The majority of the foliage plants grown in Florida are subtropical and tropical in origin and are susceptible to damage at temperatures below about 50°F [10°C] (Conover and Poole 1984). Each year, winter temperatures are low enough in one or more of the major foliage plant-growing regions in Florida to cause chilling or cold injury. This publication is intended to inform ornamental foliage plant growers about various cold protection measures that can be adopted before and during cold events to avoid or minimize losses due to chilling or cold injury. A glossary containing definitions of selected terminology and a list of cited references for further reading are included at the end of this document. Words included in the glossary are italicized in the text.
Terminology used to describe cold weather in relation to plants (e.g., freeze, frost, and chill) is often confusing, and some of the terms are sometimes used interchangeably. For example, both frost and freeze are often defined similarly as an air temperature less than or equal to 32°F [0°C]. During a frost event, water within the plant may or may not freeze, depending on several factors (including concentration of ice-nucleating bacteria and the ability of plants to supercool). Freeze injury in plants mainly occurs due to extracellular (outside the cell) ice formation inside the plant tissues, which draws water out of the cells, dehydrating and causing injury to them. Either the entire plant or sometimes plant parts—such as buds, leaves, flowers, stems, or roots—are injured by cold temperatures.
Chilling injury is different from frost and freeze injury and is caused by tissue exposure to temperatures above the freezing point and below 46°F–54°F [8°C–12°C] (Lyons 1973; Sevillano et al. 2009). Chilling injury is primarily caused by disruption of cell membranes. Damage due to chilling temperatures differs with plant species and cultivars, temperature, exposure time, type of plant organ, and developmental stage. Plants can recover from damage if the exposure is very brief, but may have lingering damage that reduces growth. Irreversible damage occurs if the exposure time is prolonged.
In Florida, cold temperature damage to foliage plants is often due to chilling temperatures. The common symptoms of chilling injury are a) rapidly wilting leaves and appearance of water-soaked areas; b) appearance of sunken pits due to cell collapse; c) accelerated senescence (aging); d) increased decay due to leakage of plant metabolites, encouraging bacterial and fungal growth; e) reduced growth or sprouting capability; and f) browning of damaged areas (necrosis) due to warm temperatures following chilling temperatures.
Cold Protection
Nursery owners growing various tropical and subtropical plants under shadehouses can take several steps to protect plants from chilling injury. For convenience, the following cold protection strategies are classified into two categories: passive protection and active protection.
Passive Protection
Cold protection methods that are adopted before low temperatures occur are referred to as passive protection. These preventive methods act for long periods of time and become beneficial when chilling events occur. The following are the primary passive methods.
- Site selection and management of cold air drainage
- Plant selection and management
- Plant nutrition and pruning management
- Irrigation management
- Cryoprotectants
- Plant coverings
- Shadehouse cladding and heat curtains
- Weather forecasts and warnings
Site Selection and Management of Cold Air Drainage
Most growers are usually aware that some areas of their property are more prone to cold damage than others. Consulting with local growers and Extension advisors to find out about the appropriate plants and varieties to grow in an area should be the first step in site selection. Typically, low-lying and northwest locations in local topography have colder temperatures and, therefore, more damage. Cold air is denser than warm air, so it flows downhill and accumulates in low-lying spots, much like water. It is advisable to avoid cold spots unless adequate cost-effective cold protection methods are included in the long-term operation. In addition, segregating plants and cultivars according to their cold sensitivity and nursery site characteristics can help reduce damage from cold temperatures. Locating production areas on the south or southeast side of large bodies of water (lakes, reservoirs) can have a moderating effect on temperatures during advective freezes (Bill et al. 1979; Johnson 1963).
Managing cold air drainage is another part of site selection and management. It is important to study the flow of cold air downslope at night to prevent cold damage problems. A careful study of topography and/or the use of smoke bombs or other smoke-generating devices to study the cold air drainage can be informative. This information can be used to properly place diversion obstacles to provide protection from cold damage. Avoid building a shadehouse in low areas where cold air settles; instead, grow tender plants in sites with good air drainage that can provide a measure of protection. Land leveling can sometimes improve cold air drainage through nursery crops so that incoming cold air continues to pass through crops without settling. Covered structures (shadehouses and greenhouses) under which plants are grown need to be completely sealed to protect plants from cold winds during advective freezes. The number of entryways for regular cultural practices should be minimized during windy cold events, and the rest of the entries should be sealed with heavy-duty groundcover or other impermeable material. However, sealing a shadehouse can lead to colder temperatures occurring inside the structure than outside due to the lack of air mixing during radiation freezes and cold events (Stamps 1994). Thus, preventing air infiltration of shadehouses during cold events is a two-edged sword—beneficial under windy conditions, but counterproductive during calm, cool weather. However, if temperatures are expected to dip below the critical levels for the tropical foliage plants being grown, the structures must be closed up to retain heat.
Plant Selection and Management
Determining the planting dates that minimize potential for chilling temperatures is important. Shipping the most susceptible plants before the chilling event is a smart thing to do to avoid losses. If chilling temperatures cannot be avoided, then select cultivars that are tolerant of low temperatures. For some genera of foliage plants, research has demonstrated that there are significant differences in chill sensitivity among genera and cultivars (Table 1) (Castaldi 1990; Chen et al. 2001; Henley, Henny, and Chen 1998; Li et al. 2008)
Knowing the relative chill sensitivity of foliage plants can help in management decisions regarding which plants to group together and where to put them to minimize the need for heating and potential for chill damage to occur.
Plant Nutrition and Pruning Management
Unhealthy plants are more susceptible to cold damage and more often fail to recover from injury than plants grown with balanced nutrition. Although the relationship between specific nutrients and cold damage is not clear (Pellet and Carter 1981), in general, applications of nitrogen and phosphorous may stimulate new growth, thereby increasing crop susceptibility to cold damage. Avoid nitrogen application, which might promote soft new growth and interfere with plant hardening during later summer and early winter. Recent research on one kind of turfgrass suggests that supplemental silicon fertilization can enhance chilling resistance (He et al. 2009). Research about the effects of silicon on foliage plant chill sensitivity is needed.
Foliage plants may be pruned for a number of reasons—to shape them, to remove diseased or damaged branches, or to induce branching, for example. Research has shown that, in some cases and for some crops, pruning prior to the occurrence of cold temperatures can lead to increased cold injury (Androcioli and Caramori 2000; Warr et al. 2004). However, these effects have not been studied in foliage plants.
Irrigation Management
Soils and growing media serve as sinks for energy during the day and sources of heat at night. Heat is transferred through conduction. Dry soils and media have more air spaces, which limit heat retention and inhibit heat transfer (Rosenberg, Blad, and Verma 1983). In addition, wet soils and media are usually darker, resulting in increased absorption of solar radiation. Therefore, watering plants to field or container capacity before cold events can help protect the plants by increasing heat absorption and storage.
Cryoprotectants
Cryoprotectants have been evaluated for protection of plants or plant parts from freezing temperatures, but not the much higher chilling temperatures that can damage tropical foliage plants. Many cryoprotectant products are designed to prevent ice nucleation from occurring on the plant surface, or to promote supercooling. Both of these strategies are inappropriate for chill-sensitive tropical foliage plants. Furthermore, many of the products tested have been of little or no benefit, regardless of mode of action (Anderson and Whitworth 1993; Gardea et al. 1993; Goulart and Demchak 1994; Hare 1993; and Perry, Bonanno, and Monks 1992).
Plant Coverings
Covering plants with aqueous (water-based) foams may be effective for cold protection (Choi and Giacomelli 1999; Krasovitski et al. 1999); however, foams may not be suitable for use in shadehouses and on tall-growing crops, and they may not provide adequate protection for foliage plants. Foams serve as physical barriers to conductive, convective, and radiant heat transfer. Foams are relatively cheap, nontoxic, easy to use, and they break down easily when exposed to sunlight. However, disadvantages include the equipment and labor required for application and the need to reapply the materials each day.
Plants can also be protected, to a limited extent, using fabric crop covers (i.e., row covers and frost blankets). These needle-punched, woven, or spun-bonded covers are lightweight and porous and, as such, can be laid directly over the crop foliage while still allowing for overhead irrigation. Crop covers are made mainly of polyethylene, polyester, and polypropylene; however, other plastics and fabrics are also used. Covers are generally soft, nonabrasive, and come in a range of thicknesses and widths. In general, the thicker the cover, the greater the protection (Hochmuth et al. 1986; Stamps 1990). Again, the taller the crop, the less effective the covers if the only heat source is the soil and/or growing medium.
Shadehouse Cladding and Heat Curtains
Seasonally applied clear polyethylene film cladding on shadehouses is often used to trap heat, warm the soil, and increase chill protection. Unfortunately, polyethylene transmits infrared radiation (IR) readily, resulting in heat being lost to the outside (Nelson 1991). Films are available that have been designed with reduced IR transmission, but they are more expensive and may not be cost-effective for seasonal applications. Airtight cladding is critical for retaining heat in shadehouses.
Retractable heat (thermal) curtains (RHCs) can be used to reduce heat loss through the roofs of shadehouses and greenhouses (Figure 1) (Paris et al. 1985, Paris et al. 1988). This method is expensive initially, but it is less labor intensive than applying and removing seasonal shadehouse cladding every year. In addition to the labor savings, the annual costs of new polyethylene sheeting can be avoided by using RHCs. Polyethylene sheeting also has the potential to reduce plant growth because of shading caused by leaving the polyethylene in place all day during the winter, when radiation levels are already low. All these factors may make RHCs cost-effective in the long run.
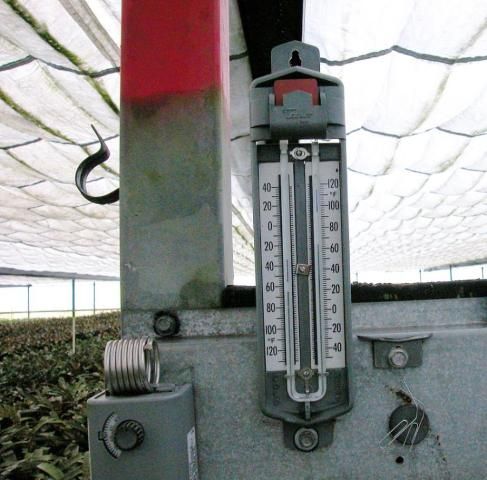
Credit: Robert H. Stamps
Weather Forecasts and Warnings
It is important that growers closely monitor weather forecasts. Previous years' weather data can be obtained from weather station websites (e.g., the Florida Automated Weather Network [FAWN], https://fawn.ifas.ufl.edu). Information can also be obtained by contacting your local UF/IFAS Extension agent. This information can be used to plan for current and future years' nursery operations. Understanding the local weather forecast allows time to react and take necessary precautions. Some losses can be prevented if growers align planting dates, cold protection measures, cultivation practices, and shipping dates based on weather forecasts and plant requirements.
Active Protection
Active protection includes strategies that are adopted during and after the freeze. These are temporary and are labor or energy intensive (sometimes both). Several of these strategies are combinations of both passive and active methods and may be adopted as needed. An accurate temperature monitoring system is a prerequisite for any active protection plan. Following are the primary active methods:
- Temperature monitoring
- Heating (geothermal or combustion)
- Combination methods
Temperature Monitoring
For accurate temperature measurements, the sensor must be accurate and must be placed in such a way as to provide true air temperatures (Hanan 1998). Temperature sensors range from simple, inexpensive, liquid-containing glass thermometers to more complex solid-state electronic devices, such as thermisters. Even a high-quality, liquid-containing glass thermometer—one with 0.2°F subdivisions—costs less than $30. For automated systems, thermostats that have sensors consisting of corrosion-resistant coils filled with liquids (or, in some cases, gases) are often used. The coil serves to increase surface area and improve (shorten) response times. Thermostats are typically located near the center of greenhouses and shadehouses and are shielded from direct sunlight. Electronic sensors are also commonly used, especially as components of automated climate control systems.
If temperatures in the greenhouse or shadehouse are not uniform during cold events, then readings should be taken in the spots that are typically coldest. In addition, measures should be taken to increase uniformity, or plants should be arranged in the structure to take those temperature differences into account. All readings, whether taken by thermometer or automated thermostat, should be taken at plant height to ensure measurement of the air temperatures that surround the plants. Aspirated thermometers and thermostats have fans that move air across the sensor and provide more accurate monitoring and faster response.
Heating
Any attempts to heat shadehouses and greenhouses effectively and economically require that they be sealed in some way to reduce air movement and heat loss (see "Shadehouse Cladding and Heat Curtains" section).
Following are the primary active methods:
- Geothermal heating
- Combustion heating
Besides sealing the shadehouses, heat must be released inside the structures in adequate amounts, based on the volume of the structures, to maintain the target temperatures. Two primary sources of energy are generally used to supply heat inside shadehouses and greenhouses in Florida—geothermal and combustion. There are advantages and disadvantages to using either source.
Geothermal Heating
Water, primarily from underground wells, is the most common "low-temperature geothermal" heat source. Surface waters, which are also heated by the earth, can also be used; however, during cold weather, surface waters are typically colder than groundwater. As water cools at temperatures above freezing, sensible heat is released. For example, as 72°F well water cools to 32°F, it gives off 83,664 calories of heat per gallon of water. Besides supplying heat inside shadehouses, raising the relative humidity to saturation inside a shadehouse can lead to the formation of fog. Fog can prevent transmission of infrared radiation from the crop, thereby reducing heat loss (Mee and Bartholic 1979).
The advantages of using water for cold protection instead of heaters are that a) equipment and installation costs are generally less (conventional irrigation systems could even be used, but they are not generally recommended except in emergencies), b) operational costs are greatly reduced since the only fuel needed is that used to run the water supply pumps (Castaldi 1990), c) maintenance costs are low since the equipment components do not wear out quickly and are inexpensive to replace, and d) less air pollution is created due to lower fossil fuel consumption.
Disadvantages of using water for heating are that a) leaching of nutrients and pesticides can occur if the water flows through the growing medium, b) reduced crop root-zone aeration and foliar wetting could lead to increases in leaf and root diseases, c) the shadehouse and surrounding areas could become flooded, and d) water, a limited resource, is removed from the aquifer if well water is used. However, the use of various low-pressure mist and fog systems that use significantly less water and direct it differently than regular irrigation systems can greatly reduce and/or eliminate some of the listed disadvantages. The following are examples of some systems designed to minimize some of the disadvantages of using water for heating shadehouses.
Over-the-Roadways Mist Systems. Water is applied using mist nozzles installed over the roadways so that the water neither leaches through the plant beds nor directly wets the crop foliage (Figure 2). This increases the moisture and heat content of the air and results in the formation of fog inside the shadehouse. In one shadehouse where an over-the-roadways mist system was used, water consumption was reduced by more than 50% compared to using the conventional over-the-bench mist irrigation system.
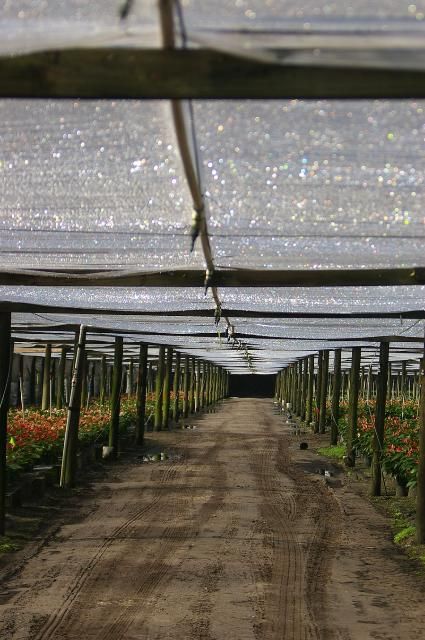
Credit: Robert H. Stamps
Under-the-Bench Mist Systems. In shadehouses where the plants are growing on open benches, heating water can often be applied using mist nozzles installed under the benches. This type of installation eliminates leaching and waterlogging of the growing medium and allows the heat to rise through the crop. Fog is produced as the air becomes saturated, the same as with the other systems. Under-the-bench systems may or may not save water, depending on the operating pressure, orifice size, and number of mist nozzles that are used.
Among-the-Plants Fog Systems: With these relatively new systems, the water is applied using low-pressure fog nozzles arranged among the plants (Figure 3). Fog quickly fills the shadehouse and creates a radiant heat loss barrier. These systems have been shown to greatly reduce water requirements, compared even to the mist-based cold protection systems. It has been determined that a low-pressure fog system uses 86% less water compared to the over-the-roadway mist system mentioned above (Figure 4) (Stamps et al. 2010).
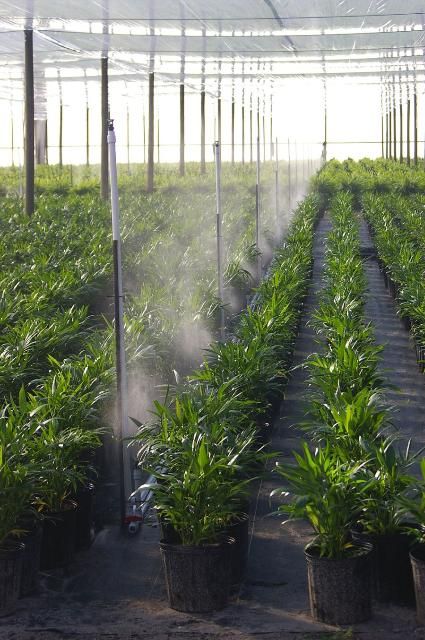
Credit: Robert H. Stamps
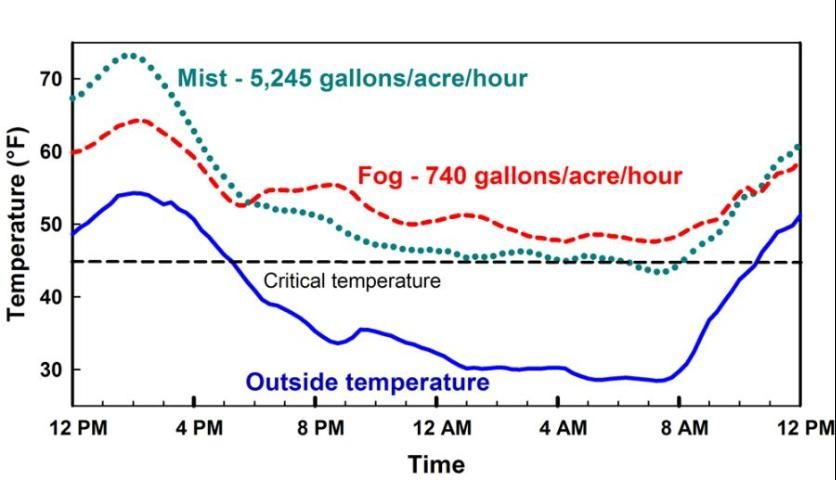
Combustion Heating
Various kinds of heaters—burning solid, liquid, or gaseous fuels—can be used to provide supplemental heat to shadehouses to help replace energy losses during cold events. Fossil fuels, such as liquefied petroleum gas, natural gas, and fuel oils, are the most often used source of energy; however, logs, lumber, and other nonfossil fuel sources can also be used in the appropriate heaters. Temperatures inside shadehouses will not drop to damaging levels if sufficient heat is generated inside the shadehouses to replace the energy lost. For best results, shadehouses should be leak proof.
Some advantages of using combustion heaters are that a) water is not removed from the aquifer; b) humidity in the shadehouse can be kept lower than when using water for heat, thereby helping to reduce the chances of foliar disease development; c) it does not impact the growing media; and d) large differentials between outside and inside temperatures can be maintained given adequate numbers, sizes, and types of heaters. A big disadvantage of using combustion compared to using geothermal energy sources is the much higher capital, operating, and maintenance costs (Castaldi 1990). Additional disadvantages of combustion heating include a) the release of air pollutants, some of which can adversely affect tropical foliage plants inside the shadehouse if the heaters are not properly vented (Waters and Conover 1981); b) the potential for fuel spills or releases that could cause environmental contamination; and c) the use of a limited resource. For detailed information about the selection, installation, and use of combustion heaters in greenhouses or enclosed shadehouses, see Hanan 1998 and Nelson 1991.
Combination Methods
Crop Covers and Irrigation. Research has shown that the combination of crop covers and over-the-crop water applications can maintain warmer temperatures under the covers than when covers are used alone (Stamps 1991). Whether or not this combination would be effective and economically feasible for use to protect tropical foliage plants from cold is unknown.
Under-the-Bench Mist System and Retractable Heat Curtains: Recent studies (Stamps et al. unpublished data) showed that a combination of an under-the-bench mist system combined with the use of retractable heat curtains maintained about a 30°F (17°C) temperature differential between inside and outside the shadehouse during an advective freeze. As mentioned previously, this combination would minimize the seasonal use of polyethylene film cladding.
Glossary
Advective freeze. A weather event in which temperatures drop to or below the freezing point (32°F [0°C]) because of large-scale mass movements of cold air (windy conditions).
Cladding. A covering over the outside of a building (e.g., polycarbonate panels on a greenhouse and polyethylene sheeting on shadehouses).
Combustion. Exothermic (process of releasing energy in the form of heat, light, electricity, or sound) chemical reaction between a fuel and an oxidant (e.g., oxygen).
Conduction. The flow of heat through a material or from one body to a cooler body in contact with it.
Convective. Heat transfer caused by the circulation of gases or liquids.
Crop cover. A cold protection device that is draped over a crop to trap heat from the soil.
Cryoprotectant. Any agent added to living tissue that reduces susceptibility to cold injury.
Fossil fuel. A fuel formed from the remains of organic materials and largely comprised of carbon and hydrogen. Coal, oil, and natural gas are all fossil fuels.
Geothermal. Pertaining to the heat of the earth.
Radiation. Energy transferred through space as electromagnetic waves.
Radiation freeze. A weather event in which temperatures drop to or below the freezing point (32°F [0°C]) under calm conditions.
Sensible heat. Heat absorbed or transmitted when the temperature of a substance changes, but the substance does not change state.
Supercooling. Process whereby the fluids in plants can remain liquid at temperatures below freezing, partly due to the presence of antifreeze proteins that prevent ice nucleation.
Literature Cited
Anderson, J. A., and J. Whitworth. 1993. "Supercooling strawberry plants inoculated with ice-nucleation active bacteria and treated with Frostgard." HortScience 28:828–830.
Androcioli, A., and P. H. Caramori. 2000. "Influence of coffee pruning on the severity of frost damage. Brazilian Arch. of Biol. and Technol." 43:41–44.
Bill, R. G., E. Chen, R. A. Sutherland, and J. F. Bartholic. 1979. "Simulating the moderating effect of a lake on downwind temperatures." Boundary-Layer Meteorol. 16:23–33.
Castaldi, M. 1990. "Frost protection economics." Amer. Fruit Grower 110:6–7.
Chen, J., R. W. Henley, R. J. Henny, R. D. Caldwell, and C. A. Robinson. 2001. "Aglaonema cultivars differ in resistance to chilling temperatures." J. Environ. Hort. 19:198–202.
Choi, C. Y., and G. Giacomelli. 1999. "Freeze and frost protection with aqueous foam-field experiments." HortTechnology 9:662–667.
Conover, C. A., and R. T. Poole. 1984. "Light and fertilizer recommendations for production of acclimatized potted foliage plants." Foliage Digest VII(8): 1–6.
Gardea, A. A., P. B. Lombard, C. H. Crisosto, L. W. Moore, L. H. Fuchigami, and L. V. Gusta. 1993. "Evaluation of Frostgard as an antifreeze, inhibitor of ice nucleators, and cryoprotectant on pinot-noir leaf tissue." Amer. J. of Enolo. and Viticult. 44:232–235.
Goulart, B., and K. Demchak. 1994. "Cryoprotectants prove ineffective for frost protection on strawberries." J. of Small Fruit & Viticult. 2:45–51.
Hanan, J. J. 1998. Greenhouses: Advanced technology for protected horticulture. Boca Raton, FL: CRC Press.
Hare, M. D. 1993. "Effects of frost and frost protectants on tall fescue seed production." New Zealand J. of Agricultural Res. 36:399–405.
He, Y., H. Xiao, H. Wang, Y. Chen, and M. Yu. 2009. "Effect of silicon on chilling-induced changes of solutes, antioxidants, and membrane stability in seashore paspalum turfgrass." Acta Physiol. Planta. 32:487–494.
Henley, R. W., R. Henny, and J. Chen. 1998. "Chilling injury on twenty Aglaonema cultivars." Southern Nursery Assoc. Res. Conf. Proc. 43:117–121.
Hochmuth, G. J., S. R. Kostewicz, S. J. Locascio, E. E. Albreghts, C. M. Howard, and C. D. Stanley. 1986. "Freeze protection of strawberries with floating row covers." Proc. Fla. State Hort. Soc. 99:307–311.
Johnson, W. O. 1963. "The meteorological aspects of the big freeze of December 1962." Proc. Fla. State Hort. Soc. 76:66–69.
Krasovitski, B., E. Kimmel, M. Rozenfeld, and I. Amir. 1999. "Aqueous foams for frost protection of plants: Stability and protective properties." J. of Agricultural Eng. Res. 72:177–185.
Li, Q., J. Chen, R. H. Stamps, and L. R. Parsons. 2008. "Variation in chilling sensitivity among eight Dieffenbachia cultivars." HortScience 43:1742–1745.
Lyons, J. M. 1973. "Chilling injury in plants." Annu. Rev. of Plant Physiol. and Plant Mol. Biol. 24:445–466.
Mee, T. R., and J. F. Bartholic. 1979. "Man-made fog." In Modification of the aerial environment of plants, eds. B. J. Barfield and J. F. Gerber, 334–352. St. Joseph, MI: Amer. Soc. of Agr. Eng. Monogr. No. 2.
Nelson, P. V. 1991. Greenhouse operation and management. Englewood Cliffs, NJ: Prentice-Hall.
Paris, J., J. Houle, M. Bricault, and H. Jackson. 1985. "Simultaneous evaluation of five thermal screens for greenhouses." Proc. of 9th Biennial Congr. of the Intl. Solar Energy Soc. 1:631–635.
Paris, J., J. F. Houle, M. Bricault, and H. A. Jackson. 1988. "Heat conservation in greenhouses by means of underground heat-storage and thermal curtains." Canadian Agricultural Eng. 30:133–142.
Pellet, H. M., and J. V. Carter. 1981. "Effect of nutritional factors on cold hardiness of plants." Hort. Rev. 3:144–171.
Perry, K. B., A. R. Bonanno, and D. W. Monks. 1992. 2 putative cryoprotectants do not provide frost and freeze protection in tomato and pepper. HortScience 27:26–27.
Rosenberg, N. J., B. L. Blad, and S. B. Verma. 1983. Microclimate: The biological environment. New York: John Wiley & Sons.
Sevillano, L., M. T. Sanchez-Ballesta, F. Romojaro, and F. B. Flores. 2009. "Physiological, hormonal and molecular mechanisms regulating chilling injury in horticultural species: Postharvest technologies applied to reduce its impact." J. of the Sci. of Food and Agr. 89:555–573.
Stamps, R. H. 1990. "Spunbonded polypropylene covers aid cold protection of Asparagus virgatus during radiation freezes." Proc. Fla. State Hort. Soc. 103:158–159.
Stamps, R. H. 1991. "Cold protection of leatherleaf fern using crop covers and overhead irrigation in shadehouses." HortScience 26:862–865.
Stamps, R. H. 1994. "Intermittent irrigation for cold protection conserves water in iced shadehouses during radiation freezes." Proc. Fla. State Hort. Soc. 107:204–207.
Stamps, R.H., S. Natarajan, L. Parsons, and J. Chen. 2010. "Water-based cold protection of foliage plants in shadehouses." HortScience 45:1668–1672.
Warr, J. C., G. J. Keever, D. A. Findley, and J. Kessler. 2004. "Time of pruning effects on cold hardiness of butterfly bush." J. Environ. Hort. 22:1–4.
Waters, W. E., and C. A. Conover. 1981. "Greenhouses, related structures, and environmental control." In Foliage Plant Production, ed. J. N. Joiner, 40–72. Englewood Cliffs, NJ: Prentice-Hall.
Acknowledgments
This publication and some of the research reported in this document were funded by the Southwest Florida Water Management District.