Introduction
Myxozoans are metazoan (multi-celled) parasites of invertebrates and fishes in both marine and freshwater environments. Though less common, some myxozoans infect amphibians, reptiles, birds, and mammals. This UF/IFAS Extension publication will discuss myxozoans that infect fishes and provide examples of species that infect aquarium and warmwater species.
Myxozoan infections in fish are often incidental (present but not causing significant disease); however, heavy parasite loads can lead to major disease outbreaks and heavy losses in aquaculture and wild fisheries. Most myxozoans target specific organs or tissues within one species of fish or a few closely related species, but some can infect a wider variety of fish species. Infections can last the lifetime of the fish, leaving the fish with permanent injury. Similar to other fish disease-causing organisms, some myxozoans have spread to areas of the world to which they are not native through the transport of infected fish and invertebrate hosts.
Myxozoan Size, Shape, and Structure
Myxozoans vary in appearance and are classified based upon morphology (size and shape) of the myxospore (the life stage commonly found in fishes) including the number and arrangement of shell valves and polar capsules (Figure 1). Myxozoa can have 2 to 13 shell valves (rarely 14 or 15) and 1 to 13 polar capsules (Lom and Dykova 2006, Okamura et al. 2015). Polar capsules contain a coiled, projectile, penetrating structure called a polar filament that is released and used to anchor the parasite to the host during infection.
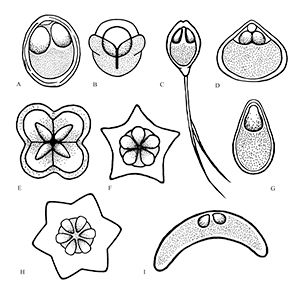
Credit: Kirstin Cook, UF/IFAS
General Life Cycle and Transmission
For those myxozoans infecting fish where the life cycle has been identified, most require two hosts to complete their life cycle: usually 1) the fish and 2) an invertebrate, either a certain type of worm or a bryozoan (Figure 2). For freshwater myxozoans, worm hosts are often oligochaete worms (segmented worms that lack a specialized head, such as tubifex worms) and for marine myxozoans, worm hosts may be polychaete worms (marine segmented worms, typically with "bristles" on each segment). However, direct fish-to-fish transmission through ingestion of infected tissue and exposure to contaminated water or individuals shedding spores has been demonstrated in some marine species. The life cycles and invertebrate hosts for most myxozoans are unknown.
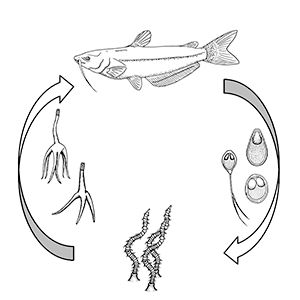
Credit: Kirstin Cook, UF/IFAS
Most fish myxozoans whose life cycles have been determined contain two main life stages: 1) the actinospore, present in the annelid (worm) host, and 2) the myxospore, present in the fish. The actinospore stage can look very different from the myxospore stage (Figure 3). Other developmental stages of actinospores or myxospores can also be found in the hosts. The mature actinospore released from the annelid host is the infective stage for the fish host and penetrates the skin or gill. The parasite migrates to its target tissue and may begin to increase in number before it gets there. Myxozoans often form "cyst-like" structures known as plasmodia (amoeba-like masses of material with many nuclei) within the target tissue. These contain parasites at different stages of development. Mature myxospores (the most easily identified stage found in fish) develop in these plasmodia and are either shed from infected hosts or released into the environment when the fish dies.
While myxozoans are associated with specific geographic ranges, transport of infected fish and annelid (worm) hosts can lead to spread of these organisms and sporadic outbreaks outside reported geographic ranges. Indeed, one study (Hallett et al. 2005) sampled oligochaetes sold as live feed for aquarium fish and found 12 types of actinospores in 6 of 7 samples, demonstrating the potential for introducing parasites into new areas or systems with naïve hosts that may be more susceptible to infection.
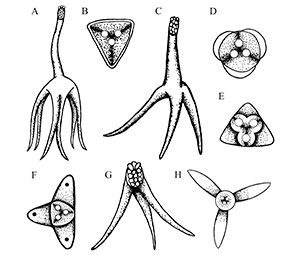
Credit: Kirstin Cook, UF/IFAS
Diagnosing Myxozoan Infections in Fish
As discussed previously, myxozoans in small numbers do not usually cause significant disease. Large numbers of them, however, can cause disease and significant losses. Myxozoan infections are limited to specific types of tissue. Diagnosing a myxozoan infection involves identifying characteristic spores within those target tissues and observing a few distinctive clinical signs.
Grossly (i.e., without use of a microscope), myxozoan-infected tissue may look like a mass, nodule, granuloma, tissue discoloration, or cyst. However, because these changes can be seen in other diseases and because some myxozoans produce no gross lesions at all, a proper diagnosis requires microscopic examination of fresh tissues (wet mounts) (Figure 4) or other tests (histopathology, see Figure 5, or molecular methods such as PCR) to be carried out by a fish health professional or diagnostic lab.
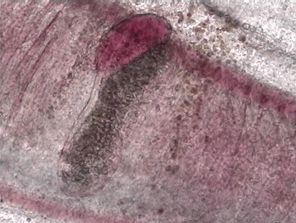
Credit: UF/IFAS Tropical Aquaculture Laboratory
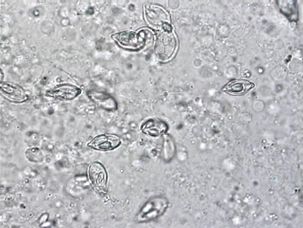
Credit: UF/IFAS Tropical Aquaculture Laboratory
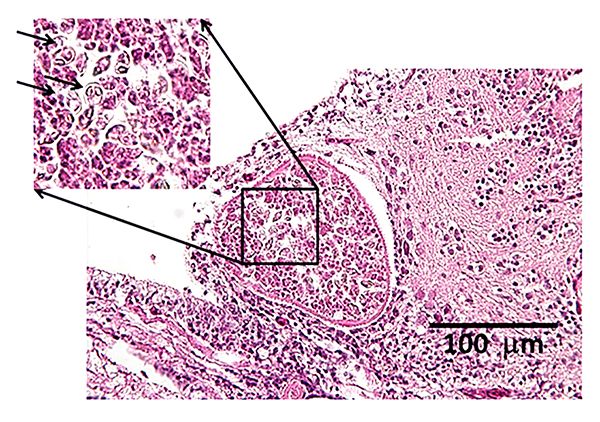
Credit: UF/IFAS Tropical Aquaculture Laboratory
The presence of spores containing polar capsules in affected tissues confirms the diagnosis. Spores are more easily detected on histological sections using special stains (Giemsa or Modified Wright's stain). A strong immune response causing inflammation can make spore detection difficult. Spores, if present in easily accessible, soft tissues, can be identified by examining wet mounts and smears. Fresh, unfixed spores are needed in order to morphologically identify organisms to the genus level, because fixative solutions can cause artifact changes. Molecular techniques (PCR) and careful morphological analysis are often required for reliable species-level identification.
Myxozoans of Importance in Warmwater Fish
Henneguya spp.
There are over 200 known species of Henneguya, one of the largest groups. Most Henneguya spp. infections have been described in freshwater fish, but a few have been identified in marine species. The Henneguya life cycle has only been determined for four species, all of which involve an intermediate host. Three species (H. ictaluri, H. exilis, and H. mississippiensis) involve the channel catfish, Ictalurus punctatus and the freshwater tubificidan worm, Dero digitata, as hosts. H. nuesslini, on the other hand, requires a host brown trout or brook trout followed by infection of the tubificidan worm Tubifex tubifex. These worms proliferate in detritus-laden water, allowing myxozoans to proliferate and lead to severe infections in fish.
Henneguya species are important pathogens in the US channel catfish aquaculture industry. H. ictaluri is the causative agent for hamburger gill disease, aka proliferative gill disease in channel catfish. Grossly, the gills are blunted, swollen, and look like "hamburger meat." Affected fish are usually in respiratory distress. The parasite damages the gill cartilage leading to cartilage fracture and remodeling seen on wet mounts and blunting seen grossly. Low to high mortality is seen in H. ictaluri outbreaks, with major outbreaks occurring in the spring and minor outbreaks in the fall. Infections can occur in any age class, but most severely impact juvenile fish. Another gill myxozoan of channel catfish, H. exilis, also damages the gill by forming pseudocysts containing plasmodia within the gill lamellae.
Depending on the species, Henneguya may either form grossly visible cysts at the ends of gill filaments, microscopic pseudocysts within the gill tissue, or no discernable cysts, as is the case with H. ictaluri. While the gill is the target tissue for many Henneguya species, some species may target other tissues including the skin and fins, urinary bladder, kidney, gall bladder, intestine, or ovary. Like other infections caused by myxozoans, Henneguya infections are often diagnosed based on the shape of the parasite on wet mount examination, although H. ictaluri is rarely seen on wet mount examination. The Henneguya spore is ellipsoid (oval) in shape with two shell valves that taper into two tails usually about twice as long as the spore body (Figure 6). The anterior (front) portion of the spore body contains two parallel, elongate polar capsules containing the polar filaments. The posterior (back) portion next to the tail contains a large vacuole (an enclosed, membrane-bound compartment containing various compounds) and two nuclei. Henneguya myxospore measurements vary slightly between species. A review of the genus by Eiras (2002) provides information on size measurements, target tissues infected, fish hosts, and countries where specific species were discovered. The myxospore of H. mississippiensis (not included in the review) is similar and slightly smaller compared to H. ictaluri and measures 14.4–19.3 microns (µm) (about 1.4 to 1.9 hundredths of a millimeter) in spore body length, 40.7–61.6 µm (4.1–6.2 hundredths of a millimeter) in total length, and 4.5–5.5 µm (4.5–5.5 thousandths of a millimeter) in width. (There are 1000 microns in one millimeter.) Because the spores of many species are very similar, molecular methods (e.g., PCR) are often used to accurately determine species. While no treatments exist for Henneguya infections, supportive care in the form of supplemental aeration can help decrease mortalities due to gill damage caused by H. ictaluri. Alternatively, fish may be harvested and processed early if near market size; however, many often die before they reach the processing facility and need to be discarded.
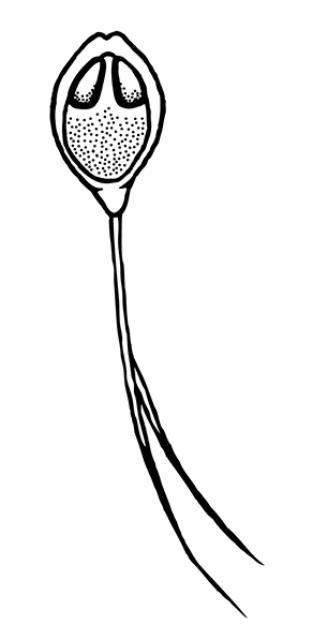
Credit: Kirstin Cook, UF/IFAS
Kudoa spp.
Worldwide, there are 95 described species in the genus Kudoa. Some infect a specific host while others have a wide host range (many different species), infecting warm and coldwater marine to brackish teleosts and elasmobranchs (sharks, rays, skates). The best-studied of these is Kudoa thyrsites, an important parasite of Atlantic salmon and several other marine species. K. thyrsites infestation causes the muscle tissues in dead fish to liquefy, a process known as "soft flesh syndrome." This parasite is well-known in salmonid aquaculture, and several other related species have been identified in the Gulf of Mexico and southeastern United States in important game fishes. Recently, K. inornata was identified as a frequent cause of soft flesh syndrome in the skeletal muscle of spotted sea trout from South Carolina.
Additional Kudoa species described in the region include: K. hypoepicardialis (Figure 7) in bluefish, black drum, Warsaw grouper, Atlantic tripetail, man-of-war fish, and red snapper; K. ovivora in bluehead wrasse, slippery dick wrasse, yellowhead wrasse, blackear wrasse, and bucktooth parrotfish; K. shkae in hardheaded catfish; K. leiostomi and K. branchiata in spot fish; K. cerebralis in striped bass; and K. crumena in Spanish mackerel. Kudoa species have also been diagnosed in cobia (unpublished data). The life cycle for Kudoa species has not been determined; however, fish-to-fish transmission has been suggested for K. ovivora. Most of the previously mentioned species do not cause outward signs of disease, though K. hypoepicardialis, K. ovivora, and K. branchiata may cause gross lesions with heavy infections in the heart, ovaries, and gills, respectively.
Kudoa species have specific target tissues, which most commonly include muscle, gills, or, less often, the connective tissue of the central nervous system and visceral organs. Spore shapes and sizes vary for different Kudoa species. The myxospore for K. inornata measures 5.3–5.5 µm in length, 5.8–6.0 µm in width, and 5.9–6.1 µm in thickness. Kudoa species have square, rectangular, or star-shaped myxospores and other characteristics used by parasite experts (e.g., two uninucleate sporoplasms where one envelops the other, and indistinct sutures between four valves, each containing one polar capsule). The presence of myxospores in target tissues as seen on fresh wet mounts (muscle squashes) and histopathology aids in the identification of this parasite. Molecular techniques have been developed for a handful of species, particularly K. thyrsites. There are no known treatments for Kudoa myxozoans. Because potential intermediate hosts of Kudoa sp. remain unidentified, management strategies for avoiding infection are limited to general myxozoan control strategies discussed below.
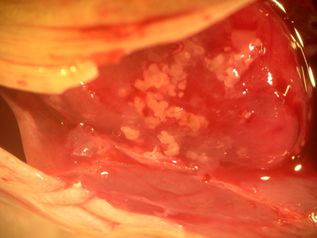
Credit: UF/IFAS Tropical Aquaculture Laboratory
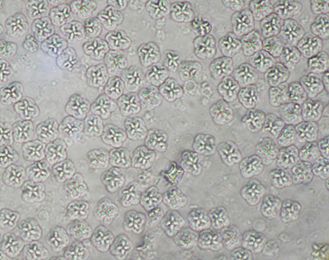
Credit: UF/IFAS Tropical Aquaculture Laboratory
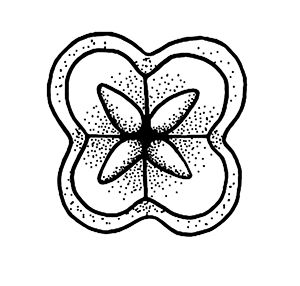
Credit: Kirstin Cook, UF/IFAS
Hoferellus carassii
Primarily a disease of goldfish, Hoferellus carassii is the cause of "kidney bloater disease." As the name suggests, fish typically present with asymmetrical, severe coelomic (body cavity) swelling due to abnormal enlargement of the kidneys. However, other diseases can cause swelling, so a complete diagnostic work up is required. In the case of H. carassii infection, the swim bladder may become displaced, leading to buoyancy issues, but otherwise, the kidney is typically the only affected organ. The kidney is often filled with cysts and enlarged due to the swelling and abnormal increased growth of the kidney tubules. In advanced stages of disease, a yellow fluid can be found within some of the dilated tubules.
The disease is typically seen in goldfish production facilities of Europe, North America, and Asia. Fish become infected in the summer, begin to show clinical signs in the fall, and experience the most severe clinical signs and mortality with the production of spores in the spring. Myxospores are shed in the spring through the urine.
Similar to Henneguya, Hoferellus carassii requires both the fish and an oligochaete worm host (tubificid, i.e., tubifex-like worms). The oligochaetes Branchiura sowerbyi and Nais cf. elingus have been implicated as intermediate hosts for H. carassii. The presence of myxospores in lesions on either tissue wet mounts or histopathology, particularly in the kidneys, supports the diagnosis (Figure 8). H. carassii myxospores are lemon-shaped with posterior projections. The myxospore averages 8.4 µm in width anteriorly and tapers to 4.1 µm posteriorly, 13.0 µm in length, and 7.6 µm in thickness. No treatments exist for H. carassii, but disease control may be achieved by disinfecting ponds and restocking with known, uninfected fish.
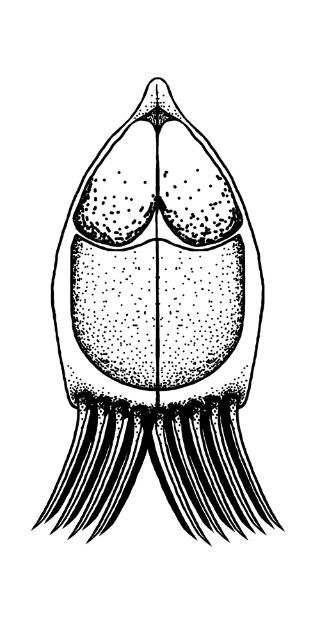
Credit: Kirstin Cook, UF/IFAS
Enteromyxum leei
A myxozoan parasite of sea bream and other tropical marine fish in Europe and Asia, Myxidium leei was recently reclassified as Enteromyxum leei based on genetic work. This myxozoan is responsible for devastating production losses in several sea bream species in aquaculture facilities from the Mediterranean and Asia. Affected fish have poor weight gain, weight loss, poor feed conversion, reduced marketability, and mortality. Additionally, E. leei causes morbidity and mortality in a number of tropical fish species housed in public aquaria, particularly in fish belonging to the families Labridae (wrasses) and Blenniidae (blennies). With a broad host range, E. leei infections have been associated with high temperatures, high fish biomass, or transportation stress, making quarantine essential for preventing major outbreaks or accidental introduction of the parasite to established collections. While E. leei has not been reported in North America to date, care should be taken when importing susceptible species to ensure it is not introduced. A number of native North American species, most notably red drum, are susceptible to infection. Introduction of this pathogen to non-native areas has previously occurred with the importation of infected sharpsnout sea bream and common sea bream to the Red Sea. Quarantine and routine screening of newly acquired fish from endemic areas are essential to preventing the introduction of this myxozoan into North America.
Disease signs often vary depending on the host species, but the disease typically progresses to severe inflammation of the intestine with clinical signs including emaciation, discoloration, scale loss, and death. Gross necropsy findings may also include distended gall bladder, soft and pale liver, and thickened intestinal walls with occasional hemorrhage. A direct life cycle has been reported for E. leei, meaning it can be transmitted from one fish to another. Fish-to-fish transmission may be by exposure to contaminated effluent or by ingestion of infected tissue. It is not clear if it also has an annelid host like other myxozoans. Fish possibly consume the myxospores, which travel through the gut mucosa to infect the fish, leading to intestinal disease and emaciation. Like other myxozoans, E. leei is diagnosed by identifying characteristic spores in either tissue wet mounts or histopathology, particularly in sections of the gastrointestinal tract. E. leei myxospores are bow-shaped and semicircular, averaging 6.9 microns in width, 14.7 microns in length, and 6 microns in thickness. In lateral or frontal view, the sporoplasm is seen centrally as an irregularly shaped hourglass with a large central vacuole and two elongate polar capsules on each side that taper distally. No treatments exist for E. leei.
Treatment, Prevention, and Control
Currently, there are no effective drugs available for the treatment of myxozoan infections. Myxospores are often resistant to many common disinfectant regimens, making disinfection and quarantine challenging. Oligochaete (e.g., tubifex and tubifex-like worms) and polychaete (marine worms, including bristleworms) management is an important aspect for controlling these parasites. Producers should avoid using oligochaete and polychaete worms as live feed because these worms may harbor infective myxospores. Reducing habitat for oligochaete populations by using concrete ponds and selective breeding for resistant fish strains contributed to decreased myxozoan disease prevalence in farmed trout operations. In natural pond production, regular and frequent cleaning and mucking of ponds, particularly after harvesting, removes the detritus that tubificid worms, particularly Dero digitata, feed on, reducing their numbers. Producers should also quarantine any newly imported or introduced fish because these are potential sources of introducing myxozoans. Fish showing clinical signs should be submitted to a fish health professional for complete necropsy and histopathologic evaluation. Additionally, treating incoming water sources with ozone and ultraviolet irradiation has been effective in removing some myxozoans.
In cases where myxozoans are endemic (already present) to a facility and preventing exposure is not possible, supportive care can help minimize stress on the fish. Proper supportive care will depend on the myxozoan infection and clinical signs, but may include supplemental oxygen (for gill infections), altering salinity or temperature, lowering fish density, etc.
When myxozoans are introduced to fish in areas that were previously "clean" of that species of myxozoan, it is recommended that one isolate the infected population and depopulate and disinfect the affected environment to help prevent disease spread and establishment in new areas, and/or to reduce the loading. Usually, ponds are disinfected with hydrated lime after they have been harvested, drained, and cleaned. The lime rapidly increases pH to 10 or 11, which kills many disease agents and pests, including oligochaetes. Please see UF/IFAS Fact Sheet FA-38 for more information on the use of lime. Some attempts have been made to use potassium permanganate to decrease populations of the oligochaete Dero digitata in channel catfish ponds; however, a recent study demonstrated it to be ineffective in production ponds. Hard surfaces and field gear (not earthen ponds) can be disinfected with benzalkonium chloride (Quaternary Ammonium Compounds, QAC; 500ppm for 10 minutes), didecyl dimethyl ammonium chloride (a QAC; 400ppm for 5 minutes), or chlorine (200–500ppm for 15 minutes) (Bowker et al. 2014). With no effective treatments and few environmental disinfectants, preventing the introduction of myxozoans is the best management strategy.
Summary
While often incidental and not the primary cause of a disease outbreak, under certain conditions myxozoan infections can have a profound impact on aquaculture facilities, commercial and recreational fisheries, and public aquariums, leading to significant morbidity and mortality and impacts on growth performance. Clinical signs vary depending upon the type of myxozoan and the tissue infected, but diagnosis is usually straightforward— identification of characteristic spores in target tissues on wet mounts, stained tissue smears, histopathology, or molecular testing. Limiting introduction of myxozoans by using good biosecurity is key because no effective treatments exist and disinfecting the environment is challenging. Depending upon a producer's goals and the myxozoan(s) identified, options during outbreaks include providing supportive care or depopulation and disinfection.
References and Selected Readings
Blaylock, R. B., S. A. Bullard, and C. M. Whipps. 2004. "Kudoa hypoepicardialis n. sp. (Myxozoa: Kudoidae) and associated lesions from the heart of seven perciform fishes in the northern Gulf of Mexico." Journal of Parasitology. 90(3):584–593.
Bowker, J. D., J. T. Trushenski, M. P. Gaikowski, and D. L. Straus, Editors. 2014. "Guide to using drugs, biologics, and other chemicals in aquaculture." American Fisheries Society Fish Culture Section. http://www.fws.gov/fisheries/aadap/PDF/guidetousingdrugs.pdf (accessed March 2020)
Cobcroft, J. M., and S. C. Battaglene. 2013. "Ultraviolet irradiation is an effective alternative to ozonation as a sea water treatment to prevent Kudoa neurophila (Myxozoa: Myxosporea) infection of striped trumpeter, Latris lineata (Forster)." Journal of Fish Diseases. 36(1):57–65.
Diamant, A., J. Lom, and I. Dyková. 1994. "Myxidium leei n. sp., a pathogenic myxosporean of cultured sea bream Sparus aurata." Diseases of Aquatic Organisms. 20:137–141.
Diamant, A. 1997. "Fish to fish transmission of a marine myxosporean." Diseases of Aquatic Organisms. 30:99–105.
Dyková, I., I. de Buron, I. Fiala, and W. A. Roumillat. 2009. "Kudo inornata sp. n. (Myxosporea: Multivalvulida) from the skeletal muscles of Cynoscion nebulosus (Teleostei: Sciaenidae)." Folia Parasitologica 56(2):91–98.
Eiras, J. C. 2002. "Synopsis of the species of the genus Henneguya Thélohan, 1892 (Myxozoa: Myxosporea: Myxobolidae)." Systematic Parasitology. 52:43–54.
Eiras, J. C., A. Saravia, and C. Cruz. 2014. "Synopsis of the species of Kudoa Meglitsch, 1947 (Myxozoa: Myxosporea: Multivalvulida)." Systematic Parasitology. 87:153–180.
Feist, S., and M. Longshaw. 2010. "Phylum Myxozoa." Fish Diseases and Disorders, Volume 1: Protozoan and Metazoan Infections. Ed. P. T. K Woo. 2nd ed. Vol. 1. CABI. Wallingford, Oxfordshire, UK. 230–296.
Gleeson, R. J., M. B. Bennett, and R. D. Adlard. 2010. "First taxonomic description of multivalvulidan myxosporean parasites from elasmobranchs: Kudoa hemiscylli n. sp. and Kudoa carcharhini n. sp. (Myxosporea: Multivalvulidae)." Parasitology. 137:1885–1898.
Hallett, S. L., S. D. Atkinson, C. Erséus, and M. El-Matbouli. 2005. "Dissemination of triactinomyxons (Myxozoa) via oligochaetes used as live food for aquarium fishes." Diseases of Aquatic Organisms. 65:137–152.
Katharios, P., G. Rigos, and P. Divanach. 2011. "Enteromyxum leei (Myxozoa), a lethal intruder of tropical pet fish: first case in Humphead Wrasse, Cheilinus undulatus (Rüppell, 1835)." Journal of Exotic Pet Medicine. 20(2):138–143.
Lazur, A. M., C. E. Cichra, and C. Watson. 2013. The Use of Lime in Fish Ponds. FA38. Gainesville: University of Florida Institute of Food and Agricultural Sciences. https://edis.ifas.ufl.edu/publication/fa028 (accessed February 2024)
Lom, J. and I. Dyková. 2006. "Myxozoan genera: definition and notes on taxonomy, life-cycle terminology and pathogenic species." Folia Parasitologica. 53:1–36.
Mackenzie, K. and C. Kalavati. 2014. "Myxosporean parasites of marine fishes: their distribution in the world's oceans." Parasitology. 141(13):1709–1717.
Mischke, C. C., M. J. Griffin, D. J. Wise, and T. E. Greenway. 2014. "Potassium permanganate is not an effective pond disinfectant to control Dero digitata." Journal of the World Aquaculture Society. 45(3):350–353.
Moran, J. D. W., D. J. Whitaker, and M. L. Kent. 1999. "A review of the myxosprean genus Kudoa Meglitsch, 1947, and its impact on the international aquaculture industry and commercial fisheries." Aquaculture. 172:163–196.
Noga, E. J. 2010. Fish Disease: Diagnosis and Treatment. 2nd ed. Wiley-Blackwell, Ames, Iowa.
Padrós, F., O. Palenzuela, C. Hispano, O. Tosas, C. Zarza, S. Crespo, and P. Alvarez-Pellitero. 2001. "Myxidium leei (Myxozoa) infections in aquarium reared Mediterranean fish species." Diseases of Aquatic Organisms. 47:57–62.
Pavanelli, G. C., J. C. Eiras, and A. Saraiva. 1998. "Henneguya spp. (Myxozoa, Myxosporea, Myxobolidae) parasitizing fishes from Paraná river, Brazil." Acta Scientiarum. 20(2):161–163.
Okamura, B., A. Gruhl, and J. L. Bartholomew. (eds) 2015. Myxozoan Evolution, Ecology, and Development. Cham, Switzerland: Springer International Publishing.
Roberts, R. J. 2012. Fish Pathology. 4th ed. Wiley-Blackwell, Ames, Iowa.
Rosser, T. G., M. J. Griffin, S. M. A. Quiniou, L. H. Khoo, T. E. Greenway, D. J. Wise, and L. M. Pote. 2015. "Small subunit ribosomal RNA sequence links the myxospore stage of Henneguya mississippiensis n. sp. from channel catfish Ictalurus punctatus to an actinospore released by the benthic oligochaete Dero digitata." Parasitology Research. 114:1595–1602.
Swearer, S. E., and D. R. Robertson. 1999. "Life history, pathology and description of Kudoa ovivora n. sp. (Myxozoa, Myxosporea): an ovarian parasite of Caribbean labroid fishes." Journal of Parasitology. 85(2):337–353.
Trouillier, A., M. El-Matbouli, and R. W. Hoffmann. 1996. "A new look at the life cycle of Hoferellus carassii in the goldfish (Carassius auratus auratus) and its relation to 'kidney enlargement disease' (KED)." Folia Parasitologica. 43:173–187.
Yokoyama, H., K. Ogawa, and H. Wakabayashi. 1993. "Involvement of Branchiura sowerbyi (Oligochaeta: Annelida) in the transmission of Hoferellus carassii (Myxosporea: Myxozoa), the causative agent of kidney enlargement disease (KED) of goldfish Carassius auratus." Gyobyo Kenkyu. 28(3): 135–139.