This is Activity 12 in a series of 25 in the Teach Aquaculture Curriculum.The introduction to this series is available at https://edis.ifas.ufl.edu/FA177.
Abstract
In this activity students will learn methods for spawning bivalve molluscs like clams or oysters using temperature manipulation. Students will use an ocular micrometer to measure the diameter of bivalve eggs and the length of bivalve larvae. The following lesson plan will have students monitor the density of larvae in their culture system and understand production protocols used in bivalve hatcheries.
Objectives
Students will be able to:
- Describe the reproductive biology and spawning of bivalve molluscs.
- Apply techniques used for spawning molluscs.
- Describe the conditions used in hatcheries for commercial production of bivalve molluscs.
Grade Level
9–12
Subject Area
Biology, Aquaculture
Time
Preparation: Spawning—indeterminant; daily exchange 10 minutes
Activity: Spawning—indeterminant; daily exchange 20 minutes
Clean-up: 10 minutes
Student Performance Standards (Sunshine State Standards)
06.03 Illustrate correct terminologies for animal species and conditions (e.g., sex, age, etc.) within those species (LA.910.1.6.1, 2, 3, 4, 5; SC.912.L.14. 19, 31, 33).
11.01 List and explain the meaning of morphology, anatomy, and physiology (LA.910.1.6.1, 2, 3, 4, 5; LA.910.2.2.2; SC.912.L.14.7).
11.02 List and describe the physiology of aquatic animals (LA.910.1.6.1, 2, 3, 4, 5; LA.910.2.2.2; SC.912.L.14.11, 12, 13, 14, 15, 16, 17, 18, 19, 21, 22, 28, 29, 31, 32, 33, 34, 36, 40, 41, 42, 43, 45, 46, 47,48, 51; SC.912.L. 18. 7, 8, 9).
11.10 List and describe the major factors in the growth of aquatic fauna and flora (LA.910.1.6.1, 2, 3, 4, 5; LA.910.2.2.2; SC.7.L.17.1, 2, 3).
12.01 Recognize and observe safety practices necessary in carrying out aquaculture activities (LA.910.1.6.1, 2, 3, 4, 5; LA.910.4.2.2, 5).
13.02 Explain how changes in water affect aquatic life (LA.910.1.6.1, 2, 3, 4, 5; LA.910.2.2.2; SC.912.L.17.2, 3, 7, 10).
13.03 Explain, monitor, and maintain freshwater/saltwater quality standards for the production of desirable species (LA.910.1.6.1, 2, 3, 4, 5; LA.910.2.2.2).
14.01 Identify factors to consider in determining whether to grow an aquaculture species (LA.910.1.6.1, 2, 3, 4, 5; LA.910.2.2.2; SC.7.L.17.3).
14.02 Identify/describe facilities used in a grow-out operation (LA.910.1.6.1, 2, 3, 4, 5; LA.910.2.2.2).
14.04 Determine the purpose and functions of a hatchery (LA.910.1.6.1, 2, 3, 4, 5; LA.910.2.2.2).
14.05 Identify and describe the sexual reproductive process and methods of reproducing aquaculture organisms (LA.910.1.6.1, 2, 3, 4, 5; LA.910.2.2.2; SC.7.L.16.3).
14.06 Identify and describe the spawning facilities used in aquaculture (LA.910.1.6.1, 2, 3, 4, 5; (LA.910.2.2.2).
15.01 Identify the types of growing systems and important factors in their selection, design, and use (LA.910.1.6.1, 2, 3, 4, 5; LA.910.2.2.2).
Interest Approach
Have students decide if most oysters and clams eaten in the United States are wild or cultured. Explain to them that most of the shellfish we consume in the United States are actually farmed in open, public waters along our coasts. Shellfish aquaculture utilizes the natural food in our coastal waters, and requires that the water be clean and free of toxins and harmful pathogenic bacteria. Therefore, shellfish farmers are at the forefront of environmental stewardship of our coastal waters because their livelihoods depend upon it. Polluted waters can be closed to shellfish farming operations. Next, have students discuss how different organisms reproduce. Then explain that oyster's and clam's mode of reproduction is called synchronous broadcast spawning. Tell them they will be conducting synchronous broadcast spawning in bivalves in the course of the lesson. This activity is designed to have students use the equipment and techniques associated with spawning molluscs.
Student Materials
- Introduction to the Biology of Molluscs section
- Spawning and Larval Culture of Molluscs (Lecture) http://www.irrec.ifas.ufl.edu/teachaquaculture/curriculum/4broodstock.php
- Hard Clam Spawning and Oyster Settlement Program videos http://www.irrec.ifas.ufl.edu/teachaquaculture/curriculum/4broodstock.php
Teacher Materials
See Table 1.
Student Instructions
- Read the Spawning and Larval Culture of Molluscs (Lecture) for homework in preparation for this laboratory.
- Once assigned to a group, prepare area to be used to spawn bivalves.
- Obtain warm seawater (35 g/L) at 32°C.
- Place the bivalves in a spawning container with seawater (35 g/L) at 22°C; add microalgae.
- Gradually (over a period of 15–30 minutes) add warm seawater to raise the temperature to 30°C.
- If the bivalves do not spawn within 30 minutes of reaching 30°C, gradually lower the temperature (over 10–20 minutes) by adding seawater at 22°C, then repeat the cycle. Very ripe bivalves may spawn on the first warming, or it could take several cycles of temperature fluctuations.
- If spawning does not occur after several cycles, the next option is to strip sperm from a male (dissected by the teacher prior to class), mix with seawater, and pipette into the spawning container to induce spawning.
- If spawning does not occur, it is most likely that the bivalves were not in reproductive condition; arrange to get conditioned bivalves from a commercial hatchery or other source.
Teacher Instructions
Preparations
- Obtain your clam or oyster specimens. Dissect a few to determine their ripeness and save for later.
- Divide your class into groups, or complete spawning as a demonstration.
- Ensure that all materials are available, particularly methods to change water temperature such as buckets of heated seawater (35 g/L) at 32°C (spawning is induced through thermal shock).
Activity
- The teacher should coordinate with students to facilitate the spawning process described above.
Post Work/Clean-Up
- When students are finished inducing spawning in their bivalves, have them remove live bivalves for disposal. All bivalves should be disposed of in a sealed plastic bag or container.
- Consult your school or district rules regarding proper disposal of clams. For further information visit http://www.nsta.org/about/positions/animals.aspx.
- Have each group thoroughly rinse and sanitize spawning containers, glassware, and other materials used for spawning.
Anticipated Results
- Students will observe or conduct broadcast spawning of bivalves and the methods used by hatcheries to induce spawning.
- Students will observe or conduct the fertilization of bivalve molluscs.
Support Materials
- Activity 12 Spawning & Rearing Bivalve Molluscs: Larval Culture http://irrec.ifas.ufl.edu/teachaquaculture/curriculum/4broodstock.php
- Video: Hard Clam Spawning http://irrec.ifas.ufl.edu/teachaquaculture/curriculum/4broodstock.php
- Video: Oyster Settlement http://irrec.ifas.ufl.edu/teachaquaculture/curriculum/4broodstock.php
- Whetstone, J. M., L. N. Sturmer, and M. J. Oesterling. 2005. Biology and culture of the hard clam (Mercenaria mercenaria). SRAC Publication No. 433. http://srac.tamu.edu
Explanation of Concepts
Methods of external reproduction in bivalve molluscs
Microscope skills and volume/density calculations
Biology of plankton and larval development
Introduction to the Biology of Molluscs
Molluscs are animals belonging to the phylum Mollusca. There are around 93,000 recognized living species of molluscs, making Mollusca the largest marine phylum with about 23% of all named marine organisms. Representatives of the phylum live in a wide range of habitats including marine, freshwater, and terrestrial environments. Molluscs are a highly diverse group in size, in anatomical structure, in behavior, and in habitat. The phylum is typically divided into nine or ten taxonomic classes, of which two are entirely extinct. Cephalopod molluscs such as squid, cuttlefish and octopus are among the most neurologically advanced of all invertebrates. The giant squid and the colossal squid are two of the largest known invertebrate species. The gastropods (snails and slugs) are by far the most numerous molluscs in terms of classified species, and account for 80% of the total number of classified molluscan species.
Molluscs have such a varied range of body structures that it is difficult to find defining characteristics that apply to all modern groups. The two most universal features are a mantle with a significant cavity used for breathing and excretion, and the structure of the nervous system. As a result of this wide diversity, many textbooks base their descriptions on a hypothetical "generalized mollusc." This has a single, "limpet-like" shell on top, which is made of proteins and chitin reinforced with calcium carbonate, and which is secreted by a mantle that covers the whole upper surface. The underside of the animal consists of a single muscular "foot." Although molluscs are coelomates (an organism with a coelom, a fluid-filled cavity), in bivalve molluscs the coelom is very small, and the main body cavity is a hemocoel (series of spaces between organs through which blood circulates termed an open circulatory system).
The "generalized" mollusc feeding system consists of a rasping "tongue" called a radula and a complex digestive system in which exuded mucus and microscopic, muscle-powered "hairs" called cilia play various important roles. The "generalized mollusc" has two paired nerve cords, or three in bivalves. The brain, in species that have one, encircles the esophagus. Most molluscs have eyes, and all have sensors that detect chemicals, vibrations and touch. The simplest type of molluscan reproductive system relies on external fertilization, but there are more complex variations. All produce eggs from which may emerge trochophore larvae, which are free-swimming and ciliated, more developed veliger larvae, or miniature adults. A striking feature of molluscs is the use of the same organ for multiple functions. For example, the heart and nephridia (kidney-like structures) are important parts of the reproductive system as well as the circulatory and excretory systems; in bivalves, the gills both "breathe" and produce a water current in the mantle cavity that facilitates excretion and reproduction.
The mantle secretes a shell that is mainly chitin and conchiolin (a protein) hardened with calcium carbonate, except that the outermost layer is all conchiolin. The mantle cavity is a fold in the mantle that encloses a significant amount of space, and was probably at the rear in the earliest molluscs, but its position now varies from group to group. The underside of the body generally consists of a muscular foot, which has been adapted for different purposes in different classes. In gastropods, it secretes mucus as a lubricant to aid movement. In molluscs that have only a top shell, such as limpets, the foot acts a sucker attaching to the animal to a hard surface, and the vertical muscles clamp the shell down over it; in other molluscs, the vertical muscles pull the foot and other exposed soft parts into the shell. In bivalves, the foot is adapted for burrowing into the sediment; in cephalopods it is used for jet propulsion, and the tentacles and arms are derived from the foot.
Most molluscs have one pair of gills, or only one gill. Generally the gills are feather-like in shape, although some species have gills with filaments on only one side. They divide the mantle cavity so that water enters near the bottom and exits near the top. Their filaments have three kinds of cilia, one of which drives the water current through the mantle cavity, while the other two help to keep the gills clean. Each gill has an incoming blood vessel connected to the hemocoel and an outgoing one connected to the heart.
Most gastropod molluscs have muscular mouths with radulae, "tongues" bearing many rows of chitinous teeth, that are designed to scrape bacteria and algae off rocks. The particles are sorted by a group of cilia, which send the smaller particles, mainly minerals, to the protostyle so that eventually they are excreted, while the larger ones, mainly food, are sent to the stomach's caecum (a pouch with no other exit) to be digested. Bivalve molluscs filter their food and are called ciliary feeders. Phytoplankton and fine particles are delivered to the mouth by ciliary currents from the gills and carried to the stomach where digestion begins. The intestine is usually long, ending close to the anus closely adjacent to the excurrent siphon. The principal function of the intestine is feces formation, achieved by mucous secretion and water absorption. Carnivorous gastropod molluscs usually have simpler digestive systems.
A typical mollusc has a pair of tentacles on the head containing chemical and mechanical sensors, a pair of eyes on the head, a pair of statocysts in the foot that act as balance sensors, and a pair of osphra (olfaction or smelling) reproductive systems. Two gonads sit next to the coelom that surrounds the heart. The gonads shed ova or sperm into the coleom, from which the nephridia extract them and emit them into the mantle cavity.
Spawning and Larviculture of Molluscs
Bivalve molluscs, such as clams, oysters, and scallops have unique reproductive biology in that they are all hermaphroditic, that is that they are both male and female during their lifetime. Clams and oysters are protandrous (begin as males and later develop into females). In contrast, many fish such as grouper are protogynous (females that later develop into males). Scallops are even more unique; they are simultaneous hermaphrodites. Oocytes (eggs) and sperm are located overlying the visceral mass (see bivalve anatomy). Oocytes range from pale yellow to white or orange and appear slightly granular. Sperm have a more creamy texture (Figure 1). As the gametes develop they can completely fill the body cavity within the two valves (shells), particularly in ripe females. Scientists developed the condition index to indicate the degree to which a bivalve's body cavity is filled with gametes.
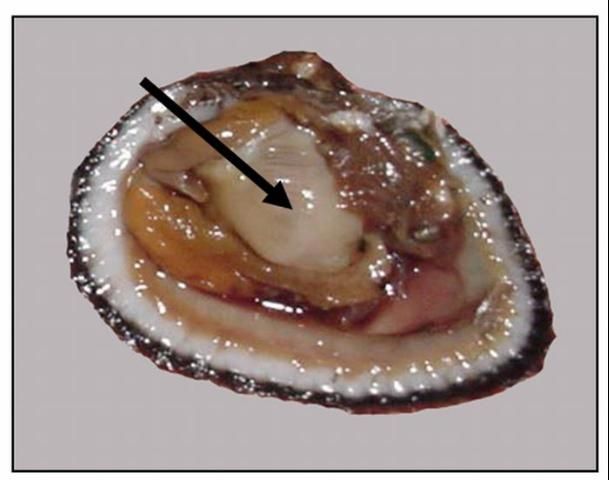
Credit: LeRoy Creswell, UF/IFAS
Bivalve molluscs are broadcast spawners, which means their eggs are fertilized outside their bodies. Males and females release their gametes through their excurrent siphon, and the eggs are fertilized in the open water. As a result, it is important that the eggs and sperm are released at the same time to ensure fertilization before currents carry the gametes in different directions; hence, the term synchronous spawning.
Bivalves become conditioned to spawn at a time that will optimize the survival of the planktonic larvae. Larvae will not survive if food is unavailable. They eat microscopic, unicellular, planktonic plants called phytoplankton. In temperate areas, such as New England and the mid-Atlantic region, phytoplankton standing crop gradually increases in the spring as the water warms. At higher latitudes, then, since temperature is the primary factor for phytoplankton growth, bivalves typically spawn in the late spring and then again in the fall after they have regenerated their gonads. In lower latitudes (tropical climates), the seasons are distinguished more by precipitation than by temperature (wet/dry season rather than winter/summer). In tropical climates, runoff of nutrients from the land plays the primary role in stimulating phytoplankton growth and bivalve reproductive condition. In Florida, which has a subtropical climate, bivalves respond to both of these factors. Along the east coast of Florida the spawning period for clams extends generally from early March through mid-May and again in October through November. Most large hatcheries have "conditioning tanks" that hold the broodstock at a constant, cool temperature (22°C). In order to bring the clams into spawning condition, significant amounts of algal feed are required, and usually only large hatcheries with year-round operations are equipped to handle the algal feed requirements. Small hatcheries typically collect broodstock from the environment when conditions are right, operating their larviculture system for a few months a year.
Spawning
When broodstock clams are in spawning condition, they should be transferred to a spawning table or trays; tables are usually long and narrow, less than 6 inches deep, and painted black to facilitate observation of the eggs and sperm (Figure 2). Glass or clear plastic trays can be placed on a black surface (or black paper) with the same result. Place the clams on a table or in trays and add cool seawater (20°C). If algal food is available, it should be added to induce the clams to extend their siphons and begin feeding. After about 20 minutes, and after the clams have their siphons extended, indicating that they are filtering, raise the water temperature to around 30°C over a 20–30 minute period. This technique is known as "thermal shock," and it provides a critical stimulus for synchronous spawning. Typically, male clams will begin to spawn before females, ejecting a constant stream of sperm into the water (Figure 3A). Females release eggs in a more sporadic fashion in response to the presence of sperm. If the clams do not begin to spawn within 30 minutes to an hour, the water temperature should be lowered again to 20°C and the process repeated. Interestingly, clams tend to spawn on rising temperature cycles during the spring, and falling temperature cycles in the fall.
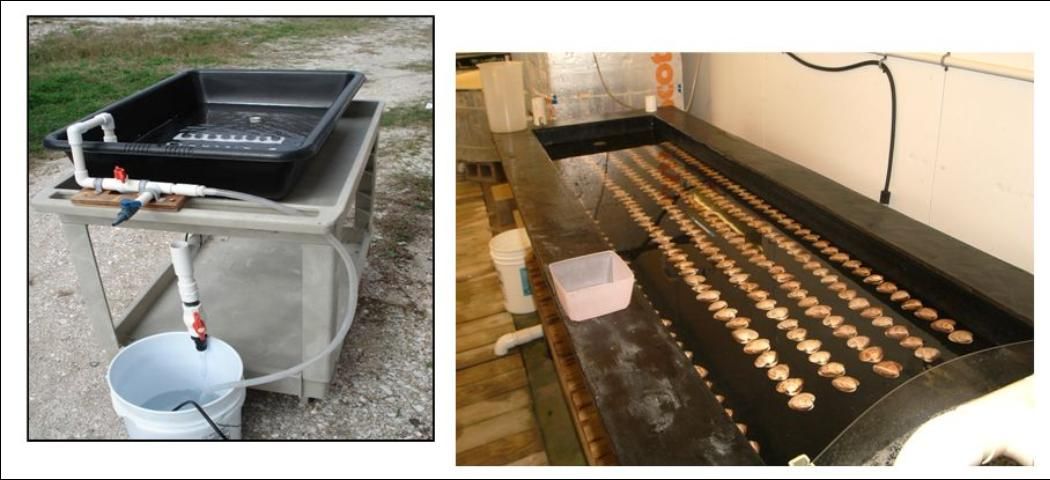
Credit: LeRoy Creswell, UF/IFAS
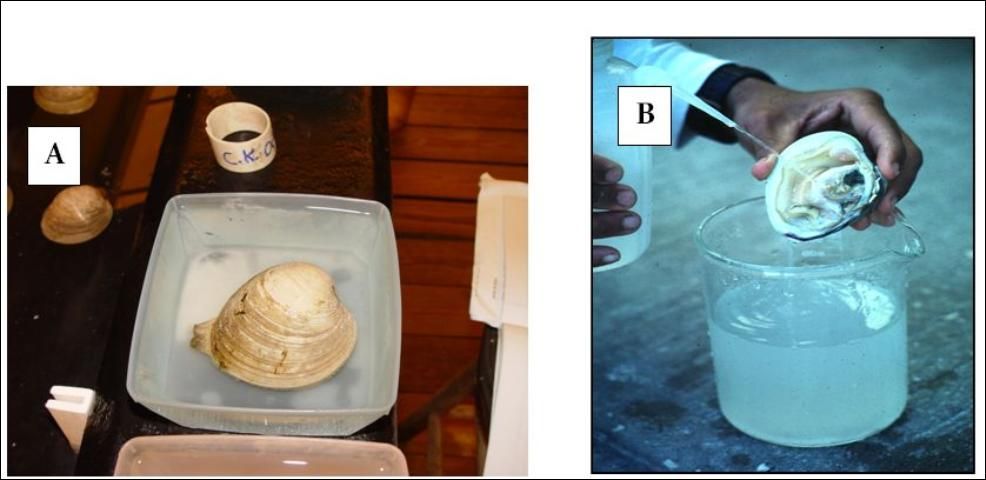
Credit: LeRoy Creswell, UF/IFAS
If clams fail to spawn after two or three heating/cooling cycles, sperm can be extracted from gonads of a dissected male and put in suspension with seawater (Figure 3B). The sperm water mixture is then pipetted into the spawning water near the incurrent siphon of a feeding female. The sperm ingested provides a strong stimulus for spawning, and if the females are in spawning condition, the technique is usually effective. Spawning males should be removed quickly from the spawning waters to avoid excessive amounts of sperm, which results in "polyspermy," a situation in which several sperm penetrate a single egg. Eggs fertilized by many sperm do not properly develop. Similarly, once females begin to spawn they should be isolated. A very slight amount of sperm is sufficient to fertilize all of the eggs. Fertilized eggs should be collected on a 20-μm (micrometers or microns) screen and thoroughly rinsed to remove excess sperm. The fertilized eggs are almost neutrally buoyant and require only slight aeration to keep them suspended in the water column.
Larviculture
The resulting fertilized eggs, now called zygotes, cleave within 45 minutes. The zygote develops into the multi-celled trochophore stage. The trochophore is a motile, non-shelled, ciliated mass that spins around randomly in the water column. After about 24 hours, trochophores develop into a shelled, free-swimming larval form, the straight-hinged or D-shaped veliger, still measuring approximately 60 μm and with a rudimentary shell. Extending out from between the two valves is the velum or velar lobes, a mass bordered by cilia (looking much like eyelashes) that create a sweeping motion used for swimming and for collecting food particles.
The new veligers are stocked in larval rearing tanks at a density of about 10 larvae/mL. For the next several days they will be moved daily to a new clean tank filled with filtered seawater. The veligers are drained onto fine mesh screens of specific size to retain them. They are counted, observed under a microscope to detect abnormalities, disease, or mortalities, and then returned to a new tank filled with seawater that has been filtered through a 5-μm filter and disinfected with ultraviolet (UV) light. Microalgal feed is added to the tank to achieve the appropriate algal cell density based on the number and size of the veligers (usually ranging between 10,000 and 40,000 algal cells/mL). The larvae actively feed on the phytoplankton for about 8–10 days at 28°C. As the veliger grows to 200 μm, the umbo, or raised beak of the shell, becomes prominent (the clam begins to look more like a clam), and the muscular foot the clam will use to burrow into the sediment begins to form. As the veliger reaches this final stage, called the pediveliger stage, it extends its foot in search of appropriate bottom sediments suitable for metamorphosis, also called settlement or set. If a substrate is detected, the larva will lose its ciliated velar lobes, and its free-swimming lifestyle, burrowing into the sediment to assume its relatively stationary, benthic, adult form. At this stage, a hard clam is about the size of the period at the end of this sentence.
Metamorphosis (Setting)
When the clam larvae reach the pediveliger stage, they are removed from the larviculture tank and transferred to a setting trough. The setting trough is shallow (about 30 cm deep by 60 cm wide by 240 cm long, although the exact dimensions and shape can vary) with a false bottom (about 2.5 cm above the bottom) covered in 115-μm mesh screen. Below the screen is a one-inch perforated PVC pipe running the length of the trough. The pipe is connected to an airlift pump that pumps the water from underneath the screen back to the water surface, creating a downwelling effect. This downward current places the pediveligers in contact with a substrate and facilitates metamorphosis. Algal food is added to the setting tray to sustain the larvae for the next 24–48 hours as they reabsorb their velar lobes and settle to the bottom. The newly set clams remain in the trough for two to three weeks until they will be retained on a 210-μm screen during sieving.
Other bivalves (those that do not bury into the sediment) are usually provided a hard substrate for setting. Oysters, for example, will attach to the side of a fiberglass tank and have to be physically removed. Typically, the culturist will provide oyster shell or concrete-coated fiberglass as a substrate. Probably the best approach is to use ground oyster shell (called micro-cultch) so that the newly set oyster (called spat) will be individually attached. Similarly, mussels, scallops, ark clams, and others will attach to surfaces using fine threads, called byssal threads or byssus. Culturists often use plastic "onion bags," polypropylene rope, or some other fibrous material as an attachment surface for these species.
Resources
Lorio, W. J. and S. Malone. 1995. Biology and Culture of the Northern Quahog (Mercenaria mercenaria) Southern Regional Aquaculture Center Publication 433. http://srac.tamu.edu/
Wallace, R. K., P. Waters, and F. S. Rikard. 2008. Oyster Hatchery Techniques. Southern Regional Aquaculture Center Publication 4302. http://srac.tamu.edu/
Helm, M. M. and N. Bourne. 2004. The hatchery culture of bivalves: a practical manual. FAO Technical Paper No. 471. http://www.fao.org/docrep/007/y5720e/y5720e00.htm
Other References
Adams, C. et al. 1991. Investing in commercial hard clam culture: A comprehensive guide to the south Atlantic states.Florida Sea Grant Report No. 104 (SGR-104). Gainesville, FL. 128 pp.
Huner, J. V. and E. E. Brown (Eds.). 1985. Crustacean and Mollusk Aquaculture in the United States. AVI Publishing Company, Inc., Westport, Connecticut. 476 pp.
Castagna, M. and J. Kreuter. 1981. Manual for growing the hard clam Mercenaria. Sea Grant Program, Virginia Institute of Marine Science, Gloucester Point, VA. 107 pp.
Landau, M. 1992. Introduction to Aquaculture. John Wiley and Sons, New York. 440 pp.
Manzi, J. and M. Castagna. (Eds.). 1989. Clam Mariculture in North America. Elsevier Press, New York, New York. 461 pp.
Manzi, J. and J. M. Whetstone. 1983. Intensive hard clam mariculture: A primer for South Carolina watermen. South Carolina Sea Grant Consortium Publication No. 91–01. 20 pp.
Quayle, D. B. and G. F. Newkirk. 1989. Farming Bivalve Molluscs: Methods for Study and Development. Advances in World Aquaculture, Volume 1. World Aquaculture Society, Baton Rouge, LA. 294 pp.
Sarkis, S. 2008. Installation and operation of a modular bivalve hatchery. FAO Fisheries Technical Paper No. 492. Rome, Italy. 173 pp.
Vaughan, D., L. Creswell, and M. Pardee. 1990. A manual for farming the hard shell clam in Florida. Tallahassee, FL: Florida Dept. of Agriculture and Consumer Services. 42 pp.