertilizer research studies. We have organized the N section according to mixed fertilizer studies and N-only studies. Within the N-only studies, we have presented the research according to the irrigation system used in the study.
Nitrogen-Mixed Fertilizer Trials
Mixed fertilizers were often used in early watermelon experiments to establish crop fertilizer requirements (Figure 1). Attributing crop response to a single nutrient, however, could not be done with certainty from research with a blended N-P-K material. Since N is usually the most limiting major nutrient in sandy soils, yield responses in mixed studies are considered here as responses to N. Polyethylene mulch was not used in these studies unless specified. Watermelons planted at the Horticultural Research Unit near Gainesville and the Suwannee Valley Research and Education Center in Live Oak received either 30 or 60 lb/acre N from a 6N-8P2O5-8K2O commercial mix (Nettles and Lundy 1958). Sprinkler irrigation was applied once in Gainesville. No irrigation was used in Live Oak.
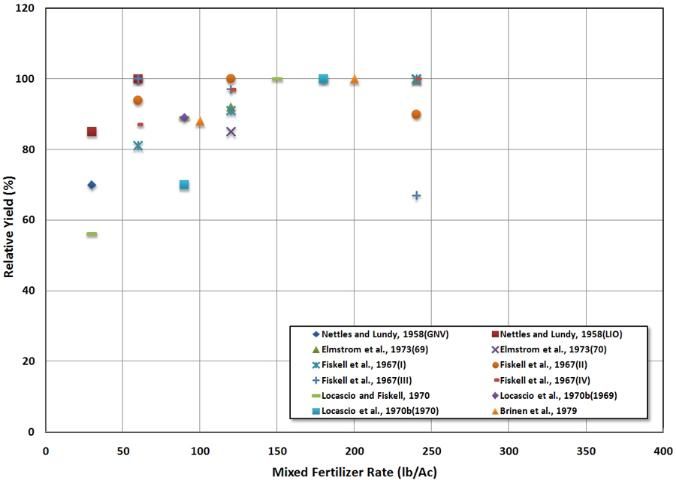
Researchers indicated additional N that was applied may have increased watermelon yields in these 1958 trials where optimum yields were obtained with 60 lb/acre N (262 and 147 cwt/acre, 100% RYs at Gainesville and Live Oak, respectively). Relative yields with 30 lb/acre N were 70 and 85%, respectively. An increase in applied N from 30 to 60 lb/acre resulted in 8% greater early yields and a 5% increase in average fruit weight to 25 lb/fruit at Gainesville. Fruit weight was not presented for Live Oak. Yields in Gainesville increased only 6% (treatment mean = 230 cwt/acre) with fertilizers containing 25% organic material from caster pomace and tankage compared to yields with inorganic sources alone. The inorganic fertilizer blend resulted in greater yields when applied entirely at preplant than when applied in two applications.
Subsurface irrigation (Figure 2) was used on 90% of Southwest Florida watermelon fields at the time of an early research project in Immokalee in 1959–1960 (Everett 1960). Watermelon crops often extended through 8 to 10 harvests and required frequent topdressings of N and K, which were generally applied by aircraft at two-week intervals. Watermelon yield responses were evaluated for varying N rates from 90 to 325 lb/acre and for N sources, NH4NO3, (NH4)2SO4, NaNO3, and a 30% organic-N commercial fertilizer. Experiments were conducted in 1958, 1959, and 1960 at the University of Florida South Florida Field Laboratory near Immokalee. Mixed fertilizers, 4-8-8 or 6-8-8 (N-P2O5-K2O), were banded on both sides of the row and beneath the level of the row in three applications: at planting; when vines were 12 to 18 inches in length; and when vines were 3 feet in length. Topdressing fertilizer applications (10 to 15 lbs lb/acre N) were broadcast to simulate airplane application. One topdressing application was made in 1958, three in 1959, and four in 1960. Rows were spaced 10 feet apart.
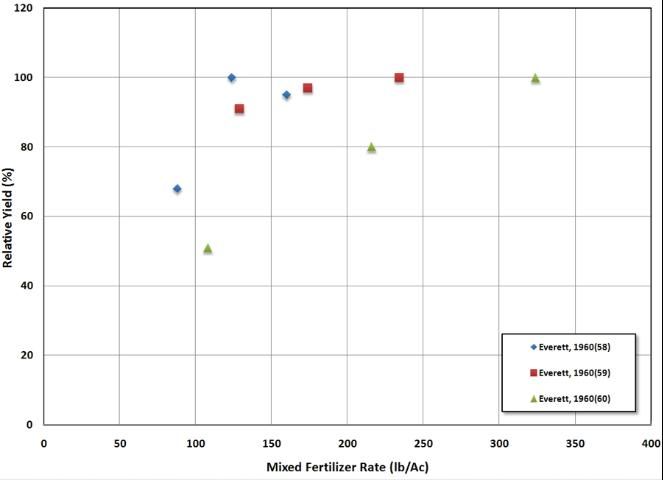
Marketable watermelon yields responded to fertilization with 100% RY at higher N rates each year. Highest yields occurred with 124 and 324 lb/acre N (306 and 458 cwt/acre, 100% RY) in 1958 and 1960, respectively. Yields were not significantly affected by increasing N rates in 1959. The authors noted that N rates higher than 150 lb/acre were required in 1960, a dry season when frequent irrigations likely leached N.
Consistent and significant positive yield responses were observed for organic versus inorganic N sources during the three seasons (Everett 1960). Plants grown in 1959 with the commercial 30% organic-N mix, 4N-8P2O5-8K2O, applied at 90 lb/acre N yielded 25% more marketable fruit (720 cwt/acre) than the average high yields with N rates of 110, 150, and 200 lb/acre from plots fertilized with the inorganic source, 6N-8P2O5-8K2O, or (NH4)2SO4. The same organic N mixed fertilizer applied at three N rates (80, 160, and 240 lb/acre in 1960) resulted in an average yield of 788 cwt/acre, double the average yields with the same rate of (NH4)2SO4. Research did not establish yield response to trace elements within the organic mix. Nitrogen sources leading to high yields also resulted in the highest NH4 to NO3 ionic ratios from soil samples taken throughout the season. The NH4 to NO3 ratio was 1.09 with the (NH4)2SO4 fertilizer and 0.37 with the organic mixed fertilizer, both of which led to high watermelon yields. Lower yields and lower ionic ratios of 0.19 and 0.11 occurred with NH4NO3 and NaNO3, respectively. Advantages of the organic mix were a more constant supply of NH4 and NO3-N with decomposition, reduced N leaching due to rain or irrigation, and 75 and 65% of yields harvested early (for best prices), as opposed to an average 55 and 28% with inorganic sources (1959 and 1960, respectively). These studies showed that watermelon yields were greatest with 150 to 200 lbs/acre N except when more was needed in a dry year probably due to leaching from irrigation.
In 1964, researchers determined that copper was the yield-enhancing micronutrient present in organic fertilizer (Locascio et al. 1964). Copper deficiency symptoms, resulting in failure to set fruit in cases of severe deficiency, were observed in plants in Gainesville. All plants were fertilized with 120 lb/acre N from 4N-8P2O5-8K2O. Plants fertilized without the addition of copper, including a gypsum-containing treatment, resulted in yields significantly lower (38 cwt/acre) than plants with treatments containing copper (56 cwt/acre) from either inorganic sources (Cu EDTA and Frit 503) or an organic source (Chicago sludge). Plants were spaced 5 feet apart and rows 9 feet apart in a 1964 Immokalee experiment. Yields increased linearly as copper concentrations in mature leaves taken at fruit maturity increased from 4 to 10 ppm. Higher leaf-tissue copper concentrations (7 to 8 ppm) resulted with inorganic copper sources applied at 3 lb/acre copper. Fruit yields with these inorganic copper sources were 800 cwt/acre of watermelon compared to 653 cwt/acre with organic sources of applied copper (rows 10 feet apart). Additional research (Locascio et al. 1966; Locascio and Fiskell 1966) confirmed the importance of copper at 3 lb/acre, derived from the native soil or from fertilizer, for the production of high-yielding watermelon crops.
Experimentation with fertilizer placement in 1967 resulted in significant changes in recommendations for fertilizer application practices with watermelon (Fiskell et al. 1967; Locascio et al. 1970a). A 6N-8P2O5-8K2O fertilizer was applied with N rates of 60,120, and 240 lb/acre at four sprinkler-irrigated sites. Soils at three sites were Immokalee, Leon, and Lakeland fine sands; soil type at the fourth site was not specified. Treatments were three applications of single- or double-banded fertilizer; a broad-band application with 80% of the fertilizer placed in a 10-inch- wide band 2.5 inches below the seed; and broadcast fertilizer, half at preplant and half at early-runner stage. Plastic mulch was applied at the fourth site, with the 240 lb/acre N treatment, while the remaining sites were unmulched. Plant rows were spaced 9 to 10 feet on center.
Yields were generally greatest with 120 lb/acre N, averaging 96% RY. Yields ranged from 174 to 476 cwt/acre with this N rate across sites (Fiskell et al. 1967). Yields increased to 100% RY with 240 lb/acre N at two locations but were reduced to 90 and 67% RY with 240 lb/acre N at the remaining locations. The recommended N rate for watermelon production at the time of this research was 120 lb/acre. A significant result of this study (Fiskell et al. 1967) was the finding of improved yields with modified broadcast fertilizer placement compared to band placement, and this information was incorporated in the recommendations for watermelon production. Yields with broadcast fertilizers averaged using four experiments and three N rates were 33% higher than yields where single- and double-banded fertilizer applications were used and 53% higher than where fertilizer was placed in a broad-band at bed center. The latter placement method was the most commonly used technique at that time. After harvest, soil-soluble salt levels were correspondingly lowest with the broadcast treatment. Single- and double-band-placed fertilizers resulted in equal yields, while broad-band-placed fertilizers resulted in the lowest yields. Seed germination failure, seedling mortality, and delayed maturity combined to reduce yields with broad-band treatments due to soluble-salt concentrations two and three times higher than with other placement methods three months after planting. Yields with plastic mulch were similar to yields with no mulch at the fourth site.
Early experimentation with blended 6N-8P2O5-8K2O fertilizer gave way to yield-comparison studies with sulfur-coated urea (SCU) compared to uncoated urea, and sulfur-coated KCl compared to uncoated KCl (Locascio and Fiskell 1970). Nitrogen rates used in the study were 30, 60, and 150 lb/acre on beds spaced 9 feet on center. Trials were conducted near Gainesville on Leon fine sand with factorial arrangements of the above treatments applied broadcast/incorporated preplant. In a fifth treatment, equal amounts of uncoated urea and KCl were side-dressed at preplant, at thinning, and at early runner stage.
Despite heavy rainfall (nine inches) in the second month after planting, and a dry third month requiring three acre-inches of water from overhead irrigation, high yields resulted with 150 lb/acre N (524 cwt/acre, 100% RY). Preplant SCU applications resulted in 15% higher average yields by weight (458 cwt/acre) than with preplant uncoated urea (400 cwt/acre), significant at the 10% level. High yields correlated positively with high soil nitrate levels, which were 72 ppm higher with SCU than with uncoated 6N-8P2O5-8K2O three months after fertilization. Combined average yields with coated or uncoated 6N-8P2O5-8K2O applied preplant were similar, however, to yields with uncoated 6N-8P2O5-8K2O applied in three split applications. Sulfur-coated fertilizers reduced pH from 5.81 to 5.26 within one month of fertilization, but had no lasting effect five months after fertilization.
In further research at Gainesville and Live Oak, preplant 6N-8P2O5-8K2O was broadcast and incorporated at 10, 30, 50, 70, and 90% of N rates 90 and 180 lb/acre (Fiskell et al. 1970; Locascio et al. 1970b). The remaining fertilizer was banded in two equal side-dressings, once at thinning and again at early runner stage, to evaluate sustained nutrient supply for a 100-day watermelon crop. Beds were spaced 9 feet on center in two-year experiments at Gainesville and Live Oak, including a wet year (1970) and a dry year (1969).
Early growth was limited by greater amounts of band-placed fertilizer and accelerated by greater amounts of broadcast-placed fertilizer. Researchers cited soil-soluble salt levels in the bed nine times higher with banded fertilizer treatments, which likely discouraged early growth. Several weeks after fertilization, soil-soluble salt levels and growth equilibrated, resulting in similar yields with both placement treatments with 90 lb/acre N. With N rates to 180 lb/acre, yields with broadcast-placed fertilizer were 20% higher than yields with the band placed fertilizer (406 versus 342 cwt/acre). Optimum yields were obtained with 180 lb/acre N, averaged across all placement methods in 1969, a dry season, and 1970, a wet season (300 and 450 cwt/acre, 100% RY, respectively). Researchers concluded that three equal 6N-8P2O5-8K2O fertilizer applications totaling 180 lb/acre N, the first application broadcast and incorporated, resulted in the best yields. During the first dry season, yields were unaffected by the percentage of preplant-applied fertilizer, while peak yields were obtained with 30% preplant fertilizer during the wet season.
The same N rates, 90 and 180 lb/acre, from a mixed 12N-16P2O5-16K2O fertilizer were evaluated for yield response in factorial experiments involving different row spacings, fertilizer application methods, and mulch (Brinen et al. 1979). Experiments were conducted in Leesburg and Gainesville on overhead-irrigated plots. Row spacing and mulch interacted in their effects on yield at Leesburg. Watermelon yields on mulched beds increased as in-row plant spacing decreased from 15 to 5 feet (260 to 420 cwt/acre, respectively). Without mulch, yields were highest with the ten-foot spacing (410 cwt/acre). Mulch and fertilizer rates significantly affected yields and mean fruit weights in the Gainesville spring 1977 trial. Yields, averaged using mulched and unmulched treatments, increased 14% as N rate increased from 90 to 180 lb/acre N (590 cwt/acre, 100% RY). Significant (5% level) yield increases occurred with mulch treatments, resulting in 10% more fruit production (580 cwt/acre) with mulched than with unmulched plants. Mean fruit weight was also significantly (1% level) greater, 25 compared to 24 lb, with mulched than unmulched watermelons, respectively.
Controlled-release (CR) sources of N and K were compared with soluble sources in a spring 1993 trial at Live Oak (Hochmuth and Hochmuth 1994). The IFAS recommended N rate of 150 lb/acre was applied as soluble N (NH4NO3 and KNO3), or 50% CR-N (polymer coated urea) with soluble K, or 50% CR-N and K (50:50 polymer-coated urea and KNO3). Fertilizers were either all broadcast/incorporated preplant or applied in three equal applications: preplant (broadcast), banded at four-leaf, and banded at early runner stage. Fields were mulched with clear plastic during fumigation; then the mulch was removed before 'Royal Sweet' watermelons were transplanted. Beds were spaced on 7.5-foot centers, and N rates were expressed on an 8-foot row spacing, the standardized bed spacing for watermelon (Hanlon and Hochmuth 1989; Hochmuth and Hanlon 2009). Sprinkler irrigation was used to keep soil moisture at -10 centibars, measured by a tensiometer with the ceramic tip placed 6-inches deep in the root zone.
Since N and K rates were combined, yield response to N rate alone could not be evaluated. Average marketable yields using two independent K rates, 100 and 150 lb/acre K2O, were 22% higher (340 cwt/acre) with CR 50:50 N and K sources than with soluble sources (278 cwt/acre) when both were applied preplant. Conversely, soluble sources resulted in yields 6% greater than CR 50:50 when both were split applied. Leaf N concentrations were above sufficiency levels at early-runner-stage sampling (Hochmuth et al. 1991; 2009).
Nitrogen Rates
Subsurface Irrigation
Two cultivars, 'Jack of Hearts' [seedless] and 'Sangria' [seeded], were evaluated for response to N rates of 130 and 320 lb/acre, at the UF/IFAS Gulf Coast Research and Education Center in Bradenton (Maynard 1995). Fertilizer was broadcast uniformly at 130-60-180 lb/acre N-P2O5-K2O and incorporated in beds spaced 9 feet apart. Additional N and K were banded for total N-P2O5-K2O treatments of: 320-60-180, 130-60-360, and 320-60-360 lb/acre, followed by mulching with black polyethylene. Average marketable yield responses with 130 and 320 lb/acre N were similar, resulting in 95 and 100% RY (655 cwt/acre), respectively. Leaf-tissue N concentrations were high (>3.0%) at both fruit set and first harvest for both cultivars. High yields and tissue N concentrations were obtained with 130 lb/acre N, near the current IFAS recommendation of 150 lb/acre N.
Drip Irrigation
Drip irrigation is a technology for delivering water to the crop, just as is sprinkler or subsurface irrigation, but with increased water application efficiencies (Smajstrla et al. 2002). We acknowledged potential variations in fertilization/water efficiencies among irrigation methods by presenting yield responses to fertilizer by irrigation method.
Drip irrigation began to be accepted by watermelon producers in the 1980s (Figure 3). Early fruit production with drip-irrigated watermelons on deep Apopka fine sands at Leesburg was twice the early yields of plants with sprinkler irrigation (111 and 50 cwt/acre, respectively); (Elmstrom et al. 1981). Early yield represented 40% of the total yield with drip-irrigated plants in Leesburg, as opposed to 17% of the total yield with sprinkler irrigation. At Gainesville, early watermelon yields were similar with both irrigation methods. Total yields at both locations were not affected by the method of irrigation. Soils used for the Gainesville experiment were finer textured and on a higher water table than soils at the Leesburg site. Variations in soil type and water table resulted in similar total yields on irrigated or unirrigated plots in Gainesville, but unirrigated Leesburg plants produced only 44% of the fruit produced by irrigated plants. Plant rows were spaced 10 feet apart at both locations.
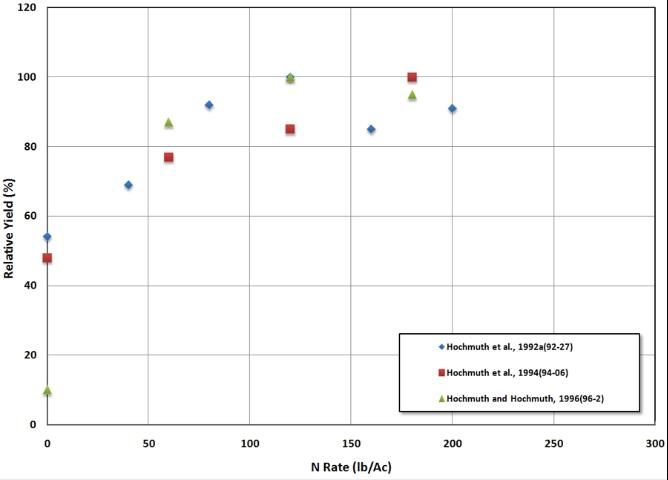
In a dry spring at Gainesville and Leesburg (spring 1981), water use was reduced 36 and 44%, respectively, with drip irrigation compared to overhead irrigation. Although total yields were not affected by irrigation method, the benefits of drip irrigation were numerous, including reduced water use, fewer weeds with restricted water application area versus general water application over cropped and noncropped areas with overhead irrigation, reduced plant stress periods due to equipment limitations with once-weekly overhead water applications, reduced occurrence of disease, and greater early melon yield on deep dry soils.
Fertilizer placement was an additional factor studied in this unmulched irrigation study (Elmstrom et al. 1981). Nutrients, 150-200-200 lb/acre N, P2O5, K2O, were applied one-third at preplant and two-thirds fertigated in both an overhead and drip-irrigated treatment. In a second overhead irrigated experiment, two-thirds of the fertilizer was applied to the soil preplant and one-third was applied at early runner stage. Total yields were similar regardless of the fertilizer split application and regardless of the irrigation method used. Another drip irrigation study in Live Oak by Hochmuth and Hochmuth (1992), showed that fertilizer placement did not have any effect on watermelon growth and yield. Several variations of banding and broadcast placements were evaluated.
Standard and icebox watermelons were grown on Lakeland fine sandy soils, spring 1988, at the UF/IFAS Suwannee Valley Agriculture REC near Live Oak (Hochmuth et al. 1992a). Beds on five-foot centers were used for the smaller icebox watermelons along with N rates of 0, 40, 80, and 120 lb/acre N (NH4NO3). The "standard" size 'Crimson Sweet' watermelons were grown on 7.5-foot centers with 0, 40, 80, 120, 160, and 200 lb/acre N from urea. Mehlich-1 soil tests revealed high P and low K soil indices. No P was applied, which was the IFAS recommendation with the soil testing in the high range, and 50 lb/acre K2O from potassium magnesium sulfate was applied, a rate 100 lb/acre of K2O lower than the current recommended rate for soils testing low in K. Fertilizers were hand-applied across the bed center, based on higher yielding results with this application method (Fiskel et al. 1967), as opposed to band fertilizer placement. Beds were then cultivated to incorporate the fertilizer and mulched with black polyethylene. Drip irrigation and tensiometers were used to maintain soil moisture at -10 centibars.
Yields of standard watermelon increased quadratically through 120 lb/acre N (380 cwt/acre, 100% RY). Early yields, with 120 lb/acre N, were 40% of the total yields. Researchers noted heavy reliance on drip irrigation during this extremely dry spring season. Yields of icebox watermelon did not change with N rates from 40 to 120 lb/acre N (392 cwt/acre with 40 lb/acre N). Researchers noted high yields with lower N rates for icebox watermelon but indicated additional trials were needed to confirm the optimum N rate for this type of watermelon.
Researchers noted a positive yield response with the highest N rate (180 lb/acre N) in another experiment at UF/IFAS Suwannee Valley Agriculture Research and Education Center, Live Oak (Hochmuth et al. 1994). Fertilizers were mixed, broadcast in a three-foot swath across the row, and incorporated at rates as follows: 0, 60, 120, and 180 lb/acre N from NH4NO3. Fertilizers were calculated based on an 8-foot row spacing, but the actual rows were spaced 7.5 feet apart. The crop was drip irrigated to maintain soil moisture at -10 centibars as measured by tensiometer. Beds were mulched with black polyethylene and 'Royal Sweet' watermelon transplants were set out on March 29, 1993. Marketable yields increased linearly between 0 and 180 lb/acre N, doubling from 265 to 555 cwt/acre (100% RY) with 65% of the total yield from the first two harvests. Average fruit weight also increased linearly from 11.9 to 18.0 lb with increasing N rates from 0 to 180 lb/acre. Researchers attributed part of the increased demand for N to a severe watermelon mosaic virus infection. Leaf N concentrations of plants with the check treatment were very high, 6.2 and 5.7% (>3.5% is adequate) at first flower and early fruit, respectively; this finding indicated abundant N available from mineralization early in the season from soils with 1.8% organic matter. Fresh petiole sap nitrate-N concentrations were below the adequate range only at the half-mature fruit stage. Apparently yield suffered from inadequate N late in the season.
Watermelon research continued the following spring in Live Oak with further monitoring of petiole sap N and K concentrations (Hochmuth and Hochmuth 1996). Fertilizers, including 120 lb/acre K2O from KCl and 0, 60, 120, or 180 lb/acre N from NH4NO3, were broadcast in a 30-inch swath and tilled. Beds were spaced 7.5 feet apart. Drip irrigation was used to keep the soil moisture in the root zone at -8 to -12 centibars in beds mulched with black polyethylene.
Total yield, early yield, and average fruit weight increased with N rate to 120 lb/acre N (433 cwt/acre, 100% RY). Early harvest accounted for 72% of the total yield, and average fruit weight was 18.0 lb. Yield was slightly reduced with 180 lb/acre N (410 cwt/acre, 95% RY), early yield was reduced to half of the total yield, and average fruit weight was reduced to 15.2 lb. Quadratic regression analysis established 130 lb/acre N as the optimum N rate. Petiole nitrate-N concentrations from plants fertilized with 120 lb/acre N were 2000 (high), 880 (low), and 720 (adequate) at early, midseason, and harvest sampling, respectively (Hochmuth 1994). Sap N on the first sampling (April 22) correlated best (r = 0.94) with total marketable yield. Whole-leaf tissue N concentrations were generally above sufficiency ranges.
Sprinkler Irrigation
Sprinkler irrigation was the accepted irrigation system in Central and Northern Florida, mostly movable guns and center pivots, and fertilizer research was conducted with sprinkler irrigation during the 1960s through the 1980s (Figure 4). Sulfur-coated urea and urea fertilizer sources, fertilizer placement methods, mulch types, and N rates (zero through 200 lb/acre) were evaluated in factorial experiments near Gainesville and Live Oak in 1971 through 1973 (Locascio et al. 1973). In all trials, plants received 170 lb/acre P2O5 and 150 lb/acre K2O in broadcast or banded applications, depending on the mulch treatment used. Broadcast fertilizer was spread throughout a 5-foot swath over beds spaced 9 feet apart and then tilled into the soil. Banded fertilizer was hand applied 3 inches deep to one side of the seed area, and split fertilizer applications were applied three times, first across the bed before planting, then in eight to 18 inch broad bands to either side of the plant row as the plants grew.
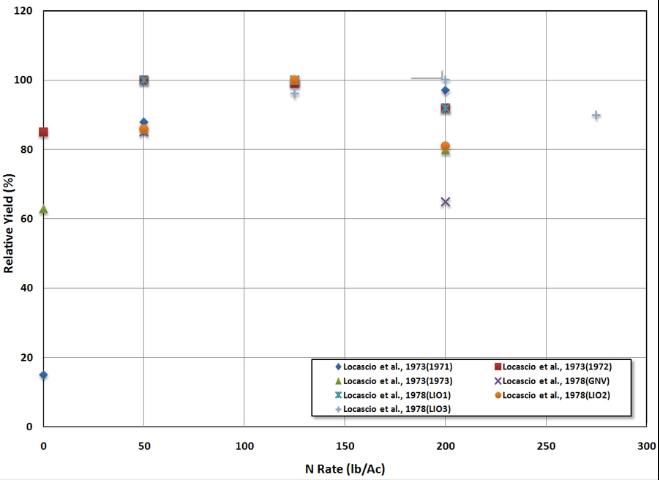
Yield responses to N rate, averaged for Gainesville and Live Oak sites in 1971, were quadratic for 1971 and also were quadratic during 1973 at Live Oak, resulting in highest yields with 125 lb/acre N (356 and 376 cwt/acre, respectively, 100% RY). With 200 lb/acre N, corresponding RYs were reduced at these locations to 97 and 80% RY, respectively (Locascio et al. 1973). Yields were not significantly different at Live Oak in 1972 with N rates from 0 to 200 lb/acre. Yield optimized this season with 50 lb/acre N (352 cwt/acre, 100% RY). Watermelon yields were always optimized with 125 lb/acre N. Heavy cover cropping before watermelon planting may have reduced the need for additional N in some of these experiments.
Nitrogen sources did not affect yields or tissue N concentrations at Gainesville and Live Oak in 1971 but were a factor in yield responses in Live Oak trials during 1972 and 1973. The highest yields in 1972 resulted from plants fertilized with urea banded with strip mulch (400 cwt/acre), followed by plants fertilized with broadcast sulfur-coated-urea (SCU)-30 (% dissolution in 7 days), urea broadcast in three applications, and SCU-44 (% dissolution in 7 days) (360 cwt/acre). Winds removed 50% of the strip and paper mulch in 1973, reducing yields with these mulched treatments. Equal yields of 380 cwt/acre were produced with broadcast isobutylidene diurea (IBDU), NH4NO3 broadcast in three applications, or urea broadcast under polyethylene mulch. A lower yield of 360 cwt/acre was produced with SCU-41 broadcast. Best N fertilizer sources overall were single broadcast applications of SCU-30 and 44 (% dissolution in 7 days), IBDU, urea under polyethylene, or three broadcast applications of urea or NH4NO3.
Sulfur-coated urea and uncoated urea were evaluated in studies at several sites in the early 1970s (Locascio et al. 1978). Yields with SCU were equal to urea in split applications except in one wet season. N rate and N source did not interact. Maximum yields were obtained with 125 lb N per acre.
Watermelon yield responses to N fertilizer for both drip and sprinkler irrigation are plotted in Figure 5. These data are from studies that made up the majority of studies with nitrogen. So that graphical presentation of yield responses might not be biased in any way by low-yielding studies, watermelon yield responses to N are plotted in Figure 6 for only those studies with a treatment yield greater than 330 cwt per acre, the state average yield for 2007–2008. Careful inspection of this figure shows that yield is most often maximized with 150 lb/acre N. There was only one study (Everett 1960) out of the 19 studies where more than 150 lb per acre N was needed.
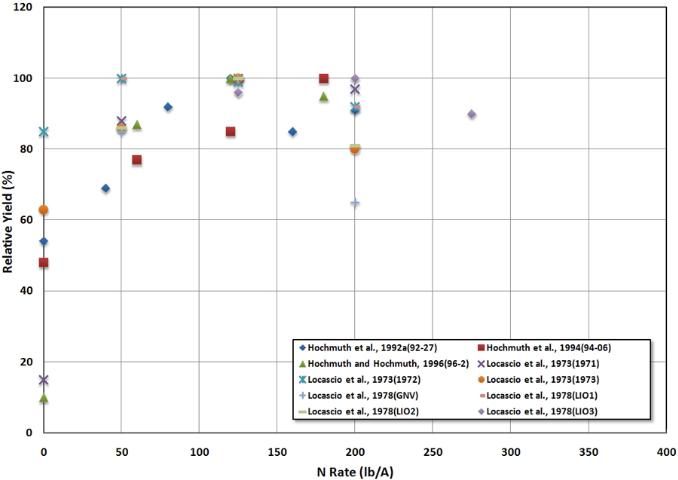
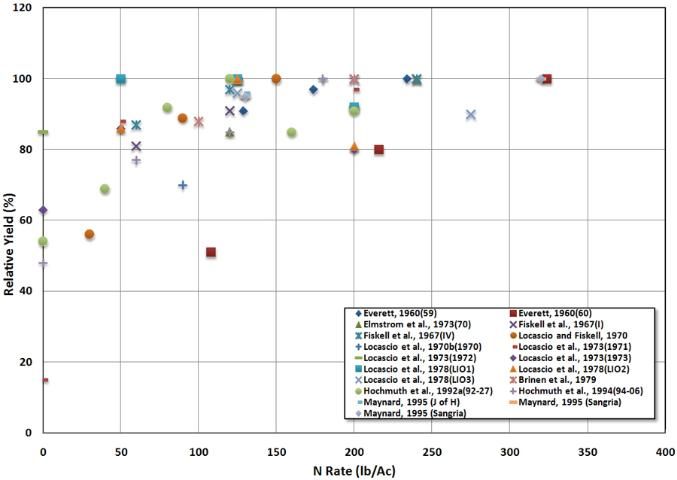
Nitrogen and Water
Management of nitrogen depends on careful attention to irrigation practices to minimize N leaching. Most of the studies summarized in this review focused on yield and fruit quality in response to fertilizer rate. More studies in the late 1990s began to study the impacts of fertilizer management and irrigation efficiency on nutrient movement and fertilizer use efficiency. A method was described by Simonne et al. 2003 for using a dye injected into the drip irrigation system to demonstrate water (and thus mobile nutrients such as nitrate-N) movement in the soil.
McNeal and colleagues conducted research on N and P losses from vegetable fields in Southwest Florida in the mid-1990s. The basis for the project was that nitrate-N was being found in drinking water wells in Central Florida counties (McNeal et al. 1994). These researchers noted differences in nitrate pulses in groundwater samplers between the deep sandy soils in the central ridge of the state where citrus is grown compared with the flatwood soils in Southwestern Florida where vegetables are grown (McNeal et al. 1995). The research showed rapid dissipation of nitrate pulses below the vegetable area compared with the citrus area. Denitrification was offered as a mechanism to explain the differences in nitrate dissipation. These authors offered several best management practices for vegetable and citrus producers.
The same research group studied phosphorus. Unlike nitrate pulses that dissipated, phosphate tended to persist in the high upper water tables in vegetable fields. Naturally occurring phosphate deposits appeared to possibly contribute phosphate to shallow water tables (Stanley et al. 1995). Understanding the local geology relating to phosphate deposits is important in locating water retention areas.
Nitrogen Summary
In 80% of the combined mixed fertilizer and N-only response trials, optimum yields (95 to 100% RY) were reached between 120 and 180 lb/acre N largely on overhead irrigated fields. In studies where only mixed fertilizers were used, best yields resulted with 120 through 240 lb/acre. In later studies that isolated yield response to only N, optimum yields were achieved with lower N rates, 120 to 130 lb/acre N. Yields were increased when fertilizer was broadcast/incorporated instead of banded on overhead irrigated fields and when cover crops were grown before planting. Yield response to higher N rates resulted when virus disease occurred on drip irrigated fields and during wet or dry seasons on subsurface irrigated fields. Drip irrigation often resulted in similar total yields as with overhead irrigation, but early yields were consistently higher (from 35 to 70% of total yields) when drip-irrigated trials were conducted on deep, dry sandy soils.
Research results with N fertilization support the current target N recommendation of 150 lb/acre for planting patterns using beds on 8-foot centers, using supplemental applications for leaching rains or extended harvesting seasons. Some growers have stated a concern that yields have increased over the years to the point where more N is needed than currently targeted by the recommendations. To test this idea, a separate graph was constructed (Figure 6) that presents yield responses to N rate for only those studies that had at least one fertilizer treatment that resulted in greater than 330 cwt/acre, the current average state yield. For several of these studies, yields were above 450 cwt/acre. In 18 out of 19 studies, yield was maximized with 150 lb per acre N or less.
The successful future of vegetable production depends on the improvement of fertilizer and irrigation efficiency (Hochmuth 1992; 2000). More recent research has been conducted in an effort to optimize fertigation management. A drip-irrigation study conducted by R. Hochmuth et al. (2005) on a number of farms operated by major vegetable growers in North Florida employed the use of a tracer dye, which was added to the irrigation water. This study showed the close link between fertigation and nutrient management. As a result of these experiments, farmers were able to reduce their water and N fertilizer use. More importantly, greater understanding was gained about the timing of application of fertilizers and the vulnerability of applied fertilizers to leaching into the environment. The results indicate that the practices of applying fertilizers preplant and very early during the planting season before the establishment of the root zone may need to be adjusted to reflect more sustainable agricultural practices.
Watermelon production practices were evaluated for yield and for nitrate leaching in a study in the mid-1990s (Simonne et al. 2002). Watermelon practices included N at the recommended rate and 1.5 times the recommended rate from all inorganic N (ammonium nitrate). A third treatment consisted of the recommended rate of N, one-half of which was supplied from poultry manure (with litter). Watermelon yields were not affected by N rate or program. Nitrate leaching was less with N at the recommended rate or with the program including the manure.
Phosphorus and Potassium Soil Testing
Mehlich-1 extractant indices (expressed as ppm soil-extracted nutrient) are classified as very low, low, medium, high, and very high; and a crop specific fertilizer recommendation is made from that classification (Hochmuth and Hanlon 1995a; 2000, Simonne and Hochmuth 2010). The M-1 solution became the accepted extractant standard in 1979 at the University of Florida. Before M-1, ammonium acetate and water extractants were used. Indices recorded from these methods cannot be directly equated with M-1 indices, but review of research results from studies with these extractants presents a profile of watermelon response with varying soil fertility conditions at that time. This review also summarizes practices in water management, fertilizer sources, fertilizer application methods, and the effect of mulch in the nutrient management system.
Phosphorus
Watermelon yield responses to P fertilization are presented in Figure 7. Acidic soils at Immokalee and Gainesville were limed to pHs ranging from 5.2 to 5.7 for factorial trials comparing P sources, P rates, and rates of Cu (Locascio et al. 1968). All soils were low in water extractable P. Phosphorus sources, superphosphate (SP), and concentrated superphosphate (CSP) were applied to virgin Immokalee fine sands in Immokalee. Diammonium phosphate (DAP), ammoniated superphosphate (ASP), and SP fertilizers were applied to Leon fine sands in Gainesville. In Immokalee trials (1965 and 1966), watermelons were fertilized with 120 lb/acre N and 240 K2O (5 hills, 3 x 10 feet apart). In both Gainesville trials (1966 and 1967), crops were fertilized with 130 lb/acre each N and K2O (8 hills, 5 x 9 feet apart). Fertilizers were applied in bands, once before seeding and again at early runner stage, for each trial. Supplemental N and K2O (15-0-14) were sidedressed as needed.
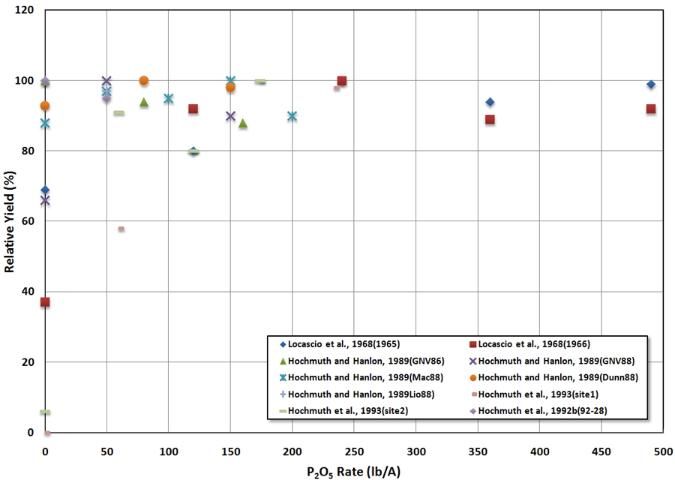
The type of irrigation applied was not specified in this study nor was use of mulch indicated. Based on the predominant form of irrigation used at each location at the time of the experiment, sprinkler irrigation was presumed with the Gainesville experiment, and subsurface irrigation was presumed with the Immokalee location. On the Immokalee fine sand soils, highest yields of 'Charleston Grey' watermelon were obtained with 240 lb/acre P2O5 from SP and CSP in 1965 and 1966 (464 and 442 cwt/acre, 100% RY) (Locascio et al. 1968). Yields with 120 lb/acre P2O5 were 80 and 92% RY each year (Figure 7). In 1966, the highest yields in Gainesville were with 160 lb/acre P2O5 from ASP (264 cwt/acre). In 1967, high yields in Gainesville again occurred with 160 lb/acre P2O5 (284 cwt/acre) averaged using ASP and SP treatments. Application of DAP in Gainesville (1966) reduced yields and raised soil pH from 5.5 to 6.1. Leaf tissue P concentrations averaged using three copper rates (0, 2, and 4 lb/acre) were deficient at early and first flower stages (< 0.25%) and adequate (0.25 to 0.5%) at harvest stage with all P rates from 0 to 480 lb/acre P2O5 in Immokalee. Increasing P rates decreased tissue copper concentrations at flowering. Tissue P concentrations at early-runner stage in Gainesville trials (1966 to 1967) were adequate (0.25 to 0.5%) for both seasons. Increasing P rates decreased tissue copper concentrations in both seasons.
Preplant fertilizer rate and placement were evaluated in a three-year study (1969–1971) at Leesburg (Elmstrom et al. 1973a). Two P rates were evaluated: 120 and 245 lb/acre P2O5 with 120 lb/acre N and K2O or 240 lb/acre N and K2O. These trials predated the M-1 soil test, but a "quite low" soil P evaluation (less than 5 lb/acre) was provided by an unspecified soil analysis method (ammonium acetate was in use at the time of this experiment). All of the P fertilizer and half of the N and K were either banded six inches below the planting row in a ten-inch band or broadcast in a 40-inch swath and incorporated. The remaining N and K were sidedressed at seedling emergence and before vines began to run. Treatments in 1971 included banding all of the P and broadcasting all of the N and K. The experimental plots were 20 x 24 feet and had two rows per plot. Mulch use and irrigation method were not specified. Band application of all applied P did not result in increased yields or higher tissue P concentrations in any year compared with broadcasting all of the P (Elmstrom et al. 1973a). Similar tissue P concentrations from band-applied treatments and from broadcast treatments indicated no variation in P availability between placement methods. High yields occurred with 120 lb/acre each N-P2O5-K2O applied in bands (290, 520, and 606 cwt/acre, 1969, 1970, and 1971, respectively, 100% RY). Yields were also optimized with 120 lb/acre N when the fertilizer was broadcast in 1969 and 1970 (274 and 576 cwt/acre, respectively, 100% RY) and resulted in 96% RY in the third season, 1971. Yields decreased 7 to 10% when 245 lb/acre P2O5 were banded with 120 lb/acre N and K2O. Tissue P concentrations increased 10 to 15% with banded P only when all of the N and K, instead of half, were broadcast and incorporated preplant at 240 lb/acre N and K2O (1971) (Elmstrom et al. 1973a). The authors cited increased growth stimulated by higher available N and K for the enhanced demand for and absorption of the banded P. Yields, however, were not affected by increased P absorption. IFAS recommends broadcasting and incorporating all P2O5 in the bed before planting, regardless of irrigation method (Hochmuth and Hanlon 1995; 2000).
Overhead Irrigation
Watermelon trials were conducted on unmulched fields in Gainesville in 1986 and on polyethylene mulched fields in Gainesville (research farm) and Dunnellon (commercial farm) in 1988 (Hanlon and Hochmuth 1992; Hochmuth and Hanlon 1988; Hochmuth et al. 1993a). Crops for P rate trials in Gainesville were direct-seeded while transplants were used in N and K factorial trials in Dunnellon. Fertilizers from NH4NO3, CSP, K2SO4, and KCl sources were mixed and BC/incorporated during bed formation at all sites. Nitrogen was added uniformly at 120 lb/acre on beds spaced 8 feet apart in Gainesville experiments and 12 feet apart in Dunnellon (fertilizer was calculated based on 8-foot centers for all locations).
Excellent yields, generally double the state average of 170 cwt/acre at the time of these studies, occurred at all sites with the recommended P rate based on preplant M-1 extractant soil tests. Gainesville soils tested high (46 ppm) and very high (120 ppm) for M-1 extracted P in 1986 and 1988, respectively, and no additional P was recommended. Watermelon yields did not respond to increased P applications from 0 to 155 lb/acre P2O5 in either year at Gainesville. Optimum yields occurred in 1986 on unmulched plots with zero lb/acre P2O5 (460 cwt/acre, 100% RY) and in 1988 on mulched plots with 50 lb/acre P2O5 (390 cwt/acre, 100% RY), though this yield was not different from yield with zero lb/acre P2O5. Researchers noted that soils in the beds were drier where overhead irrigation was applied to mulched fields, which likely resulted in lower overall yields compared to unmulched fields. Plants grown on mulched soils in Dunnellon 1988 produced optimum yields with 80 lb/acre P2O5 (320 cwt/acre, 100% RY) as per the recommendation for soils with medium M-1 P concentrations. However, yields at this site were not statistically different with P rates from 0 to 155 lb/acre P2O5. Tissue P concentrations at early fruit set were adequate for both Gainesville trials. Data for Dunnellon were not presented.
Drip Irrigation
Phosphorus and K fertilizers were broadcast and incorporated in Macclenny and Live Oak in1988 trials. Fertilizers mixed from NH4NO3 (120 lb/acre N), CSP, K2SO4, and KCl sources were applied uniformly to fields that were then mulched with black polyethylene (Hochmuth and Hanlon 1988; Hochmuth et al. 1993a). Yields were similar with all applied P rates to 200 lb/acre P2O5 at Macclenny and to 50 lb/acre P2O5 at Live Oak. High yield occurred with 150 lb/acre P2O5 (405 cwt/acre, 100% RY), the recommended rate for soils very low in M-1 soil-extracted P (6 ppm) at Macclenny (12-foot bed spacing). The Live Oak watermelon crop (7.5-foot bed spacing) resulted in high yields with zero lb/acre P2O5 (455 cwt/acre, 100% RY). No phosphorus is recommended for soils very high in M-1 extracted P (115 ppm). Phosphorus whole-leaf tissue concentrations at early fruit set were adequate (0.4 to 0.49%) with all P rates at both sites.
Watermelon response to P fertilizer was evaluated at two more Macclenny sites (1989). Both Ultisol soils were interpreted as very low in M-1 soil-extracted P, 5.6 and 10.2 ppm at sites one and two, respectively (Hochmuth et al. 1993b). Site one was newly cleared pine land, with pH 5.6, and site two was previously a bahiagrass pasture, pH 5.8. Soils were fertilized with 120 lb/acre N and K2O and experimental rates of P. A fertilizer mixture derived from NH4NO3, CSP, KCl, and potassium magnesium sulfate was hand spread, incorporated by rototiller, and mulched with black polyethylene. Rows were 9 feet on-center, and soil water potential was measured with tensiometers and maintained at -12 centibars.
Watermelon yield response was quadratic at both locations. Maximum (quadratic equation) yields at sites one and two occurred with 200 lb/acre P2O5 (397 and 656 cwt/acre, 100% RY, respectively). Yield responses, however, were not different at P rates between 50 and 200 lb/acre P2O5 (linear-plateau model). At early harvest, no fruits were harvested from plants at site one with the check treatment, and very few were taken from the check treatment at site two. Total marketable yields remained low, 1.5 and 36% RY, with each zero-P treatment. Leaf-tissue P concentrations reflected deficient and near deficient levels with check treatments at both sites (Hochmuth et al. 1993b). The authors suggested considering the midpoint between the shoulder value of the linear-plateau model and the maximum point with the quadratic model as the optimum P fertilization amount. This would result in a recommendation of about 80 lb per acre P2O5.
Watermelon ('Crimson Sweet') yield responses to P fertilization were measured at Live Oak on soil with a Mehlich-1 P index of 115 ppm (very high). Watermelon yield did not respond to P fertilizer (Hochmuth et al. 1992b). Yields were 547 cwt/acre (100% RY) with zero P. Watermelon whole-leaf P concentration was 0.47% when fruits were 2 inches in diameter (about 35 days from harvest).
Two pot-cultured watermelon crops in Quincy were designed to test P availability using various liming practices. The experiment included lime applied one month before or after application of DAP (fall 1992) and tested the availability to plants of residual soil P the following spring (Rhoads and Olson 1994). Pots contained 2 kg of Norfolk loamy sand (Ap horizon) with less than 5 ppm (very low) M-1 soil-extracted P. Fertilizer was applied per kg of soil at the following rates: 128 mg N from NH4NO3, 300 mg K2O from K2SO4, 90 or 180 mg P2O5 from CSP and DAP, and 1 g lime from CaO, which was applied either four weeks before or after P application (fall 1992). Nitrogen alone was applied for the spring planting. Soil water levels approached field capacity by addition of 100 mL tap water when the soil surface dried. A check treatment for each season omitted P and lime applications.
Phosphorus availability was 26% greater with CSP than DAP when lime was applied four weeks before P. Lower overall P availability occurred with both sources when lime was applied after P with 18% more available P from CSP than DAP in the first season. The highest P uptake value was taken as 100% for each season, and all other P uptake responses were calculated as a percent of that maximum. First-season P uptake was greater with CSP, 36%, than DAP, 19%, as P rate increased from 90 to 180 mg/kg, but P uptake equilibrated in the second season, (34% and 31% for CSP and DAP, respectively). On acidic soils, CSP was more effective as a P source, but either source performed well in residual tests on soils limed several weeks before soil sampling.
Phosphorus Summary
The maximum UF/IFAS recommended P rate of 150 lb/acre P2O5 for very low P was supported by a predominance of optimum yield responses at or below this rate. Standardized IFAS recommendations for P application were adjusted downward from the 1989 recommendation of 160 lb/acre P2O5 for soils very low in M-1 P to the 150 lb/acre P2O5 rate. Recommended rates for low and medium M-1 soil P concentrations were also adjusted downward from 160 and 100 lb/acre P2O5 to 120 and 80 lb/acre P2O5, respectively. Yield response differed from the recommended rate in Gainesville and Immokalee, where acidic soils low in water-extracted P increased the demand for this nutrient (Locascio et al. 1968). Yield response was similar with SP and CSP sources, though DAP used on acidic soils raised pH and reduced yields. A second-season planting responded equally to DAP or CSP when lime was applied in the previous planting season. Band or broadcast placement of P had little effect on watermelon yields or tissue P concentrations when half of the N and K fertilizers were broadcast preplant. Broadcasting all of the N and K preplant increased absorption of banded P in one study but did not increase yields.
Potassium
Overhead Irrigation
Watermelon yield responses to K fertilization are presented in Figure 8. Watermelon yield responses to K fertilizer at M-1-based recommendations were tested in Dunnellon 1988 (Hanlon and Hochmuth 1992; Hochmuth and Hanlon 1988; Hochmuth et al. 1993a). Watermelon crops were fertilized with 120 lb/acre N (NH4NO3), three rates of P2O5 (CSP), and five rates of K2O from a mixture of KCl and K2SO4. Mixed fertilizers were broadcast/incorporated in the bed area followed by mulching with black polyethylene and planting of 'Crimson Sweet' watermelon transplants. Beds were spaced 8 feet on center. Soils in Dunnellon tested very low (10 ppm) for M-1 soil-extracted K. Total yields increased from zero through the 150 lb/acre K2O recommended rate (91% RY) and peaked with 200 lb/acre K2O, (329 cwt/acre, 100% RY) exceeding the state average of 170 cwt/acre for 1988 (Figure 6).
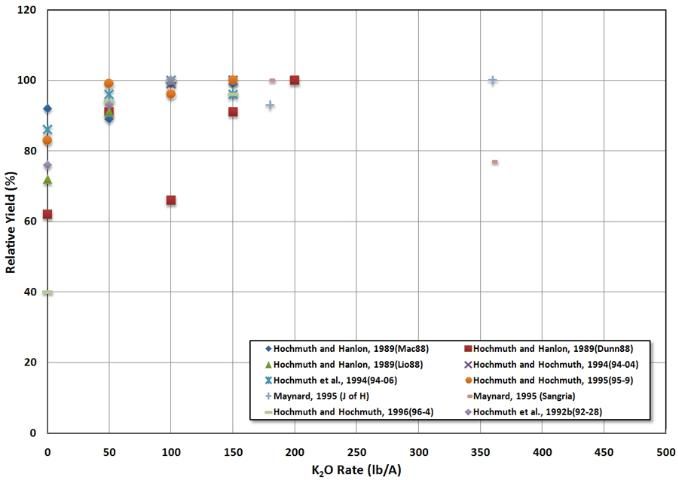
Yield benefits and reduced nutrient leaching from controlled-release (CR) fertilizer sources tested on other vegetables, such as potato and pepper, prompted a Live Oak watermelon trial with CR fertilizers in spring 1993 (Hochmuth and Hochmuth 1994). Yield responses were compared from the following fertilizer sources: all soluble N and K, half CR-N with soluble K, and half CR-N and K (50/50). Potassium was applied at 100 or 150 lb/acre K2O as one preplant application or three split applications. Preplant fertilizers, including 150 lb/acre N, were broadcast/incorporated, fields were mulched with clear polyethylene only during fumigation week (then removed), and 'Royal Sweet' watermelons were transplanted. Beds were spaced on 7.5-foot centers, and fertilizer rates were calculated based on an 8-foot bed center. Overhead irrigation was used to maintain soil moisture at -10 centibars.
Soil used for these studies tested medium (52 ppm) for M-1 soil-extracted K, and 80 lb/acre of K2O was recommended (Hochmuth and Hochmuth 1994). Yields averaged using CR treatments and two application methods responded similarly to 100 or 150 lb/acre K2O producing 99 and 100% RYs (322 cwt/acre), respectively. Preplant applications of CR (50/50) fertilizer increased yields 18% (340 cwt/acre) over preplant soluble sources (278 cwt/acre), but three split applications of CR (50/50) decreased yields 6% (329 cwt/acre) compared to split applications of soluble sources (350 cwt/acre). Comparable yields (340 and 350 cwt/acre) were obtained with soluble sources applied in split applications or with CR (50/50) fertilizers applied entirely preplant. Leaf tissue K concentrations at early runner-stage were adequate with all rates and placement schedules.
Subsurface Irrigation
Varying amounts of N and K fertilizer were evaluated for their effect on 'hollow-heart,' a condition where the rind grows faster than the inner tissue causing an internal cavity, incidence using two watermelon cultivars at the UF/IFAS Gulf Coast Research and Education Center, Bradenton, spring 1995 (Maynard 1995). Yield responses to 180 and 360 lb/acre K2O were similar. The effect of varying N and K rates on the incidence of hollow-heart was tested on cultivars chosen for their tendency to resist hollow-heart (Maynard 1995). Higher N and K2O rates decreased hollow-heart incidence 40 and 44% (130–320 lb/acre N and 180–360 lb/acre K2O, respectively) in 'Jack of Hearts,' which was prone to hollow-heart. The same fertilizer increases, however, had no effect on hollow-heart incidence in 'Sangria,' the watermelon with hollow-heart tolerance. Elsewhere, researchers observed that hollow-heart onset occurs more frequently in fruit set on the seventh to eighth node (crown-set fruit) than fruit set on the twentieth node (lateral-set fruit), that some cultivars are more susceptible than others, and that seedless varieties are more susceptible than seeded varieties (Kano 1993). The effects of fertilizer, water management, and environmental factors on the incidence of hollow-heart are as of yet unknown. Previous work with drip irrigation concluded that incidence of hollow-heart was not significantly affected by increasing irrigation water levels—4, 7, and 12 acre-inches applied seasonally by drip irrigation to five watermelon varieties (Clark and Maynard 1994; Maynard and Clark 1993).
Drip Irrigation
Varying K rates were broadcast and incorporated on fields in Macclenny and Live Oak in 1988 to evaluate yield response to M-1-based K recommendations (Hanlon and Hochmuth 1992; Hochmuth and Hanlon 1988; Hochmuth et al. 1993a). Soils were fertilized with 120 lb/acre N (NH4NO3), five P2O5 rates (CSP), and four K2O rates (mixture of KCl and K2SO4) before being mulched with black polyethylene.
Macclenny soils tested very low in M-1 soil-extracted K (6 ppm), and 150 lb/acre of K2O was recommended. Potassium rates were calculated on 8-foot bed centers for both locations with beds spaced on 12-foot centers at Macclenny and 7.5-foot centers at Live Oak. Maximum yield response occurred with 70 lb/acre K2O (411 cwt/acre, 100% RY) based on an 8-foot bed center. Early fruit set leaf K concentrations did not differ with increasing K rates. Tissue K concentrations averaged 2.0%, which was below the adequate range (2.3 to 3.5%), despite yields above the state average of 170 cwt/acre. Soils in Live Oak tested medium (38 ppm) in M-1 soil-extracted K, and 80 lb/acre of K2O was recommended. High yield occurred with 100 lb/acre K2O (512 cwt/acre, 100% RY), and lower yields resulted with 50 lb/acre K2O (91% RY). Leaf K concentrations increased linearly within the adequate range at Live Oak.
Watermelon ('Crimson Sweet') yield response to K fertilization was measured on a sandy soil with a Mehlich-1 soil test index of 38 ppm (Medium) for which 100 lb/acre of K2O was recommended (Hochmuth et al. 1992b). Early yield increased linearly in response to K from 0 to 100 lb/acre K2O per acre. Total yields did not respond to K2O (593 lb/acre with 100 lb K2O lb/acre, 100% RY). Whole-leaf K at 2-inch fruits increased from 2.1% with 0 K2O to 2.9% with 100 lb K2O lb/acre.
Soil from the upper six inches at the Suwannee Valley Agricultural Research and Education Center, Live Oak, was medium (45 ppm) for M-1 soil-extracted K, and 80 lb/acre of K2O was recommended (Hochmuth et al. 1994). Fertilizers were mixed, broadcast three feet across the row, and incorporated at experimental rates: 0, 50, 100, and 150 lb/acre K2O (KCl) and 0, 60, 120, and 180 lb/acre N (NH4NO3). Beds, spaced 7.5-feet on center, were mulched with black polyethylene and drip irrigated to maintain -10 centibar tensiometer readings. 'Royal Sweet' watermelon transplants were set out on March 29, 1993.
Early harvest yields peaked with 100 lb/acre K2O, (371 cwt/acre, 100% RY), but total season yields were not significantly affected by K rates from zero to 150 lb/acre K2O. Although yields were not greatly different, a slight total yield increase occurred with 100 lb/acre K2O (472 cwt/acre, 100% RY). Leaf tissue K concentrations were adequate through the first fruit stage with all K rates but deficient with all K rates two weeks before harvest. Yields higher than the state average of 230 cwt/acre did not appear affected by reduced leaf tissue K concentrations late in the season. Petiole K concentrations generally were 10 to 20% lower than the recommended range, though levels increased uniformly with increasing K rates until preharvest sampling. As with leaf tissue K concentrations, petiole sap K levels fell late in the season but only with the 0 and 50 lb/acre K2O rates.
Soluble N and K sources, NH4NO3 and KNO3, were supplemented with 0, 25, and 50% CR sources (polymer coated KNO3 and urea) at Suwannee Valley Research and Education Center near Live Oak in spring 1994 (Hochmuth and Hochmuth 1995). Beds were spaced on 7.5-foot centers, and fertilizers were calculated based on 8-foot bed centers. Nitrogen at 150 lb/acre was mixed with other fertilizers, broadcast 30 inches across the bed area, and rototilled. Fields were mulched with black polyethylene, and tensiometers were used to guide irrigation to maintain soil moisture at -8 to -10 centibars.
Potassium fertilizer from either all soluble or soluble plus 25 or 50% CR-K sources had no effect on total marketable watermelon yield or fruit size. Current M-1 interpretations recommend 120 lb/acre K2O for soils testing low (23 ppm) in K, but K2O applications of 50 lb/acre produced the same yields as those with the 150 lb/acre treatment. Yields averaged using all K sources for each rate were highest with 150 lb/acre K2O (452 cwt/acre, 100% RY) and slightly lower with 100 and zero lb/acre K2O (96% and 88% RY, respectively). Leaf K concentrations were deficient only with the check treatment at first flower, 2.5% (2.7 to 3.5 % is adequate), although yields with this treatment surpassed the state average of 230 cwt/acre with production of 378 cwt/acre of fruit.
Research continued in the spring of 1994 at the Suwannee Valley Research and Education Center with an evaluation of M-1-based K recommendations and calibration of petiole sap testing data (Hochmuth et al. 1996). Potassium from KCl was applied in rates of 0, 50, 100, and 150 lb/acre K2O with 150 lb/acre N from NH4NO3. Fertilizers were blended, broadcast 30 inches across the bed and rototilled. Beds were spaced on 7.5-foot centers with fertilizer rates calculated on an 8-foot bed spacing. Beds were mulched with black polyethylene and drip irrigated to maintain soil moisture tension at -8 to -10 centibars.
Based on a very low (18 ppm) M-1 soil-test result at this site, 150 lb/acre K2O was recommended. Total season marketable yield was maximized with 100 lb/acre K2O, (442 cwt/acre, 100% RY), in a quadratic response to applied K. With 150 lb/acre, yield was reduced to 96% RY. Petiole sap K concentrations, however, were near the recommended range only with 150 lb/acre K2O. Researchers concluded that current petiole sap sufficiency ranges were appropriate for samples taken early in the season but were high for samples taken late in the season. Whole leaf K concentrations were adequate with all low K rates until harvest when 150 lb/acre K2O was needed to ensure adequate K.
Watermelon yields responded positively to K rates 30 to 50% below the recommended rate or responded similarly to K rates above and below the recommended rate in the above drip-irrigated trials. Broadcast and incorporation of fertilizers preplant, as done in these studies, may have enhanced yield response. Banded-K fertilizers reduced yield response by 7 to 10% in Leesburg experiments (Elmstrom et al. 1973b). Soils analyzed for nutrient and soluble salt (SS) concentrations using saturated soil extract found soil SS concentrations increased 25% and 60% in 1969 and 1970, respectively with band versus broadcast treatments. Researchers cited early fertilizer burn and later K leaching losses, due to limited soil contact with the banded fertilizer, as factors in reduced yield response to banded fertilizers.
Blossom-End Rot
A significant inverse relationship occurred between the rate of applied K and Ca tissue concentration measured at early runner stage (Elmstrom et al. 1973b). Ca tissue concentrations decreased 10 to 25% with a K2O rate increase from 120 to 240 lb/acre (beds were 2-row plots 20 x 24 feet). The antagonistic effect of K on Ca absorption, however, did not increase the incidence of blossom-end rot (BER) in watermelon. BER was occasionally noticed on watermelon fruits, especially in previous years when elongated-shaped fruited varieties were grown, e. g., 'Charleston Grey.' Also, in years past, watermelons were often grown without irrigation, which increased BER. BER is rarely observed today in commercial watermelon production largely due to the less sensitive round or oblong fruited types and closer attention to irrigation. Conversely, when the incidence of blossom-end rot was high (45%), tissue Ca levels were also high (1.46%). Researchers suspect other nutrients or environmental factors increased blossom-end rot incidence. More information on BER in crops such as pepper can be found in Hochmuth and Hochmuth 2009.
Potassium Summary
Watermelon yields generally responded to K at or near the recommended K rate based on M-1 soil test analysis for each site. Potassium rates were adjusted upward from 1989 recommendations of 120 to 150 lb/acre K2O (1995) for very low K soils and from 100 to 120 lb/acre K2O (1995) for low K soils. Experiments that received drip-irrigation and broadcast-applied fertilizers generally responded well below the higher 1995 rate. When yields required more or less than the recommended K rate, dry soil conditions often increased demand for K, or broadcast/incorporated K fertilizer was more efficiently utilized by watermelon as with the above drip-irrigated studies. Preplant broadcast/incorporated application of 50/50, CR with soluble K sources, and overhead irrigation improved yields 18% compared to preplant soluble K. Soluble K sources resulted in the best yields when applied in three equal applications through the crop growth cycle. When soluble K was band-applied annually, soil soluble salt concentrations increased resulting in fertilizer burn of young watermelon plants. Variable yield responses to M-1-based K application reflect the mobility of this nutrient in sandy soils. Deeper soil sampling was suggested to assess K levels at depths easily reached by watermelon roots.
Overall Summary
Efficiency of watermelon production has increased during the past 50 to 60 years. Watermelons grown in Florida in 1960 from 73,000 harvested acres resulted in 115 cwt/acre. In 1995, average watermelon yields increased to 210 cwt/acre from 34,000 harvested acres, nearly twice the yield on half of the acreage. In 2007, the average yield was 320 cwt/acre. Yield increases likely resulted from a combination of improvements including factors such as new varieties, fertilizer placement, micronutrient use, lime applied according to recommendation, and coated fertilizers. Effective improvements in cultural practices were also reflected in these increased yields including reduction of weed competition with polyethylene mulch, pasture grass rotations to reduce nematode populations, effective herbicide use, drip irrigation, and fertigation. Studies are needed on optimal timing of N application with drip irrigation systems and on the fate of N in the soil system as it relates to N and irrigation management. Large-scale demonstrations on commercial farms of recommended nutrient management programs are also needed. Controlled-release fertilizers offer opportunities for increased nutrient efficiency for vegetables (Simonne and Hutchinson 2005). Additional research is needed with CR-N and K fertilizer sources to develop single application fertilizers effective in watermelon production. Limited research has been done with K fertilization of watermelon. Drip-irrigated trials dominate the research with yield responses to lower K rates than currently recommended. More work is needed tying together water and nutrient management practices with drip irrigation for best management practices (Dukes et al. 2006).
This publication summarizes the research to date on watermelon fertilization. The research to date provides support for the current fertilizer rates for fruit production; however, more work is needed to demonstrate the environmental impacts of fertilizer practices.
This literature review must not be interpreted as a recommendation—it is only a review and compilation of the research on fertilization. Reviews such as the present one may be used in the process of determining or revising recommendations. The selection of a fertilization recommendation addresses commercial yield and quality, the economics of crop production, and protection of the environment. Equally as important is to have a mechanism available to the grower to adjust production practices due to leaching rains and an extension of the growing season for additional harvests when economic conditions are favorable. To address all of these concerns, UF/IFAS vegetable recommendations are given as a single fertilizer rate and a series of footnotes that explain the addition of fertilizer during the growing season, including addressing leaching rains and additional harvests. This single initial fertilizer rate recommendation is by far superior to a range where most individuals would naturally select the highest value in the range. Therefore it is logical to select a single target rate that avoids unneeded fertilizer applications, often reducing crop nutrient efficiency and increasing the potential for environmental degradation. Thus, the current Florida approach is efficient and yet flexible at the same time.
Some BMPs supported by the research summarized above:
- Use soil testing with the Mehlich-1 soil test to determine P and K fertilizer needs.
- Research supports the current N rate of 150 lb per acre for watermelon grown on 8-foot beds.
- Any preplant fertilizer, especially under mulch, should be broadcast in the bed area and incorporated for sprinkler or drip-irrigated crops.
- Drip irrigation and fertilizer injection (fertigation) can be used to increase water and nutrient efficiency.
- There were no differences among N sources for effects on yields.
- Controlled-release N sources were effective in supplying N for watermelon production.
Literature Cited
Black, C. A. 1992. Soil fertility evaluation and control. Lewis Publishers, Boca Raton, FL. 746 pp.
Brinen, G. H., S. J. Locascio, and G. W. Elmstrom. 1979. Plant row spacing, mulch, and fertilizer rate effects on watermelon production. J. Amer. Soc. Hort. Sci. 104:724-6.
Brown, J. R. 1987. Soil testing: sampling, correlation, calibration, and interpretation. Soil Science Society of Amer. Special Publ. No. 21. Soil Science Society of America, Inc. Madison, Wisc.
Clark, G. A., and D. N. Maynard. 1994. The effects of drip irrigation rates on watermelon yields. Fla. Agr. Expt. Sta. Research Report: Gulf Coast REC Bradenton 1994-3 (March).
Dukes, M. D., L. Zotarelli, J. M. S. Scholberg, and R. Munoz-Carpena. 2006. Irrigation and nitrogen best management practices under drip-irrigated vegetable production. Procs. ASCE EWRI World Water and Environ. Resource Cong. P. 1-11.
Elmstrom, G. W., J. G. A. Fiskell, and F. G. Martin. 1973a. Watermelon yield and quality: effect of fertilizer rate and placement. Proc. Fla. State Hort. Soc. 86:196-200.
Elmstrom, G. W., J. G. A. Fiskell, and F. G. Martin. 1973b. Nutrient distribution in soil and watermelon plant uptake: effect of fertilizer timing, rate, and placement. Soil Crop Sci. Fla. Proc. 32: 154-158.
Elmstrom, G. W., S. J. Locascio, and J. M. Myers. 1981. Watermelon response to drip and sprinkler irrigation. Proc. Fla. State Hort. Soc. 94:161-163.
Everett, P. H. 1960. The influence of nitrogen source and fertilizer rate on watermelon yields. Proc. Fla. State Hort. Soc. 73:107-111.
Fiskell, J. G. A., S. J. Locascio, P. H. Everett, and H. W. Lundy. 1967. Effect of fertilizer placements and rates on watermelon yields. Proc. Fla. State Hort. Soc. 80:168-173.
Fiskell, J. G. A., S. J. Locascio, and F. G. Martin. 1970. Pattern of fertilization for watermelons: II. Influence on nutrient distribution in soil and plant uptake. Proc. Fla. State Hort. Soc. 83:149-153.
Florida Dept. of Agriculture and Consumer Services. 2005. Water quality/quantity best management practices for Florida vegetable and agronomic crops. http://www.floridaagwaterpolicy.com/PDF/Bmps/Bmp_VeggieAgroCrops2005.pdf.
Florida Dept. of Agriculture and Consumer Services. 2009. Florida Agricultural Statistics - http://www.florida-agriculture.com/fass_activities.htm.
Hanlon, E., and G. Hochmuth. 1989. Calculating fertilizer rates for vegetable crops grown in raised-bed cultural systems in Florida. Fla. Coop. Ext. Serv. Spec. Series SS-SOS-901.
Hanlon, E. A., and G. J. Hochmuth. 1992. Recent changes in phosphorus and potassium fertilizer recommendations for tomato, pepper, muskmelon, watermelon, and snapbean in Florida. Commun. Soil Sci. Plant Anal. 23(17-20):2651-65.
Hochmuth, G. J. 1988. Commercial Vegetable Fertilization guide. Fla. Coop. Ext. Serv. CIR 225C.
Hochmuth, G. 1992. Concepts and practices for improving nitrogen management for vegetables. HortTechnology. 2 (1): 121-125.
Hochmuth, G. J. 1994 (reviewed in 2009). Plant petiole sap-testing for vegetable crops. Fla. Coop. Ext. Serv. Circ. 1144. https://edis.ifas.ufl.edu/publication/cv004
Hochmuth, G. 1996a. Vegetable fertilization pp. 3-17. IN: G. Hochmuth and D. Maynard (eds.) Vegetable production guide for Florida. Fla. Coop. Ext. Serv. Circ. SP 170.
Hochmuth, G. J. 1996b. Commercial Vegetable Fertilization guide. Fla. Coop. Ext. Serv. CIR 225D.
Hochmuth, G. 2000. Management of nutrients in vegetable production systems in Florida. Soil and Crop Sci. Soc. Fla. Proc. 59:11-13.
Hochmuth, G., and K. Cordasco. 1999 (reviewed in 2008). Summary of N, P, and K, research on watermelon in Florida. Fla. Coop. Ext. Serv. Fact Sheet HS 755.
Hochmuth, G. J., and E. A. Hanlon. 1988. Mehlich-1 soil-test calibration for watermelons: phosphorus and potassium. Soil Crop Sci. Soc. Fla. Proc. 48:40-3.
Hochmuth, G. J., and E. A. Hanlon. 1989. Commercial vegetable crop nutrient requirements. Fla. Coop. Ext. Serv. Circular 806.
Hochmuth, G. J., and E. Hanlon. 1995a. IFAS standardized fertilization recommendations for vegetable crops. Fla. Coop. Ext. Serv. Circ. 1152.
Hochmuth, G. J., and E. A. Hanlon. 1995b. Commercial vegetable crop nutrient requirements in Florida. Fla. Coop. Ext. Serv. SP 177.
Hochmuth, G. J., and E. A. Hanlon. 1995c. IFAS standardized fertilization recommendations for vegetable crops. Fla. Coop. Ext. Serv. Circ. 1152.
Hochmuth, G. J., and E. A. Hanlon, 2000. IFAS standardized fertilization recommendations for vegetable crops. Circular 1152. University of Florida, Institute of Food and Agricultural Sciences, Gainesville, FL.
Hochmuth, G. J., and E. A. Hanlon. 2009. Calculating recommended fertilizer rates for vegetable crops grown in raised-bed mulched cultural systems. Fla. Coop. Extension Serv. Circ. SL 303. https://edis.ifas.ufl.edu/pdffiles/ss/ss51600.pdf.
Hochmuth, G. J., and E. A. Hanlon. 2010a. Commercial vegetable fertilization principles. Fla. Coop. Extension Serv. Cir. SL 319. https://edis.ifas.ufl.edu/pdffiles/cv/cv00900.pdf.
Hochmuth, G. J., and E. A. Hanlon. 2010b. Principles of sound fertilizer recommendations. Fla. Coop. Extension Serv. Cir. SL 315.
Hochmuth, G., E. Hanlon, and B. Hochmuth. 1992a. Field-testing N recommendations for icebox and standard watermelons in north central Florida. Fla. Agr. Expt. Sta. Research Report: Suwannee Valley REC 92-97.
Hochmuth, G. J., E. Hanlon, and R. Hochmuth. 1992b. Response of pepper, muskmelon, watermelon, and sweet corn to P and K fertilization at Live Oak, FL. Fla. Agr. Expt. Sta. Research Report: Suwannee Valley AREC 92-28.
Hochmuth, G., E. Hanlon, B. Hochmuth, G. Kidder, and D. Hensel. 1993a. Field fertility research with P and K for vegetables - interpretations and recommendations. Soil Crop Sci. Fla. Proc. 52:95-101.
Hochmuth, G. J., E. A. Hanlon, and J. Cornell. 1993b. Watermelon phosphorus requirements in soils with low Mehlich-I extractable phosphorus. HortScience 28:630-2.
Hochmuth, G., and B. Hochmuth. 1992. Placement of N-P-K fertilizer for mulched, drip-irrigated watermelons. Fla. Agr. Expt. Sta. Research Report: Suwannee Valley REC 92-20.
Hochmuth, G., and B. Hochmuth. 1994. Watermelon response to fertilization with controlled-release nitrogen and potassium fertilizers. Fla. Agr. Expt. Sta. Research Report: Suwannee Valley REC 94-04 .
Hochmuth, G., and R. Hochmuth. 1995. Effects of K rate and proportion of K supplied from controlled-release K on watermelon. Fla. Agr. Expt. Sta. Research Report: Suwannee Valley REC 95-9 .
Hochmuth, G. and R. Hochmuth. 1996. Responses of watermelon yield and whole leaf and petiole sap N seasonal profiles to N fertilization. Fla. Agr. Expt. Sta. Research Report: Suwannee Valley REC 96-2.
Hochmuth, G. J., and R. C. Hochmuth. 2009. Blossom-end rot in pepper: causes and prevention. Fla. Coop. Ext. Serv. Circ. SL284. https://edis.ifas.ufl.edu/pdffiles/ss/ss49700.pdf.
Hochmuth, G., B. Hochmuth, and C. Vann. 1994. Watermelon responses to N and K fertilization - yield, leaf, and petiole sap nutrient concentrations. Fla. Agr. Expt. Sta. Research Report: Suwannee Valley REC 94-06.
Hochmuth, G., R. Hochmuth, and C. Vann. 1996. Responses of watermelon yield and whole leaf and petiole sap K seasonal profiles to K fertilization. Fla. Agr. Expt. Sta. Research Report: Suwannee Valley REC 96-4 .
Hochmuth, G., D. Maynard, C. Vavrina, and E. Hanlon. 1991. Plant tissue analysis and interpretation for vegetable crops in Florida. Fla. Coop. Ext. Serv. SS-VEC-42. 62 pp.
Hochmuth, G., D. Maynard, C. Vavrina, E. Hanlon, and E. Simonne. 2009. Plant tissue analysis and interpretation for vegetable crops in Florida. Fla. Coop. Ext. Serv. Fact Sheet HS 964, https://edis.ifas.ufl.edu/ep081.
Hochmuth, R., E. H. Simonne, D. Studstill, J. Jones, C. Starling, J. Chandler and A. Tyree. 2005. On-farm demonstration of soil water movement in vegetables grown with plasticulture. Proc. Fla. State Hort. Soc. 118:184-186.
Kano, Y. 1993. Relationship between the occurrence of hollowing in watermelon and the size and number of fruit cells and intercellular air spaces. J. Japan. Soc. Hort. Sci. 62:103-112.
Kidder, G., E. A. Hanlon, and G. J. Hochmuth. 1989. IFAS standardized fertilization recommendations for vegetable crops. Fla. Coop. Ext. Serv. SS-SOS-907. Notes in Soil Science No. 38. July.
Locascio, S. J., P. H. Everett, and J. G. Fiskell. 1964. Copper as a factor in watermelon fertilization. Proc. Fla. State Hort. Soc. 77:190-194.
Locascio, S. J., J. G. A. Fiskell, P. H. Everett, and J. M. Crall. 1966. Watermelon response to copper and a complete micronutrient source. Proc. Fla. State Hort. Soc. 79:150-154.
Locascio, S. J. and J. G. Fiskell. 1966. Copper requirements of watermelons. Proc. Amer. Soc. Hort. Sci. 88:568-575.
Locascio, S. J., P. H. Everett, and J. G. A. Fiskell. 1968. Effects of phosphorus sources and copper rates on watermelons. Proc. Amer. Soc. Hort. Sci. 92:583-589.
Locascio, S. J., and J. G. A. Fiskell. 1970. Effects of sulfur-coated urea and potassium chloride on watermelon production and soluble salts. Soil Crop Sci. Soc. Fla. Proc. 30:113-122.
Locascio, S. J., J. G. A. Fiskell, and P. E. Everett. 1970a. Advances in watermelon fertility. Proc. Trop. Reg. Am. Soc. Hort. Sci. 14:223-231.
Locascio, S. J., J. G. A. Fiskell, and H. W. Lundy. 1970b. Pattern of fertilization for watermelons: I. Influence on plant growth and fruit yield. Proc. Fla. State Hort. Soc. 83:144-8.
Locascio, S. J., J. G. A. Fiskell, and H. W. Lundy. 1973. Watermelon response to sulfur-coated urea, mulches and nitrogen rates. Proc. Fla. State Hort. Soc. 86:201-4.
Locascio, S. J., J. G. A. Fiskell, and G. W. Elmstrom. 1978. Comparison of sulfur-coated and uncoated urea for watermelons. Soil Crop Sci. Soc. Fla. Proc. 37:197-200.
Maynard, D. N. 1992. Watermelon production guide for Florida. Fla. Coop. Ext. Serv. Spec. Publ. 113.
Maynard, D. N. 1995. Watermelon hollowheart spring 1995. Fla. Agr. Expt. Sta. Research Report: Gulf Coast REC Bradenton 1995-28 (October).
Maynard, D. N., and G. A. Clark. 1993. Drip irrigation rates affect watermelon yields. Fla. Agr. Expt. Sta. Research Report: Gulf Coast REC Bradenton 1993-4 (February).
McNeal, B. L., C. D. Stanley, L. A. Espinosa, and L. A. Schipper. 1994. Nitrogen management for vegetables and citrus: some environmental considerations. Soil and Crop Sci. Soc. Fla. Procs. 53:45-51.
McNeal, B. L., C. D. Stanley, W. D. Graham, P. R. Gilreath, D. Downey, and J. F. Creighton. 1995. Nutrient-loss trends for vegetable and citrus fields in west-central Florida. I: nitrate. J. Environ. Qual. 24 (1): 95-100.
Montelaro, J. 1978. Commercial vegetable fertilization guide. Fla. Coop. Ext. Serv. Circ. 225-B.
Mylavarapu, R. S. 2009. UF/IFAS extension soil testing laboratory (ESTL) analytical procedures and training manual. Fla. Coop. Ext. Serv. Circ 1248. https://edis.ifas.ufl.edu/ss312.
Nettles, V. F., and H. W. Lundy. 1958. Results of watermelon fertilizer trials at Gainesville and Live Oak in 1958. Proc. Fla. State Hort. Soc. 71:13-15.
Olson, S. M., and E. Simonne (eds.). 2010. The Vegetable Production Handbook. Fla. Coop. Extension Serv. https://edis.ifas.ufl.edu/collections/vph
Olson, S. M., E. H. Simonne, W. M. Stall, A. J. Gevens, S. E. Webb, T. G. Taylor, S. A. Smith, and J. H. Freeman. 2010. Cucurbit production in Florida. Chapter 9 IN: Vegetable Production for Florida. Fla. Coop. Extension Serv. https://edis.ifas.ufl.edu/pdffiles/cv/cv12300.pdf.
Rhoads, F. M., and S. M. Olson. 1994. Source of P, lime application sequence, and residual effects on P availability to watermelon. Soil Crop Sci. Soc. Fla. Proc. 53:10-13.
Schepers, J. S., K. D. Frank, and C. Bourg. 1986. Effect of yield goal and residual nitrogen considerations on nitrogen fertilizer recommendations for irrigated maize in Nebraska. J. Fertilizer Issues 3:133-139.
Simonne, E., M. Dukes, R. Hochmuth, and G. Hochmuth, D. Studstill, and W. Davis. 2002. Long-term effects of fertilization and irrigation recommendations on watermelon yield and soil-water nitrate levels in Florida's sandy soils. Acta. Hort. 627:181-186.
Simonne, E. H, and G. J. Hochmuth. 2010. Soil and fertilizer management for pepper production in Florida. Vegetable Production Handbook. Fla. Coop Extension Serv. https://edis.ifas.ufl.edu/pdffiles/cv/cv10100.pdf.
Simonne, E. H., and C. M. Hutchinson. 2005. Controlled-release fertilizers for vegetable production in the era of best management practices: Teaching new tricks to an old dog. HortTechnology 15:36-46.
Simonne, E. H., D. W. Studstill, R. C. Hochmuth, G. Mcavoy, M. D. Dukes, and S. M. Olson. 2003. Visualization of water movement in plastic-mulched beds with injections of dye with drip irrigation. Proc. Fla. State Hort. Soc. 116:88-91.
Smajstrla, A. G., B. J. Boman, G. A. Clark, D. Z. Haman, D. S. Harrison, F. T. Izuno, D. J. Pitts, and F. S. Zazueta. 2002. Efficiencies of Florida agricultural irrigation systems. Fla. Coop. Ext. Serv. Bull. 247. https://ufdc.ufl.edu//IR00004382/00001.
Stall, W. M., and R. K. Showalter. 1985. Watermelon production in Florida. Fla. Coop. Ext. Serv. Circ. 96G.
Stanley, C. D., B. L. McNeal, P. R. Gilreath, J. F. Creighton, W. D. Graham, and G. Alverio. 1995. Nutrient-loss trends for vegetable and citrus fields in west-central Florida: II. Phosphate. J. Environ. Qual. 24:101-106.