Introduction
This document describes the effects of water temperature on hard clam production in Florida. A glossary of terms is provided at the end of the document.
What is water temperature?
Temperature is the measurement of heat in a material and is related to the motion of the particles that make up the material. Many physical properties of materials depend on temperature, including phase (solid, liquid, or gas), density, and solubility. Temperature is one of the more important parameters collected with water-quality data because data such as conductivity, pH, and dissolved oxygen concentrations are dependent upon water temperatures.
Temperature also plays an important role in biology by determining the rate of biochemical reactions. Aquatic organisms have a range of water temperatures in which they function best. Outside this range, organisms do not function as well. Organisms also have upper and lower temperature tolerances that are incompatible with life.
How is water temperature measured?
Many methods have been developed for measuring temperature. Thermometers and thermistors are used most frequently to measure the temperature of liquids such as sea water.
Thermometer: Water temperature is easily measured using a thermometer. A thermometer contains a liquid that expands as its heat increases and contracts as its heat decreases. Therefore, the length of the liquid in the thermometer's tube varies with temperature. Temperature is determined by observing the length of the liquid and reading the calibrated scale printed on the side of the thermometer.
Maximum-minimum thermometer: One type of thermometer is the maximum and minimum (max-min) thermometer, which records the highest and lowest temperatures during a given time and is a simple method to determine the extremes of temperature at a given location. The thermometer consists of a U-shaped tube filled with mercury. One arm contains alcohol and records the minimum temperature; the other arm contains a vacuum and records the maximum temperature reached. As the mercury is pushed around the tube by the expansion or contraction of the alcohol, it pushes two small markers that record the furthest point reached by the mercury in each arm of the tube. The markers are reset by gravity or with a small magnet.
Thermistor: A thermistor is a temperature-sensitive electrical resistor; when water temperature changes, the resistance of the thermistor changes in a predictable way, allowing for temperature to be measured. Monitoring probes, installed at several lease areas in Florida, contain thermistors that measure water temperature (see https://shellfish.ifas.ufl.edu/water-quality-monitoring/).
Scales: Several temperature scales are in use. The Fahrenheit (°F) and Celsius (°C) scales are most frequently encountered. Throughout most of the world, and in the entire scientific world, the Celsius scale is used for measuring temperature. However, people in the United States are most familiar with and use the Fahrenheit scale. Celsius and Fahrenheit measurements can be converted using Equations 1 and 2.
Equation 1. Convert from Celsius (i.e., temperature measured in Celsius) to Fahrenheit

Credit: UF/IFAS
Equation 2. Convert from Fahrenheit (i.e., temperature measured in Fahrenheit) to Celsius

Credit: UF/IFAS
Why is water temperature variable?
Water temperature in coastal areas is regulated by many environmental variables including daily and seasonal meteorological cycles; water depth; amount of mixing due to wind, storms, and tides; and incoming water sources (e.g., precipitation, tributaries, artificial canals). Coastal water temperature fluctuates on a daily and seasonal basis. During daylight hours, energy from the sun warms the water, while heat is lost to the cooler atmosphere at night. In areas of Florida where hard clams are cultured, temperatures may fluctuate by more than 20°F (11°C) during a 24-hour period. Consider the following example from the Gulf Jackson High Density Lease Area located in the Gulf of Mexico at Levy County. On March 30, 2003, at 8:00 a.m. the water temperature was 72.3°F (22.4°C). At 7:30 a.m. the next day, the water temperature was 49.6°F (9.8°C), having fallen by 22.7°F (12.6°C) within a 24-hour period as a cold front moved through the area.
Seasonal water temperatures are also regulated by the amount of sunlight. Daylight hours are shorter and the sun is less intense (lower on the horizon) in the winter than in the summer, resulting in a net loss of energy to the atmosphere in the winter. Temperatures in shallow waters may fluctuate by more than 55°F (31°C) over the course of a year. For example, in 2003 at Gulf Jackson High Density Lease Area, temperatures reached a low of 39°F (4°C) in January and a high of 95°F (35°C) in July. For more examples of yearly, monthly, and daily water temperature fluctuation, see Figure 1, Figure 2, and Figure 3.
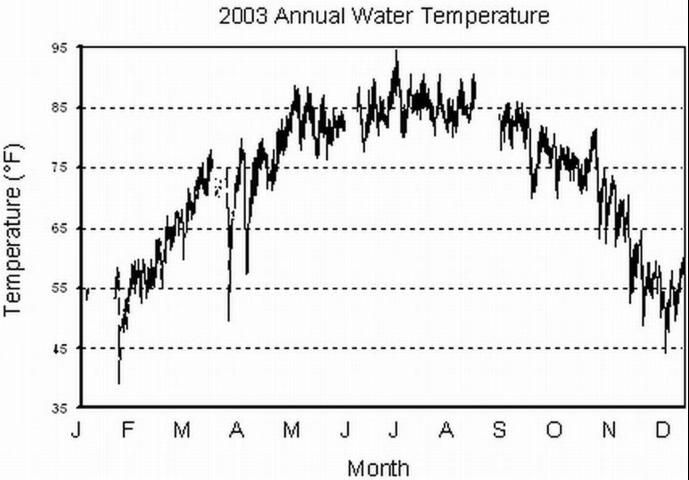
Credit: University of Florida (2003)
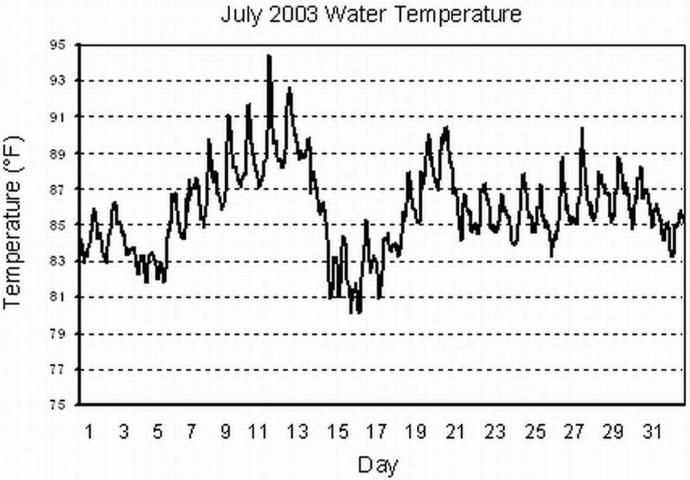
Credit: University of Florida (2003)
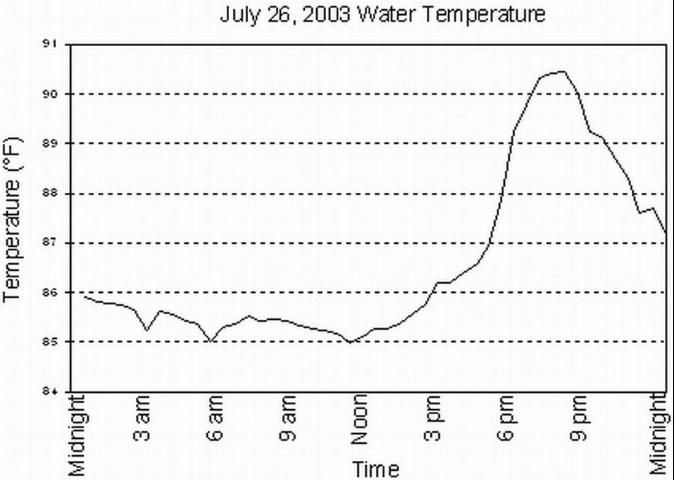
Credit: University of Florida (2003)
Water depth influences water temperature. In shallow bodies of water, energy from the sun is able to penetrate to the bottom and heat the entire water column; water in shallow tidal areas may reach temperatures near 100°F (38°C). Deep bodies of water may become stratified, with warmer, less dense water floating on top of colder, denser water near the bottom. At relatively shallow lease areas (< 6 feet at mean high water), such as the Gulf Jackson High Density Lease Area, there may be little difference in temperature between the top and bottom layers. "For example, in 2003, there was an average difference between surface and bottom temperatures of only 0.5°F (0.3°C) Shellfish Environmental Assessment Section, Florida Department of Agriculture and Consumer Services, Division of Aquaculture (personal communication). However, at deeper lease areas, such as the Sand Fly Key High Density Lease Area in Charlotte Harbor, growers report a difference of over 5°F (2.8°C) between the surface and bottom layers.
Wind, storms, and tides can have a significant impact on water temperature. Wind and storms primarily affect temperature by breaking up stratification, mixing the water, and equally distributing the heat throughout the water column. Tides also affect temperature; during high tides, cooler marine waters intrude into warmer coastal areas, the waters mix, and the temperature is lowered. The opposite happens during low tides; warm terrestrial waters (i.e., rivers and streams) flowing into estuaries have a greater influence than they do during high tide, causing the water temperature to increase. It should also be noted that tidally-induced temperature fluctuations may be greater during spring tides (new and full moons) than during neap tides (first and fourth quarter moons). In areas of Florida where hard clams are cultured, water temperature may vary by 5°F (2.8°C), or more, over a single tidal cycle. For example, at the Gulf Jackson High Density Lease Area in 2003, the temperature recorded at high tide (12:11 p.m., +3.5 feet) was 85.3°F (29.6°C), while the temperature recorded at low tide (7:44 p.m., +0.1 feet) was 90.1°F (32.3°C) (Figure 3).
The freezing point of seawater varies with salinity; seawater at 35 ppt freezes at 28.6°F (-1.9°C), while brackish water freezes at higher temperatures and freshwater freezes at 32.0°F (0.0°C). The estuarine waters of Florida rarely, if ever, freeze. However, clams may be exposed to freezing air temperatures if there is an extremely low or blowout tide during which the clams are not covered by water.
How does water temperature affect the physiology of hard clams?
Temperature plays an important role in biology by determining the rate of biochemical reactions; as temperature increases, biochemical reactions become faster. Metabolism is the biochemical breakdown of food to energy and is temperature dependent.
Like all other invertebrates, clams are cold-blooded organisms (poikilothermic); their body temperature fluctuates with that of the environment and their metabolism is directly influenced by water temperature. Increasing water temperature increases metabolic rate, while decreasing temperatures will decrease metabolic rate, affecting both growth and reproduction of clams. At the upper and lower extremes of temperature tolerance, these biochemical processes will cease, resulting in diminished growth, poor health, or death.
The limits of temperature tolerance are changeable. Frequently, the range of temperature tolerance is different in summer and in winter for the same species. An organism that is acclimated to winter temperatures may tolerate and be active at a temperature so low that it would kill an organism acclimated to summer temperatures. A winter-acclimated organism is less tolerant of high temperatures than a summer-acclimated organism.
Temperature also affects water quality. For example, the solubility of gases decreases with increasing temperature. Therefore, the amount of oxygen dissolved in water decreases by about half as the temperature is raised from 32°F (0°C) to 78°F (30°C). Because oxygen is a requirement for aerobic metabolism, at high temperatures it becomes a challenge for clams to obtain sufficient quantities.
What are signs of temperature stress?
Clams subject to temperature stress may exhibit valve, or shell, closure. Although clams can keep their valves closed for several days, they must obtain their energy through anaerobic metabolism. Clams may also exhibit shell gaping, especially following longer-term exposure to high temperatures. Signs of adverse environmental conditions in juvenile or adult hard clams may go unnoticed because they are infaunal, living buried in the sediment. However, stressed clams may rise to the surface of the sediment or fail to bury, which may indicate temperature stress or other adverse environmental conditions, such as suboptimal salinities.
How does water temperature affect hard clam production?
Hard clams inhabit coastal waters over a very wide geographic range, from Canada to Florida. This natural distribution is evidence of the adaptability of this species to a broad range of water temperatures, both as larvae and adults. Florida represents the southernmost limit of the hard clam, where subtropical temperatures allow for a long growing season. However, water temperatures in Florida may also exceed the optimum temperature range for hard clams during the summer months. A temperature range from 60 to 80°F (16–27°C) is considered optimal for hard clams. Over this range, pumping rates, feeding rates, growth, and other activities are at their maximum. Above and below this range, the clams will begin to show signs of stress. Growth ceases below 48°F (8°C) and above 88°F (31°C). Clams remain closed at temperatures below 37°F (3°C), and pumping rates decline sharply above 80°F (27°C), declining to zero at 90°F (32°C). It is difficult to determine an exact temperature that is lethal because duration of exposure is very important. A high temperature that can be tolerated for several hours may be lethal if continued for several days. As discussed below, other environmental conditions are important as well.
Our laboratory studies indicate at a salinity of 25 ppt, growout-size clam seed (10–15 mm shell length) and pasta-size clams (25–30 mm shell length) tolerate 90°F (32°C) for longer than 15 days, experiencing mortalities of only 1% and 4%, respectively. However, high temperature apparently increases the effects of salinity stress. At 10 ppt, pasta-size clams begin dying after four days of exposure to 90°F (32°C), with a total of 12% mortality by day 15, while growout-size clam seed begin dying by day 6 with a final mortality of 4.5%. At 40 ppt, both pasta-size clams and growout-size clam seed begin dying within the first day of exposure, with a total of 98% and 96% mortality by day 12 of exposure. These data are derived from laboratory experiments and should be viewed only as rough approximations of what may occur under more complex field conditions.
Other environmental conditions affect the ability of clams to survive adverse temperature conditions, including salinity and dissolved oxygen. For example, low salinity (<10 ppt), high salinity (>40 ppt), and low dissolved oxygen concentrations will intensify the effects of stressful temperatures. Furthermore, physiological conditions (e.g., energy stores and spawning stage), age, size, and acclimation history also determine the tolerance of a clam to temperature. The rate of temperature change is also important; clams will be more likely to show signs of stress if the temperature changes rapidly (i.e., hours to days), than if the temperature changes relatively slowly (i.e., days to weeks), allowing acclimation to occur.
Overview of Hard Clam Production
Hard clam production has three culture stages—production of small seed in a hatchery, growth of larger seed in a land-based nursery and/or field nursery, and growout to marketable size on an open water lease.
Hatchery—Clam culture begins in the hatchery with the production of seed. In the hatchery, adult clams are induced to spawn by altering the temperature of the water. Fertilized eggs and resulting free-swimming larval stages are reared under controlled conditions in large, cylindrical tanks filled with filtered, sterilized seawater. Larvae are fed cultured phytoplankton (microscopic marine algae) during a 10- to 14-day larval culture phase. After approximately 2 weeks, the larvae begin to settle out of the water column and metamorphose into juvenile clams. Even though a true shell is formed at this time, post-set seed are still microscopic and vulnerable to fluctuating environmental conditions. Thus, they are maintained in downwellers at the hatchery for another 30 to 60 days until they reach about 1 mm in size.
Nursery—The land-based nursery protects small seed until they are ready to be planted out onto the lease for growout. Nursery systems built on land usually consist of weller systems or raceways. Water, pumped from an adjacent saltwater source, provides naturally occurring phytoplankton and oxygen to the clam seed. Depending on water temperatures, 1–2 mm seed, obtained from the hatchery, require from 8 to 12 weeks to reach 5–6 mm in shell length, the minimum size planted in the field.
Growout—Clams are primarily grown on estuarine or coastal submerged lands leased from the State of Florida. Because clams are bottom-dwelling animals, growout systems are designed to place the clam seed on the bottom and provide protection from predators. Most clam growers in the state use a soft bag of polyester mesh material. The bag is staked to the bottom and naturally occurring sediments serve as the bottom substrate. Bag culture usually involves a 2-step process. The first step entails field nursing seed with shell lengths of 5–6 mm (1/4 inch) in a small-mesh bag. After about 3–6 months, the seed reach a growout size of 12–15 mm shell length (1/2 inch) and they are transferred to a bag of larger mesh size. A crop of littleneck clams (25-mm or 1-inch shell width) can be grown in 12–18 months.
How can I manage my crop in response to water temperature?
Consider Temperature Regime in Selecting a Lease Site
In the northeastern United States, the major temperature-related concerns for clam growers are cold water temperatures and ice. However, in Florida, we have few days in which the water temperature falls below 48°F (8°C), the temperature below which clam growth ceases. For example, in 2003 at the Gulf Jackson High Density Lease Area, only seven days had temperatures below 48°F (8°C). High temperatures, rather than low temperatures, are of greater concern in Florida. Again, taking Gulf Jackson High Density Lease Area in 2003 as an example, there were 30 days on which temperatures exceeded 88°F (31°C), the temperature above which clam growth ceases.
When considering a nursery or growout location, salinity regime should be the primary environmental factor in site selection. However, water temperature also plays an important role in the growth and survival of hard clams. Therefore, it is important to take temperature into account when selecting nursery and growout sites. In addition, two physical factors, depth and water flow, can either contribute to or offset temperature problems and should be considered in site selection. For example, shallow water (3 feet or less) will rapidly warm in the sun and may reach temperatures near 100°F (38°C) in the summer. Such shallow water depths may occur periodically at some sites during spring tides or other extremely low (blowout) tides. Growers might consider sites located in deeper water to avoid such extreme temperatures. On the other hand, deep sites may periodically experience stratification. Water, below the thermocline, may have too little oxygen or phytoplankton to support optimal clam growth.
Water currents should also be considered when selecting a site. High temperatures will be of greater concern in areas protected from currents by a landmass (for example, in the lee of an island), or that are stagnant; these areas are more likely to reach high temperatures on hot summer days. Water currents and tidal exchange allow for mixing and flushing of shallow warm water with cooler water and also help aerate the water, preventing hypoxia.
Understand the Temperature Regime at Your Site
To manage a clam crop proactively, it is important to understand the temperature regime at a given nursery or growout lease site. To better understand and respond to daily, seasonal, and annual variations in water temperature, growers should take frequent temperature measurements, as well as record their activities and subsequent crop performance.
A maximum and minimum (max-min) thermometer, which records the highest and lowest water temperatures reached during a given time period, is inexpensive and easy to use. A max-min thermometer should be placed near the bottom on the site where the clams are planted, not near the surface. Stratification of the water column can occur, resulting in warmer water on the top and cooler water on the bottom.
Taking temperature measurements over diurnal (daily) and tidal cycles will allow the grower to better understand the temperature fluctuations at a site. For example, temperature measurements taken in the summer months will help the grower determine how hot the water gets during a low tide that coincides with the heat of the day. Temperature measurements taken over a 24-hour period in the summer will allow the grower to determine when the coolest water temperatures occur and plan daily activities, such as harvest, accordingly.
Historical temperature records may also prove useful. Monthly water quality data can be obtained for shellfish harvesting areas in Florida by contacting a Shellfish Environmental Assessment Section (SEAS) field office of the Florida Department of Agriculture and Consumer Services, Division of Aquaculture (see https://www.fdacs.gov/Agriculture-Industry/Aquaculture/Shellfish-Harvesting-Area-Classification). Archived water quality data collected during 2002–2018 at selected aquaculture lease areas in 6 coastal counties can be found at http://shellfish.ifas.ufl.edu/water-quality-monitoring.
Nurse Clam Seed at Compatible Water Temperatures
Winter water temperatures in the Cedar Key area and panhandle of Florida become cold enough to reduce or stop the growth of seed clams. Therefore, land-based nurseries in these areas typically do not operate during the winter. However, land-based nurseries in southwest and east central Florida experience warmer winter water temperatures and nurse seed clams during the winter.
High summer temperatures are of primary concern, especially on the southwest coast and central east coast of Florida, where land-based nurseries typically close for the summer. In the Cedar Key area and panhandle of Florida, land-based nurseries can continue to nurse seed clams throughout the summer if maintenance is conducted daily. To prevent bacterial contamination, tanks or raceways should be rinsed daily with freshwater to control marine bacteria and prevent accumulation of sediment.
Conduct Farm Activities with Water Temperature in Mind
In the subtropical climate of Florida, seed clams can be purchased, planted, and transferred throughout the year. However, both water and air temperatures should be considered when scheduling these activities. In the winter, seed can be stressed or killed by exposure to cold air. Therefore, it is suggested that growers do not buy, plant, or transfer seed clams immediately before or during a winter cold front. Rather, growers should pay attention to local weather forecasts and schedule these activities after a cold front has passed, during warming trends. When transporting seed clams, contact with cold air can be minimized by covering the bags of clams with an insulating layer, such as empty growout bags or an old blanket.
Seed clams can be successfully purchased, planted, and transferred throughout the summer if extreme caution is taken in their handling. To minimize exposure to high air temperatures during transfer of growout-size seed to larger mesh bags, this activity could be conducted on a boat at the lease site, preferably under shade. If growout-size seed clams are transported to an upland facility to be sieved, transferred, and rebagged, these activities should be conducted in a shaded area and the growout bags should be transported back to the lease site immediately. Alternatively, the growout bags could be held overnight in an air-conditioned location, but care should be taken to prevent the clams from drying out or getting too cold. If a grower leases multiple sites or has a site that varies in depth, deeper areas that may not get as hot as shallower areas could be reserved for summer use.
When harvesting clams during the summer, growers must be aware of the effects of elevated temperature on product quality. When water and air temperatures are high, survival in refrigerated storage (shelf-life) decreases, and the maximum allowable hours from harvest to refrigeration (time-temperature matrix) is reduced in accordance with shellfish harvesting standards (Comprehensive Shellfish Control Code, Chapter 5L-1, Florida Administrative Code), to ensure product safety.
Both growers and shellfish wholesalers can minimize the effects of elevated temperature on product quality. First, growers can reduce stocking density of clams that are to be harvested in the summer. Reduced stocking density will decrease temperature stress by increasing the availability of food and oxygen to individual clams. Second, if growers examine the diurnal temperature cycle at their site, they will most likely note that both water and air temperatures are coolest in the early morning. It is therefore preferable to harvest in the early morning hours when temperatures are lower. Finally, growers must shade a product from the point of harvest until delivery to the wholesaler to keep the clams as cool as possible. Wholesalers are allowed to dry temper a product, a process by which clams are acclimated by a step-down process to the final storage temperature of 45°F (7°C) (see https://shellfish.ifas.ufl.edu/projects/shellfish-aquaculture-production-and-management/temperature-acclimation/). Dry tempering increases shelf-life during the summer months and minimizes microbial growth.
Summary
Water temperature in clam leases is an environmental factor that affects clam survival and growth. Because clam growers cannot control temperature on their leases, it should be a consideration for selecting sites and developing appropriate management strategies. The essential first step is temperature monitoring; with this information the clam grower can evaluate lease quality, determine optimal seed clam nursing periods, and plan daily farm activities. To minimize the potential economic impact to the industry, it is prudent to be aware of environmental conditions and to note any instances of mortality. Assistance from UF/IFAS Extension shellfish specialists is available.
Leslie Sturmer
UF/IFAS Extension Shellfish Agent
UF/IFAS Extension
Cedar Key, FL 32625
Phone: 352-543-5057
E-mail: LNST@ufl.edu
Shirley Baker
UF/IFAS School of Forest, Fisheries, and Geomatics Sciences, Program in Fisheries and Aquatic Sciences
7922 NW 71st St.
Gainesville, FL 32611
Phone: 352-273-3627
E-mail: sbaker25@ufl.edu
Glossary of Terms Used
Acclimation—The process of physiological adjustment to changes in conditions
Aeration—The process by which air is mixed with or dissolved into water
Aerobic metabolism—Cellular reactions requiring oxygen to produce energy from food molecules
Anaerobic metabolism—Cellular reactions producing energy from food molecules in the absence of oxygen. Anaerobic metabolism produces far less energy per food molecule than does aerobic metabolism
Biochemical reactions—Chemical reactions converting a substrate to an end product, aided by an enzyme, and forming the basis of metabolism
Blowout tide—An unusually low tide as a result of a low tide combined with a weather front, usually a cold front
Conductivity—The ability of a solution to carry an electrical current; often used to determine salinity
Diurnal—A daily cycle recurring every 24 hours; refers to the variation in temperature that occurs from the highs of the day to the lows of the night
Downweller—An open-ended cylinder in which clam seed are suspended on a screen and water flows down over the clams
Enzyme—A protein that catalyzes, or accelerates, biochemical reactions
Growout-size clam seed—Refers to clams greater than 10 mm in shell length that are grown on open-water leases in large mesh bags
Hypoxia—Reduced or inadequate concentration of dissolved oxygen in water
Infaunal—Aquatic organisms that live in the substrate, usually a soft sediment
Larva—Immature state of an organism that differs markedly in structure from the adult
Metabolism—The complete set of biochemical reactions that takes place in cells, allowing organisms to grow, reproduce, and respond to their environment
Metabolic rate—The rate at which food is converted to energy; the amount of energy expended in a given period; or the rate at which oxygen is used in aerobic metabolism
Metamorphosis—The marked and rapid transformation of a larva into an adult form
Neap tide—Tides that occur around the time of the first quarter and fourth quarter of the moon. At these points in the lunar cycle, the tide's range is minimum; high waters are lower than average, low waters are higher than average, slack water is present longer than average, and tidal currents are weaker than average
Phytoplankton—Freely floating microscopic aquatic plants (algae)
Poikilotherm—An organism whose body temperature varies with the temperature of the surrounding environment
Proteins—Complex molecules participating in every cellular process and having structural, mechanical, or enzymatic functions
Raceway—Shallow tank or tray with horizontal flow of seawater
Salinity—The concentration of salts dissolved in water
Seed—Refers to clams less than 10 mm in shell length
Shelf-life—Length of time that food remains suitable for sale or consumption; for clams, length of time shellstock remains alive in refrigerated storage
Signs—Objective evidences of disease
Solubility—The ability of a substance (e.g., salt) to dissolve into a solvent (e.g., water)
Spring tide—Tides that occur around the time of the new moon or full moon. At these points in the lunar cycle, the tide's range is maximum; high waters are higher than average, low waters are lower than average, slack water is shorter in duration than average, and tidal currents are stronger than average
Stratification—Cold (near the bottom) and warm (near the surface) waters form layers that act as barriers to mixing
Thermocline—An area of rapid change in temperature with depth
Tidal cycle—The cyclic rising and falling of the ocean surface, caused by tidal forces of the moon and sun acting on the oceans, and resulting in changes in depth and oscillating currents
Time-temperature matrix—Regulatory requirement for harvesting molluscan shellfish (clams) in which the maximum allowed time from harvest to refrigeration is based on month of the year (water temperature)
Upweller—An open-ended cylinder in which clam seed are suspended on a screen and water flows up between the clams
Weller system—Consists of open-ended cylinders suspended in a water reservoir or tank. Seawater circulates among the seed clams (either up or down), which are supported on a screen at the bottom of the cylinder
Further Reading
Goldburg, R. and G. H. Wikfors. 1991. "Growth of hard clams in Long Island Sound: sorting out the determining factors." Environ. Manag. 16: 521–529.
Kraeuter, J. N. and M. Castagna. 2001. Biology of the Hard Clam. Elsevier, Amsterdam, Netherlands. 751 pp.
Malouf, R. E. and V. M. Bricelj. 1989. "Comparative biology of clams: environmental tolerances, feeding, and growth." In: Clam Mariculture in North America. J. J. Manzi and M. Castagna, ed., 23–71. Elsevier, Amsterdam, Netherlands.
Pratt, D M. and D. A. Cambell. 1956. "Environmental factors affecting growth in Venus mercenaria." Limnol. Oceanogr. 1: 2–17.
Rice, M. A. 1992. The Northern Quahog: The Biology of (Mercenaria mercenaria). Rhode Island Sea Grant, Narragansett, RI. 60 pp.
Rice, M. A. and J. A. Pechenik. 1992. "A review of the factors influencing the growth of the northern quahog, Mercenaria mercenaria (Linnaeus, 1758)." J. Shellfish Res. 11: 279–287.
Roegner, G. C. and R. Mann. 1991. "Hard clam, Mercenaria mercenaria." In: Habitat Requirements for Chesapeake Bay Living Resources. 2nd ed. S. L. Funderburk, J. A. Mihursky, S. J. Jordan, and D. Riley ed., 5.1–5.17. Chesapeake Research Consortium, Solomons, MD.
Schmidt-Nielsen, K. 1997. Animal Physiology; Adaptation and Environment. 5th ed. Cambridge University Press, New York, NY. 612 pp.
Sturmer, L. N. 2004. Florida Shellfish Aquaculture Extension. http://shellfish.ifas.ufl.edu
Wells, H. W. 1957. "Abundance of the hard clam Mercenaria mercenaria in relation to environmental factors." Ecology 38: 123–128.