Production Facts
- In 2019, Florida ranked first nationally in watermelon production, accounting for 25.1% of the total US production, 29.6% of the US crop’s total value, and 24.7% of the national watermelon acreage (USDA/NASS 2021).
- Florida’s cash receipts for watermelon production totaled $161.54 million in 2019. During the 2019 production season, watermelon was the state’s third-ranking vegetable crop in terms of value. The watermelon production value was approximately 13.2% of the total production value of all Florida vegetables (USDA/NASS 2021).
- In 2020, Florida growers planted 26,300 acres of watermelons and harvested 25,200 acres. The average yield was 38,080 pounds per acre for a total production of 958.65 million pounds. Harvested acreage during the past decade has ranged from 19,700 acres harvested in 2014 to 25,200 acres harvested in 2020 (USDA/NASS 2021).
- In 2017, Florida had 431 farms producing watermelon representing approximately 9 % of the state’s vegetable harvested acreage. Of those, 59.2% produced watermelons on less than 5 acres, 12.5% maintained 5–49.9 acres of watermelons, 22.3% planted 50–249.9 acres, and 6% planted more than 250 acres of watermelons (USDA/NASS 2021).
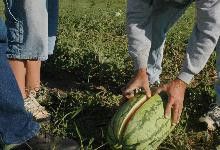
Credit: UF/IFAS Communications
- Florida is the only US supplier of watermelons from December to April. Florida watermelons are harvested throughout the year, although the vast majority of production is harvested from May to July (Bertelsen et al. 1994).
- Growers received approximately $0.164 per pound in 2020, and during the past decade, this price has ranged from $0.144 per pound in 2016 to $0.223 per pound in 2013 (USDA/NASS 2021).
- The average total production cost of conventional watermelon production in North Florida is estimated at $5,559/acre. Production costs include fixed production overhead ($518), variable operating costs ($2,565), and harvesting and marketing ($2,476). Net returns can range from $1,350 to $8,209/acre depending on yield per acre and market price. (Athearn et al. 2020).
- Production costs can be higher in South Florida than in North Florida. But South Florida growers can enter the market early, providing them with prices up to two to three times higher than those received in North Florida (Hochmuth et al. 1997).
Production Regions
Watermelons are grown throughout Florida. The greatest production region is the north to northcentral region, including Levy, Gilchrist, Marion, Alachua, Hillsborough, Hendry, Suwannee, Desoto, and Hardee Counties. In 2017, this region comprised 65.2% of the harvested acreage and 39.2% of the state’s watermelon-producing farms. The south and west-central region of Florida (including Collier, Charlotte, Manatee, and Polk Counties) and Jackson County in the Florida panhandle comprised 16.9% of the acreage and 13% of the watermelon producing farms in that year. The remaining watermelon production is distributed among 53 of the state’s other counties (USDA/NASS 2021).
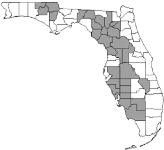
Credit: undefined
Production Practices
Watermelons require a long, warm growing season and grow best under temperatures of 70°F–85°F (21°C–29°C), tolerating temperatures up to 90°F (32°C). The plant also tolerates high humidity (Bertelsen et al. 1994). Although watermelons are produced on a number of soil types, the crop does not do well on muck soils (Spreen et al. 1995). The optimum pH range for watermelons is 6.0–6.5, although the plant will tolerate soils with pH as low as 5 (Maynard 2003). Soil temperature also affects watermelon production. Frost easily damages seedlings, and germination is very slow when the soil temperature is less than 70°F (21°C). Watermelon growers in Florida have a planting window of only a few weeks and must balance the risk of losses from frost damage with maximizing economic returns from an early harvest (Hochmuth et al. 1997).
Both seeded and seedless watermelons are produced in Florida, but seedless melons now dominate the market. Seeded watermelon varieties available in Florida include but are not limited to ‘Estrella’, ‘Jamboree’, ‘Mardi Gras’, ‘Sangria’, ‘Sentinel’, ‘Starbrite’, ‘Stargazer’, ‘Summer Flavor 790’, ‘Summer Flavor 800’, and “Top Gun’. Seedless watermelon varieties available include but are not limited to ‘Affirmed’, ‘Captivation’, ‘Crunchy Red’, ‘Fascination’, ‘Joy Ride’, ‘Kingman’, ‘Melody’, ‘Sugar Fresh’, ‘Sweet Polly’, ‘TriX313’, ‘Troubadour’, and ‘7187 HQ’ (Paret et al. 2021).
Seedless varieties are sterile hybrids, and the seeds are produced by crossing a normal watermelon and one that was genetically changed through chemical treatment at the seedling stage. When pollinated with normal watermelon plants, seedless plants produce only small, white undeveloped seedcoats. These are soft and tasteless and can be eaten with the watermelon flesh. The parent watermelon of a seedless plant produces only 5%–10% as many seeds as the normal plant, resulting in seeds that cost 5–10 times greater than seeded hybrid varieties and 10–100 times greater than standard, openpollinated varieties. Seedless varieties require soil temperatures above 80°F (26.7°C) for germination, and both germination and seedling emergence are slower for seedless varieties (Maynard 1996; Stephens 2012).
Seedless varieties of watermelons are transplanted because of the high cost of hybrid seeds. In addition, seedless varieties cannot be established by direct seeding (Bertelsen et al. 1994). A wide mix of cultural practices can be used but most acreage is now produced using plastic mulched beds and transplants. Some direct seeding of seeded watermelon is still practiced but mostly on small scale for local sales.
When transplants are used, they are first grown in greenhouses and are usually fieldready in 3–5 weeks. Bareroot transplants cannot be used. Instead, transplants are grown in planter flats to maintain the root and soil ball (Hochmuth and Elmstrom 1992; Vavrina 1992).
Transplanting watermelons permits earlier harvesting, particularly if used with plastic mulch (Hochmuth et al. 1997). Yields are generally higher for transplanted watermelons, and the resulting plant uniformity makes cultivation easier. Production costs for directseeded and transplanted watermelons were similar in a comparison of the costs and returns of both methods grown on plastic mulch in North Florida in 1995. However, transplanted watermelons had higher profitability because of higher yields and a higher market price as a result of early harvest (Hewitt 1996). Directseeding also has advantages, including lower labor requirements, the availability of precision planting (which improves efficiency), and the possible production of greater vine area (which reduces sunburn) (Vavrina 1992; Hewitt 1996).
In South Florida, watermelons are primarily grown on plastic mulch as a second crop in a double-cropping system, following tomato or pepper. North Florida growers use the mulch to warm the soil, allowing them to plant earlier in the season and ship their product to market early. Using polyethylene mulch lets producers harvest earlier, and it aids in weed control and improves the efficiency of water and fertilizer use. Plastic mulch is also a requirement for soil fumigation. When plastic mulch is used, a bed press shapes a smooth bed to maximize contact between the mulch and the bed surface. Fertilizer and soil-applied pesticides are added to the bed before the mulch is laid down. Plastic mulch measuring between 48–72 inches (122–183 cm) wide is placed on beds measuring between 20–30 inches (51–76 cm) across the top. Mulch used for doublecropping needs to be able to survive two crop seasons (Spreen et al. 1995; Hochmuth and Elmstrom 1992).
If this system is used, worker activities for the season begin with laying mulch. Overall, worker activities mostly include tractordriven operations, such as cultivation, fertilization, and laying drip tape. The only field task is shoveling dirt on the mulch to bury it, which generally requires three people on each end. A tworow plastic mulch applicator will cover about 8 acres a day. With an average size farm of 40 acres, shovel crews would be needed approximately 40 hours (5 days) a year. Placing emitters on the irrigation main line requires hand labor, and one worker can cover 15–20 acres a day. Workers setting transplants (approximately 5 days for a 40acre farm) often wear latex gloves. Workers with poles also move vines out of row middles for the layby fertilizer application made at midseason.
Primary planting dates for watermelons in Florida range from December 15 to April 15. Planting extends from December 15 to March 1 in South Florida, from January 15 to March 15 in Central Florida, and from February 15 to April 15 in North Florida. A small amount of acreage scattered among South, Central, and North Florida is planted for a fall harvest (October through December) (Hochmuth et al. 1997; Olson et al. 2012).
Seeds are planted with 60–108 inches (152–274 cm) between rows and 24–72 inches (61–183 cm) between plants, giving a plant population of 4,356 per acre at the closest spacing. Optimum yield under irrigation is generally obtained with 72–96 inches (183–244 cm) between rows and 24–48 inches (61–122 cm) between plants. Seeds are planted at a depth of 1.5–2 inches (3.8–5.1 cm).
Seed to maturity requires 80–100 days, and transplanting to maturity requires 60–90 days, depending on the variety (Spreen et al. 1995).
Moderate amounts of fertilizer are required to achieve adequate yields in watermelon production. The plant responds better when nitrogen and potassium applications are split, but fertilizer application methods vary according to the production and irrigation system. For example, when fullbed mulch production with overhead irrigation is used, fertilizers are incorporated into the bed before the plastic mulch is applied, and a liquid fertilizer injection wheel is used for any later nitrogen and potassium applications. Fertilization for the second crop in a double cropping system is achieved by using a liquid injection wheel or the microirrigation system, if applicable (Hochmuth et al. 1997; Spreen et al. 1995; Hochmuth and Elmstrom 1992; Hochmuth 1992).
In nonmulched systems with seepage irrigation, the micronutrients, phosphorus, and approximately 15%–20% of the nitrogen and potassium is incorporated into the bed before planting. The rest of the nitrogen and potassium is applied later as a side-dress band along the outside of the bed. When using drip irrigation, growers incorporate the micronutrients, phosphorus, and approximately 20%–40% of the nitrogen and potassium into the bed before planting and add the remaining nitrogen and potassium through drip irrigation (Hochmuth et al. 1997; Spreen 1995; Hochmuth 1992).
All types of irrigation (overhead, drip, seepage) can be used in watermelon production but drip irrigation is most commonly used (Maynard 1996). In general, cucurbits have slightly lower water requirements than other vegetable crops (Olson et al. 2012).
Because watermelons are very sensitive to the cold, many growers in North and Central Florida plant windbreaks during the winter to protect springplanted watermelon seedlings from blowing sand and wind damage. The warming effect near the plant also helps achieve faster plant growth. Rye is the most common windbreak used, although sorghum and ryegrass are also effective. Sugarcane can also be used in South Florida. The windbreak is planted in strips, and on seepageirrigated fields, windbreaks can be created in irrigation ditch areas and roadways (Hochmuth and Elmstrom 1992).
Cucurbit plants have separate male and female flowers, requiring pollen to be transferred for fruit development to occur. At least 1,000 grains of pollen must be deposited evenly on the stigma of female flowers. If pollen is not evenly deposited, the resulting fruit will not be uniform. Honeybees are the principal pollinators, and growers usually rent hives to place in or around their fields to achieve adequate pollination. Although natural pollinators may be present, growers cannot always rely on them to provide sufficient pollination activity because they can be affected by weather conditions (Hochmuth et al. 1997; Johnson 1992).
Research on watermelon pollination in Florida has shown that female flowers require at least eight bee visitations for adequate fruit set to occur. Sufficient pollination generally requires one strong hive for every two acres or a bee population that provides at least one bee for every 100 flowers. Hive placement is one of the many factors that may affect pollination. Hives are often placed around the perimeter of large fields for adequate distribution. Standard pollination recommendations for watermelon production in Florida are not available, and growers must work with the beekeeper to determine the optimum number of colonies and placement for each field situation (Hochmuth et al. 1997; Johnson 1992). Seedless watermelon varieties tend to require slightly higher bee populations (Maynard 1996).
Honeybee activity is greatest an hour or two after sunrise until midafternoon, coinciding with the time flowers remain open and most amenable to setting fruit. Most watermelon pollination occurs from 7 a.m. to 11 a.m., with the greatest percentage of fruit set resulting from insect visits between 9 a.m. and 10 a.m. Honeybees are highly susceptible to most of the insecticides used on watermelons. To avoid adversely affecting the bee colonies, any pesticide applications during the flowering period should be made at dusk or later, when bees are not active. It is recommended you have a written contract that includes detailed expectations of both the grower and the beekeeper (Hochmuth et al. 1997; Johnson 1992; Sanford and Ellis 2011).
Seedless watermelon plants do not produce enough pollen for adequate fruit set and development, so they are interplanted with seeded pollenizer watermelon plants. Traditionally, the pollenizer variety was planted in the outside row and then in every third row. If producers choose to utilize dedicated rows for pollenizer plants, varieties that can be easily distinguished from seedless watermelons should be chosen. For pollen to be available to the seedless plants at the appropriate time, direct field seeding of the pollinizer variety usually occurs at the same time that the seedless variety is planted in the greenhouse. When icebox (smaller) varieties are used as the pollinizer, directseeding is delayed 7–10 days because icebox varieties flower earlier. To assure sufficient pollen for lateblooming seedless plants, some growers make a second planting of the pollinizer variety 2–3 weeks after the first planting (Maynard 1996).
Because seeded watermelons have such little market-share most producers no longer use dedicated rows for pollenizer plants. Many use varieties developed specifically for use as pollenizers that are not intended to be harvested. These varieties produce small fruit that tends to have solid light green exteriors with thin, soft rinds. These varieties are intended to be planted in-row between seedless plants at a ratio of one pollenizer to three seedless plants. In this system, pollenizers are transplanted at the same time as seedless plants. This system allows producers to plant a full population of seedless watermelon plants with no dedicated space for pollenizers. Specific pollenizer varieties include but are not limited to ‘Ace’, ‘Accomplice’, ‘Patron’, ‘Poliax’, ‘Wildcard’, ‘Wingman’, and ‘SP-6’.
Watermelons are harvested in Florida from April 1 to July 15, with the most active harvest period from May 1 to July 1. April and May's production is primarily from southwest and west-central Florida. Growers in Central Florida begin harvesting in mid to late May, while production from northcentral Florida enters the market in June (FDACS 2012; Hopkins 2003). The majority of Florida’s watermelon acreage is harvested during June (Hochmuth et al. 1997). The watermelons in a field will not ripen at the same time. The first harvest produces the greatest yield and quality, while later harvests cost more because the fruit is smaller and lower quality, which reduces yields. Also, once watermelon harvest begins in other states, the value of Florida’s crop declines significantly. Although yield is maximized by harvesting several times, many Florida growers, particularly in North Florida, only pick once or twice because of the low market prices of late harvested watermelons (Bertelsen et al. 1994).
Watermelons are harvested once they reach an acceptable level of maturity for the intended market. Key harvest indices include yellowing of the ground spot, drying of the tendril, and dulling of the fruit surface. Fruit pulp should be sufficiently sweet, and it develops simultaneously with the deep red flesh color, also a desirable characteristic (Rushing 2016). Some specialty watermelon varieties produce yellowfleshed fruits. If sold at local markets, watermelons are harvested when fully ripe; however, watermelons sold at distant markets must be harvested just before full ripeness to minimize damage from handling. Producers must take caution to avoid harvesting immature fruit. Immature fruit may be firm but will not develop an adequate level of sweetness (Rushing 2016). Unlike cantaloupes, watermelons will not continue to ripen after harvest.
Workers hand-harvest watermelons by cutting them from the vine. One person in the field crew walks the field to indicate which melons to pick, and then cutters (one or two per row) cut and turn the melon so the ground spot is apparent. Two or three pickers per row follow the cutters, and melons are handled approximately three times before being placed in a box or truck, where one or two stackers work. Damage sustained during harvesting and handling may produce cracking and lower fruit quality and appearance. Therefore, harvest crews carefully lay the watermelons in rows and load them into padded field trucks to transport them to a collection point or a packing facility. Fruit harvested early in the morning are more likely to experience bruising and splitting than those harvested later in the day. During harvest, watermelons must be shaded from direct sunlight to minimize heating and sunburn.
At the collection point, workers unload watermelons by hand from the field trucks. Then the watermelons are sized and graded, not usually washed, and reloaded in bulk using plastic or fiberboard pallet bins and placed onto highway trucks. Round and seedless types are often packed in corrugated cartons, 2–5 fruit each, or in pallet bins that hold approximately 1,100 pounds of fruit; many are shipped in bulk (Hochmuth et al. 1997; Sargent 1992). Fruit can also be sorted, sized, and loaded directly into bins in the field. Bulk shipments entail handling of watermelons at least five times from harvest until arrival at the retail store. Methods to reduce handling steps, such as shipping in field bins or cartons on pallets, are desirable to reduce bruising and improve overall quality. Packaged or binshipped watermelons typically fetch higher prices than bulkshipped watermelons, because they are less likely to have sustained damage from handling, and handling is facilitated at receivers’ docks (Hochmuth 1997; Rushing 2016; Sargent 1992).
Much of the watermelon crop harvested in Florida is shipped under ambient conditions, but some containerized watermelons shipped out of state may be held in cold rooms before shipment in refrigerated trailers. Temperatures of 50°F–60°F (10°C–15°C) and <90% relative humidity are recommended to maximize postharvest quality and shelf life. However, fruit should remain refrigerated during subsequent handling; removing fruit from refrigeration will cause condensation (sweating) to form on the fruit and rapidly promote decay.
Any fruit handled above the ideal temperature is more susceptible to decay and flesh breakdown (senescence). Exposure to ethylene gas at any point during handling, storage, or transport, will also accelerate senescence and must be avoided. Ethylene is released from certain fruits and vegetables and by internal combustion engines (Hochmuth et al. 1997; Rushing 2016; Sargent 1992). Chilling injury is another cause of quality loss, occurring during storage below 50°F (10°C). Symptoms include fading of redness, increased juice leakage, and decay. Maintaining relative humidity below 90% will reduce incidence of stem end rot. For best quality, watermelons should be consumed two to three weeks after harvest.
Pest Management
The most important pest groups in Florida watermelon production are disease pathogens, particularly viral diseases, and weeds. Disease pathogens are difficult to manage, and weeds have become more difficult to manage with the loss of methyl bromide. Insect pests are generally less damaging than on other cucurbits.
Watermelon producers have access to a great deal of information regarding the production of watermelons in Florida, as well as integrated pest management programs that have been developed by researchers throughout the state and promoted through UF/IFAS Extension.
Growers employ a variety of pest management techniques, particularly for disease management (Spreen et al. 1995).
Soil Fumigation
Soil fumigation is the injection of chemicals, generally in liquid form, into the soil that subsequently volatilizes, and fumigant vapor moves through the soil. Historically the soil fumigant primarily used was methyl bromide, but it was found to be an ozone-depleting compound and its’ use was phased out. The phase-out of methyl bromide is complete, and it is no longer available to producers for soil-borne pest management. Other fumigants are available but their efficacy is not as good on a broad spectrum of pests compared to methyl bromide. These fumigants must be combined to improve efficacy. Because of the specificity of available fumigants, producers must now use site-specific management based on pests and pathogens present in given fields. New application techniques must also be employed to maximize the efficacy of these fumigants. An extensive review of available fumigants, their efficacy, and application methodology can be found in the Vegetable Production Handbook of Florida (Paret et al. 2021).
Insect/Mite Management
Insect/Mite Pests
The principal insect and mite pests on watermelons in Florida are aphids, rindworms (caterpillars feeding on the rind, including beet and fall armyworms, cabbage looper, tobacco budworm, corn earworm, saltmarsh caterpillar, and others), whiteflies, and thrips. Occasional or minor arthropod pests include seedcorn maggots, cutworms, leafminers, cucumber beetles, mole crickets, wireworms, whitefringed beetle larvae, mites, and flea beetles. Leafhoppers and fleahoppers, grasshoppers, omnivorous leafrollers, plant bugs (including lygus bugs and stink bugs), and squash bugs may occasionally be seen on watermelons but are not economically damaging (Maynard 2003; Hopkins 2003; Webb 2017; Webb 1995; Webb et al.1994).
APHIDS
Aphids that probe or feed on watermelon include melon aphid (Aphis gossypii), green peach aphid (Myzus persicae), cowpea aphid (Aphis craccivora), spirea aphid (Aphis spiraecola), Aphis middletonii, and Uroleucon pseudambrosiae, among others. Aphids constitute the principal insect pest of watermelons in Florida, primarily because of their role in virus transmission. Melon aphid, green peach aphid, and cowpea aphid feed and reproduce on watermelons and other cucurbits. The other aphids listed are important virus vectors but do not colonize watermelons. Melon aphid is the most abundant aphid colonizing watermelon in Florida. It is a major pest of cucurbits and cotton and attacks many other plants, including eggplant, pepper, potato, citrus, okra, and a range of ornamentals and weed species (Webb, et al. 1994).
Aphids feed by piercing plant tissue with their needlelike mouthparts (stylets) and sucking out water and nutrients from the plant’s vascular system. Evidence of damage includes thickening, crumpling, and downward curling leaves caused by feeding damage and injury from toxins in the saliva injected into plant tissue during feeding. Heavy aphid attacks may kill very young plants. Aphids also deposit large amounts of honeydew on the plant surface, which encourages the growth of sooty mold. Aphid populations can increase rapidly in Florida because of a short life cycle and reproduction by live birth (Stansly 2011).
Aphids damage watermelon plants directly by feeding, as well as indirectly by transmitting viruses. Papaya ringspot virus type W, watermelon mosaic virus 2, and zucchini yellow mosaic virus can be transmitted by aphids that colonize watermelons and by several aphid species that do not reproduce on watermelons. These include A. middletonii, A. spiraecola (spirea aphid, also known as green citrus aphid), and U. pseudambrosiae (Webb 2003).
These viruses are transmitted by aphids in a styletborne, nonpersistent manner. This means that an aphid can pick up virus particles in its stylet from an infected plant and transfer them immediately to a healthy plant without the virus circulating through the aphid’s body. There is no delay from when the aphid acquires the virus to when it can transmit it, but the aphid can transmit the virus for only a short period. An aphid determines the suitability of a plant as a food source by probing (inserting its stylet into the plant’s tissue to test it). As a result, viruses can be transmitted by aphids that do not actually feed on the plant but only land on it momentarily to probe. Aphid vectors that do not feed on watermelons will move from plant to plant within a field, probing each one and spreading the virus. Aphids that do feed and reproduce on watermelons may also move from one plant to another in the field under crowded conditions when winged individuals are produced. Virus transmission to watermelon plants can occur within 10–15 seconds (Webb 2003).
RINDWORM COMPLEX
Any caterpillars that feed on watermelon rinds are called rindworms, including beet armyworm (Spodoptera exigua), fall armyworm (Spodoptera frugiperda), yellowstriped armyworm (Spodoptera ornithogalli), cabbage looper (Trichoplusia ni), tobacco budworm (Heliothis virescens), corn earworm (Helicoverpa zea), saltmarsh caterpillar (Estigmene acrea), and granulate cutworm (Feltia subterranea). Many rindworms also feed on the plant’s foliage and stems, but the greatest damage occurs from feeding on the rind, which directly reduces the fruit’s quality and marketability. Rindworms generally scar the surface of the fruit in irregular patterns, rather than boring into it. Larger caterpillars inflict greater damage, and management is generally easier when the caterpillars are smaller. Rindworms are often found on the underside or the least exposed parts of the watermelon (Webb 2003).
Beet armyworm and cabbage looper are presently the most abundant rindworms on watermelons in Florida. The cabbage looper has a wide host range. In addition to watermelons, cabbage looper also attacks cabbage and other crucifers, potato, spinach, tomato, cucumber, cotton, and soybeans. After overwintering in Florida and nearby states, it migrates north each year. Females lay their eggs on leaf surfaces of host plants, and each of the emerging larvae (caterpillars) feeds for 2–4 weeks, then spins a cocoon and pupates. Between 10 days and two weeks later, the adult moth emerges. Several generations occur each year in Florida (Webb 2003).
Beet armyworm is active throughout the year in South Florida, where many generations occur annually. A monitoring program has existed in South Florida for several years, and trap captures of beet armyworm moths are highest from late March through mid-June, corresponding to the later part of the leafy vegetable season in the region. During this time, the population rises and falls in cycles of 3–5 weeks. A smaller increase in the beet armyworm population generally occurs from mid-August through October and is likely related to the presence of late summer weeds (Nuessly and Hentz 1998). Beet armyworm moths migrate from South Florida to North Florida and other southeastern states each year. Females lay more than 600 eggs each, usually in groups of approximately 100. Eggs are laid on the underside of lower leaves. The larvae feed from 1 to 3 weeks, in groups when younger and scattered on the plant when larger. After pupating in a cocoon, the adult emerges after about one week (Webb 2003).
WHITEFLIES
Whiteflies found infesting Florida watermelons include silverleaf whitefly (Bemisia argentifolii) or sweet potato whitefly B biotype (Bemisia tabaci), and greenhouse whitefly (Trialeurodes vaporariorum). The silverleaf whitefly is the most prevalent in Florida. It was previously known as sweet potato whitefly B biotype, and it is a major pest of cucurbits and other vegetable crops in the state. The first US outbreak of the silverleaf whitefly was recorded in southcentral Florida in 1986, and its aggressive establishment and associated insecticide resistance have led to heavy infestations in the state's vegetable and ornamentals industries (Norman et al. 1993). With a host range of more than 500 species of plants, the silverleaf whitefly has been observed to reproduce on at least 15 crops and 20 weed species in Florida. Whitefly populations commonly peak on the state’s crops at harvest time, as the whitefly migrates from crop to crop throughout the year. In South Florida, populations build on fall vegetables and move directly to overlapping spring crops. In south central Florida, the whitefly uses cabbage and other winter crops to survive until the spring. During the summer fallow period, whitefly populations are low because whiteflies are limited to weeds, such as water primrose, hairy indigo, and spurge. Weeds are poor hosts to the whitefly and usually harbor many natural enemies that further reduce populations (McAuslane and Smith 2015; Norman et al.1993; Stansly 1995).
Whiteflies damage watermelon plants by removing plant sap, depleting the plant of needed nutrients. On some hosts, whiteflies cause severe damage by transmitting plant viruses. On watermelons, whiteflies have been reported to transmit squash leaf curl and lettuce infectious yellows, but these viruses do not presently occur in Florida. In Florida, the principal damage to watermelons inflicted by whiteflies is caused by feeding, which greatly reduces the plants’ vigor under heavy infestations (Webb 2003).
Adult females produce an average of 160 eggs each, depositing them on the lower surface of host plant leaves. The first nymphal (immature) stage, the crawler stage, attaches itself to the leaf near the empty egg case. The whitefly passes through three more sedentary nymphal stages, appearing like transparent scales, before molting to the adult stage. Whiteflies feed by sucking the plant’s sap through their needlelike piercingsucking mouthparts. Like aphids, they extract large amounts of the plant’s sap (phloem) and excrete the excess liquid as honeydew. Sooty mold can then grow on the honeydew (Webb 2003; Norman et al.1993; Johnson, Short, and Castner 2005).
Similar to aphids, whiteflies are found mainly on the lower leaf surface, and only the first nymphal stage and the adults can move, so control using insecticides is limited if complete coverage is not achieved. Alternating insecticides is important to minimize the development of resistance. Managing whiteflies requires a combination of tactics, with an emphasis on cultural control.
Since 2004, whitefly management has become a priority for watermelon production. One main reason for this was the identification of three viruses transmitted by whiteflies. These viruses are the Squash vein yellowing virus (SqVYV), Cucurbit leaf crumple virus (CuLCrV), and Cucurbit yellow stunting disorder virus (CYSDV) (Webb et al. 2015).
THRIPS
Thrips found on Florida watermelon include tobacco thrips (Frankliniella fusca) and melon thrips (Thrips palmi). Tobacco thrips is a problem on watermelons in Central and North Florida but is rarely found in South Florida. They damage watermelons primarily in the seedling stage, with little or no damage observed once plants begin to grow rapidly. Feeding mainly occurs in the terminal buds, producing scarred leaves with silvery or clear areas that look as if they were damaged by blowing sand. Crinkling of tissue and the appearance of chlorotic areas can also result. Heavy infestations can stunt watermelon plants (Webb 1995; Webb 2003).
Melon thrips is an important insect pest on watermelons in South Florida and is only found in that part of the state, where it is a relatively new pest. It was first reported in early 1991 in the Homestead area (Miami-Dade County). It has caused large economic losses in several vegetable crops, particularly snap bean, pepper, eggplant, cucumber, winter melon, and squash. In addition to those crops and watermelons, melon thrips also feeds on cantaloupe, potato, tobacco, soybean, broad bean, spinach, and amaranth. Both adult and immature thrips feed in groups on leaves, stems, flowers, and fruits, removing sap with their sucking mouthparts. Feeding by melon thrips on watermelons results in a bronzed appearance on leaves. However, the greatest damage occurs from feeding at the tips of vines, which restricts canopy development (Webb 2003; Tsai et al. 1995; Sakimura, Nakahara, and Denmark 1986; South 1991).
Melon thrips is present throughout the growing season in South Florida but is most abundant between December and April. The pest completes its life cycle in approximately 20 days at 86°F (30°C) and approximately 80 days at 59°F (15°C). Each female thrips produces an average of 50 eggs, which are deposited in slits the female makes in the leaf tissue. Larvae usually feed on older leaves, concentrating along the leaf midrib and veins. After passing through two larval instars in 4–5 days (at 79°F–90°F or 26°C–32°C), the larva drops to the ground, where it passes the prepupal and pupal stages in a soil chamber it has constructed. After 3–4 days (at 79°F–90°F), the adult emerges and seeks out new host plant leaves to feed on (Webb 2003).
The relationship between early damage from thrips and watermelon yield has not been determined. Melon thrips is resistant to many insecticides. In addition, the use of broadspectrum insecticides may increase populations of melon thrips by killing off natural enemies that may contribute to thrips management in the field. The use of insecticides also requires thorough spray coverage and is not effective against the egg and pupal stages, which are protected (Stansly 2011; Webb 2003; South 1991).
SEEDCORN MAGGOT
Seedcorn maggot (Delia platura) often reduces plant stand in Central Florida by attacking young emerging seedlings. Seedcorn maggot adults are small flies that become active early in the spring, laying their eggs where organic matter is high, such as in manure or where cover crops have been plowed under. They also lay eggs at the base of transplants. Upon hatching, the maggots enter the seed or the stem of the developing seedling. Injury from seedcorn maggot feeding results in wilting and plant death. Maggots feed on organic matter for 2–3 weeks, passing through three larval stages (instars) during that time. As the weather warms, the adult flies seek wooded areas, where the insect over summers in the pupal stage. The flies are most active during cool, wet springs. Transplanting or seeding into soils with temperatures of at least 72°F (21.3°C) at a depth of 4 inches (10 cm) will reduce the likelihood of damage from seedcorn maggot (Webb 2003).
CUTWORMS
Cutworms, including granulate cutworm (Feltia subterranea) and others, are stout caterpillars with a dull, greasy appearance. Several cutworms, particularly granulate cutworms, can attack watermelons in Florida (Webb 2017). Cutworms are exclusively a problem for young watermelon seedlings. They are active at night but retreat to the soil or under surface litter during the day. They often cut the main stem of the seedling at the base and can greatly reduce the stands of newly emerged watermelon plants. Cutworms can also climb the plant stem to feed on foliage. Being more prevalent in spring and fall, they overwinter in the larval stage, often in grasslands. Baits applied for cutworm control should be laid out in the afternoon or early evening for maximum effectiveness (Webb 2003).
LEAFMINERS
Leafminers (Liriomyza sativae and Liriomyza trifolii) are occasionally a major pest of watermelons in South Florida. The adult fly punctures the upper leaf surface while feeding, and the female inserts eggs into the puncture holes. When each larva (maggot) emerges, it feeds between the upper and lower leaf surfaces, creating a tunnel or “mine” that winds across the leaf and becomes larger as the maggot grows. When larval development is complete, after about two weeks, the maggot cuts through the leaf surface and emerges from its mine, dropping to the soil to pupate. Where watermelons are grown on plastic mulched beds, leafminer pupae can be seen on the surface of the plastic. Leafminer populations can increase rapidly in Florida, where the life cycle can be as short as 18–21 days (Webb 2003).
Infestations are often more severe late in the season. Under heavy infestations, leaves may be nearly covered with mines, and defoliation late in the season can result in sunscald damage on the fruit. Adequately managing leafminer populations in watermelon seedlings is particularly important because the mines can serve as entry points for the fungus that causes gummy stem blight, an important watermelon disease in Florida (Hochmuth and Elmstrom 1992; Webb 2003).
CUCUMBER BEETLES
In Florida, cucumber beetles include banded cucumber beetle (Diabrotica balteata), striped cucumber beetle (Acalymma vittata), and spotted cucumber beetle (D. undecimpunctata howardi). These beetles occasionally feed on watermelons in Florida to the degree that control measures become necessary. Banded cucumber beetle is more prevalent in South Florida, while spotted cucumber beetle is most common in North Florida. The striped cucumber beetle is seen only occasionally, primarily in western and northern Florida (Watson and Tissot 1942).
Cucumber beetles prefer to feed on cucurbit plants such as squash, cucumber, and cantaloupe in addition to watermelon, but they can also feed on a wide range of crops, including corn, beet, pea, sweet potato, okra, lettuce, onion, cabbage, potato, and tomato. Spotted cucumber beetle, with larvae known as southern corn rootworm, is the most general feeder of the three, having been recorded on more than 200 species of crops, grasses, and weeds. Feeding damage from adult cucumber beetles results in ragged holes in the leaves, and the beetles may also feed on stems. The larvae are found in the soil and feed on the roots of the vines and the underside of the watermelon fruits laying on the soil. These beetles have the greatest effect on watermelon yield when plants are attacked at the seedling stage. Older plants can withstand higher amounts of feeding damage. When cucumber beetles are found on 10% of seedlings, control measures should be instituted (Watson and Tissot 1942; Capinera 2011; White 1964).
Cucumber beetles have many generations each year. The life cycle of the banded cucumber beetle may be as short as 45 days under optimal conditions. During a two to eight-week period, each female banded cucumber beetle deposits 2–15 clusters of up to 100 eggs in soil cracks, with each female depositing up to 850 eggs. Striped cucumber beetle females lay approximately 100 eggs each, at the base of host plants. The larvae hatch after 5–9 days. Banded cucumber beetles pass through three instars in 11–17 days (somewhat longer for striped and spotted cucumber beetles), during which time the larvae feed on roots and tunnel through stems of host plants, and pupation occurs in the soil. Adult banded cucumber beetles emerge after 4–6 days. Approximately 16 days after adults emerge, females begin to lay eggs. Adults live for 17–44 days, with an average longevity of 26 days (Watson and Tissot 1942; Capinera 2011).
MOLE CRICKETS
Mole crickets (Scapteriscus spp.) occasionally present a problem to watermelon producers in Florida. They damage young seedlings by feeding on and tunneling in and around the roots often girdling the stems at their base. Their presence can be confirmed easily by the tunnels they make just below the soil surface. Mole crickets are active at night, particularly on warm nights when soil is moist, and they are rarely seen during the day (Schuster and Price 1992). Mole crickets overwinter as adults or older nymphs, often a foot or more below the soil surface. Adults deposit their eggs in chambers they make in the soil, usually 4–12 inches below the surface. Nymphs hatch after three weeks, and they feed for many weeks before reaching the adult stage. Although mole crickets may attack many vegetable crops, they prefer pasture and grass, where large populations are generally found in Florida. Mole crickets are more likely to be a problem if pastureland is rotated to watermelons, a common practice in North Florida (Schuster and Price 1992; Frank, Fasulo, and Short 1998).
WIREWORMS
Wireworms (Conoderus spp.), the larvae of click beetles, are among the most destructive of soil insect pests. Conoderus spp. wireworms, including C. rudis, C. amplicollis (Gulf wireworm), C. falli (southern potato wireworm), and C. verpertinus (tobacco wireworm), are the most common on vegetable crops in Florida. Wireworms cause the greatest damage to germinating seeds, and transplants are generally less susceptible. In addition to attacking seeds before or at germination, wireworms can bore into the taproot and tunnel up the stem or feed on smaller roots of seedlings. They can cause sudden stand reductions by quickly attacking young seedlings, causing wilting, stunting, and death. Click beetles lay their eggs in the soil near plant roots, and on hatching, the wireworms feed on the nearby roots. Wireworms may spend up to several years in the soil in the larval stage and may be present at a soil depth of 1–5 feet (0.3–1.5 meters) (Stansly 2011).
Similar to mole crickets, wireworms are more numerous in pasture and grass, so watermelons planted to land previously used for pasture are likely to experience greater wireworm problems. Chemical controls for wireworms must be applied before or at planting.
WHITEFRINGED BEETLE
Whitefringed beetle (Graphognathus spp.) is an occasional pest on watermelons and other crops in North Florida. It prefers cotton, peanut, okra, soybean, cowpea, sweet potato, beans, and peas, but most vegetable and field crops are attacked by this pest. It has a host range of more than 385 species. Larvae (grubs) feed on roots, tubers, underground stems, and dead plant matter, and can inflict serious damage to the taproot by boring into it. In addition to directly killing the seedling, grub damage to roots leaves the plant susceptible to root infections. Whitefringed beetle grubs may damage plants in only a few scattered areas within a field or may affect nearly all plants within a field.
Adults feed on leaves of host plants, cutting out notches along the leaf margins. They lay eggs in the soil at the plant base, and the grub stage can live up to two years in the soil. Currently, no insecticides are effective against the grub, although several insecticides are available for adult whitefringed beetles. The presence of whitefringed beetle grubs is best determined by the crop history of the land. Watermelons should not be planted to land infested with whitefringed beetle grubs (Dixon 1988).
SPIDER MITES
Spider mites, the most common type of mite affecting crop plants, are occasional pests on watermelons in Florida. They feed on the underside of leaves, primarily along the midribs and lateral veins. After piercing the leaf surface, they suck up the plant’s sap. Mite feeding causes watermelon plants to turn pale, then yellow, and eventually brown, and the plant appears dusty. Under severe infestations, foliage dries up and dies. When many mites are present, silken webs and white flakes of molted skin can be observed on the leaf surface. Mites move from one plant to another by parachuting through the air on their webs, using the wind. When their path is interrupted by an obstacle, they crawl down and seek out host plants. Infestations begin along edges of fields and close to high objects such as fences or trees.
Before using chemical controls, you should determine the age structure of the insect population under Florida’s warm conditions. When many nymphs are present, the population is probably increasing, whereas if many adults are present, particularly males, it is probably declining. In addition, if a miticide is used, a second application is necessary in Florida 5–7 days following the first. The second application kills the mites that escaped the first application because they were in the egg stage. Timing is critical to prevent those mites from maturing and laying eggs (Stansly 2011).
Chemical Control
In 2014, insecticides/miticides were applied to 73% of the state’s watermelon acreage with total 18,800 pounds of active ingredient. Specific insecticides usage data is available through the National Agriculture Statistical Service. Insecticides labeled for use on Florida’s watermelon include (S)-Methoprene, 1,3-dichloropropene, abamectin, acequinocyl, acetamiprid, allyl isothiocyanate, alpha, cypermethrin, ammonium nonanoate, avermectin B1, azadirachtin, beta-cyfluthrin, bifenazate, bifenthrin buprofezin, Capsicum oleoresin extract, carbaryl, chlorantraniliprole, chloropicrin, clothianidin, cryolite, cyantraniliprole, cyfluthrin, cyromazine, deltamethrin, diatomaceous earth, diazinon, dimethoate, dinotefuran, esfenvalerate, etoxazole, extract of Chenopodium ambrosioides, fenpropathrin, fenpyroximate, flonicamid, flubendiamide, fluensulfone, fluopyram, flupyradifurone gamma-cyhalothrin, hydramethylnon, iron phosphate imidaclopridindole-3-butyric acid, indoxacarb, kaolin, lambdacyhalothrin, malathion, methomyl, methoxyfenozide, novaluron, oxamyl, oxydemeton-methyl, permethrin, pheromone (Z)-11-Hexadecen-1-yl acetate; pheromone (Z,E)-9,12-Tetradecadien-1-yl Acetate, piperonyl butoxide, potassium salts of fatty acids, pymetrozine, pyrethrins, pyriproxyfen, saponins of Quillaja saponaria, sodium tetraborohydrate decahydrate, spinetoram, Spinosad, spiromesifen, sulfur, thiamethoxam, zeta-cypermethrin, oils (clove, cotton seed, garlic, soybean, sesame, peppermint, rosemary, thyme, neem, mineral, paraffinic, petroleum, Bacillus thuringiensis, Beauveria bassiana Strain GHA, Burkholderia spp. strain A396, Chromobacterium subtsugae strain PRAA4-It and spent fermentation media, Metarhizium anisopliae Strain F52, Myrothecium verrucaria strain AARC-0255 and Paecilomyces sp., and Ulocladium oudemansii (U3 Strain) (USDA/NASS 2021; CDMS 2017).
ESFENVALERATE
Esfenvalerate is a broadspectrum, synthetic pyrethroid insecticide used on watermelons in Florida. During most years, it is primarily used to manage cutworms, corn earworms, and cabbage looper. It also helps in managing cucumber beetle adults, leafhoppers, grasshoppers, plant bugs (including lygus bugs and stink bugs), squash bugs, and squash vine borers (Webb 2017). Esfenvalerate may be applied up to 3 days before harvest (preharvest interval = 3 days), and the restrictedentry interval (REI) under the Worker Protection Standard (WPS) is 12 hours. A maximum of 0.25 pounds of active ingredient may be applied per acre during each season (CDMS 2017).
Cultural Control
Planting early is one of the most effective cultural controls for most insect pests of watermelons. Early production is an important goal of Florida watermelon growers because of market considerations, so this practice is common. Early planting even for the fall crop is useful because aphid populations tend to decline in midsummer and do not build again until later in the season (Webb 2017).
To reduce aphid populations and the resulting spread of aphidvectored viruses, watermelons planted during the summer for a fall harvest should be planted far from fields in cucurbit production the previous spring. In addition, weeds in and around the field that may harbor aphids should be eliminated. Using mineral oil (stylet oil) can disrupt the transmission of nonpersistent viruses by interfering with the transfer of virus particles to and from aphid mouthparts. Oil sprays on watermelons in Florida applied at four to fiveday intervals beginning early in the season have been shown to delay primary infection by slowing the start of viral epidemics in the crop and reducing viral spread. Stylet oil is most effective when virus incidence is low (less than 20%). Low virus incidence occurs under conditions of limited inoculum, so stylet oil is most effective for springplanted watermelons in Florida. Economic analysis has shown that the cost of 12–15 applications of stylet oil during the season is offset if the oil increases yield by 5%–7% (Hochmuth and Elmstrom 1992; Webb and Linda 1993).
Producers have also tried using white mulch or reflective mulch (usually black plastic painted with aluminum) to repel aphids by preventing them from landing on the watermelon plants. However, reflective mulches only had limited effectiveness, particularly as the season progresses and the plant foliage covers the mulch. In addition, white or reflective mulches delay warming of the soil in the early spring, a key concern for Florida watermelon producers (Webb 2017).
Aphids, whiteflies, and thrips populations are all affected by surrounding crops and vegetation, and properly managing weeds and crop residues is an important tactic. Destroying crop residues in and around fields can also prevent the movement of leafminers, which particularly damage lateplanted watermelon fields. In addition to destroying crop residues and avoiding planting near infested crops, cultural controls for whiteflies include early planting, rotating with nonsusceptible crops, using reflective mulches, and using physical barriers such as floating row covers (Webb 2003; Norman et al. 1993).
Florida vegetable growers commonly turn the soil several times to expose wireworms to sunlight. Deep plowing at least 30 days before planting can reduce all populations of soil insects by exposing the insects to bird predation and harsh environmental conditions. Deep plowing also denies soil insects the grass and weed sources that serve as alternate food. Whitefringed beetle grubs can best be managed by planting infested land to grass or grains for extended periods (Webb 2003).
Biological Control
Aphid predators (larvae of ladybird beetles, syrphid flies, and lacewings) and parasitic wasps have been observed to reduce aphid populations in north-central Florida later in the season. However, aphid natural enemies are not present in great enough numbers during the early part of the season to effectively manage aphid populations as a control measure by themselves (Webb 1996).
Several predators, pathogens, and parasitic insects naturally attack rindworm complex caterpillars in Florida. For example, cabbage loopers have been found to be infected with nuclear polyhedrosis virus and attacked by several parasitic insects, including wasps and tachinid flies. A protozoan (Microsporidium sp.) has been observed to attack granulate cutworm, and fungal pathogens and nuclear polyhedrosis virus both attack beet armyworm (Webb 2003).
Leafminer populations in watermelons are kept down by several parasites. However, natural enemies are susceptible to toxic pesticides used on the crop (Webb 2003). In the absence of broadspectrum insecticides, several types of organisms naturally manage whitefly populations. Predators include green lacewing larvae and ladybird beetles. Whiteflies are also killed by parasitic wasps, such as Encarsia spp. and Eretmocerus spp., as well as by disease-causing fungi, such as Beauveria, Paecilomyces, and Verticillium. Insect pathogens for managing silverleaf whitefly are currently being studied under field conditions, but commercial formulations are not yet available (Norman et al. 1993).
Several natural control agents of melon thrips have been evaluated for their potential to manage the pest in Florida, including the eulophid wasp (Ceranisus menes) and the predatory mite (Neoseiulus cucumeris). But research has not been completed on the effectiveness of these and other natural enemies in managing thrips on watermelon (Castineiras, Baranowski, and Glenn 1996; Castineiras, Baranowski, and Glenn 1997). Several fungal pathogens are also known to infect melon thrips, including Neozygites parvispora, Verticillium lecanii, Hirsutella sp., Beauveria bassiana, and Paecilomyces fumosoroseus. Preliminary studies of Beauveria bassiana and Paecilomyces fumosoroseus in Florida show potential for thrips management, but more research is needed to determine the effect of these fungi on thrips populations under field conditions (Castineiras et al. 1996).
Research efforts are underway for several biological controls of mole crickets because of the severe damage to Florida turfgrass, pasture grass, and vegetables. Presently, the most effective biological control agent for mole crickets is a steinernematid nematode. The nematode was first brought to Florida from Uruguay in 1985 and identified as Steinernema scapterisci (Nguyen and Smart 1990). This parasitic nematode has shown promise for managing mole crickets in pasture and turf in Florida, and is available commercially for mole cricket control in turf. It has been shown to be highly effective against tawny mole crickets and less effective against shortwinged mole crickets. It is most effective as a biocontrol agent in areas where mole cricket populations are highest, such as in pastures. The nematode can also be applied as a biopesticide in areas where mole cricket populations are lower, and it shows residual activity. The nematode disperses well when applied and has been recovered from infected mole crickets years after its application.
One reason it is effective is that mole crickets in North Florida have only one generation each year, while, under appropriate conditions, the nematode could produce a new generation every 10 days during eight months of the year. Populations of the nematode have become established in small areas of several Florida counties. If it becomes established in pastures surrounding vegetable crop production areas, it is expected to keep mole cricket populations below damaging levels (Frank, Fasulo, and Short 1998; Frank 1994).
Disease Management
Diseases/Pathogens
Diseases are a serious pest problem on watermelons in Florida. The state’s warm, moist climate, as well as the overlapping progression of watermelon plantings during the season from South Florida to the northernmost regions, creates conditions ideal for disease development. The incidence of the various diseases affecting watermelons in Florida varies by region, given the climatic differences in the state. The intensity of management efforts also varies by region. South Florida growers generally experience more consistent disease, but earlier harvests in that region provide higher market prices, generally creating an economically viable situation to aggressively manage diseases (Roberts and Kucharek 2005).
Three potyviruses that infect watermelon (papaya ringspot virus, watermelon mosaic virus 2, and zucchini yellow mosaic virus) occur annually in Florida. The viruses are spread by aphid vectors from infected watermelon plants or weeds to healthy watermelon plants. Because the aphids acquire and spread the viruses in a nonpersistent manner, insecticidal control is not effective. Generally, growers have observed spray efforts to be futile and instead concentrate their management efforts on other tactics. Recently, the whitefly transmitted viruses (squash vein yellowing, cucurbit leaf crumple virus, and cucurbit yellow stunting disorder virus) have been observed annually in Florida’s watermelon production areas. These viruses have become of particular importance since the identification of the insecticide-resistant B. tabaci B biotype whitefly. However, even without the resistant biotype, it can be difficult to manage whiteflies in watermelon. Cucurbit viruses are among the most difficult of Florida’s pests to manage. Florida watermelon crop loss as a result of viruses varies each year and by place. For example, little damage occurred from viruses in 1998 as a result of their late arrival that year, but in any year, fields with losses of 50%–100% can be common (Roberts and Kucharek 2005).
The most important fungal diseases are gummy stem blight (caused by Stagonosporopsis spp.), downy mildew (caused by Pseudoperonospora cubensis), Fusarium wilt (caused by Fusarium oxysporum f. sp. niveum), and powdery mildew (caused by Erysiphe cichoracearum). Other known diseases include phytophthora blight (caused by Phytophthora capsici), bacterial fruit blotch (caused by Acidovorax avenae subsp. citrulli), alternaria leafspot (caused by Alternaria cucumerina), seedling blight (caused by Pythium spp., Rhizoctonia solani, and Fusarium spp.), angular leafspot (caused by Pseudomonas syringae), anthracnose (caused by Colletotrichum orbiculare), and rind necrosis (usually caused by Erwinia spp.). Blossom end rot, a physiological disorder related to calcium deficiency and water stress, is also an occasional problem. Finally, Cercospora leafspot (caused by Cercospora citrullina) and southern blight (also called southern stem rot or white mold and caused by Sclerotium rolfsii) are only rarely seen on watermelons in Florida (Hochmuth et al. 1997; Maynard 2003; Roberts and Kucharek 2005).
PAPAYA RINGSPOT VIRUS TYPE W
Formerly referred to as watermelon mosaic virus 1, papaya ringspot virus type W (PRSVW) is a greater problem in South Florida, where it can severely damage the crop. Epidemics of the papaya ringspot virus have been linked to weeds as the primary source of inoculum (Roberts and Kucharek 2005). Papaya ringspot virus has been shown to overwinter in wild cucurbits such as wild balsam apple and creeping cucumber, and it is spread from these to springplanted watermelons in South Florida. During most years, the virus reaches Central and North Florida in early summer (Webb and Linda 1993).
WATERMELON MOSAIC VIRUS 2
Watermelon mosaic virus 2 (WMV2) is another potyvirus that causes regular problems for watermelon production, particularly in Central and North Florida during spring production. The incidence of watermelon mosaic virus 2 in Central Florida rarely exceeded 5% during the 1960s and 1970s. However, in the late 1980s, the virus caused severe losses to the spring watermelon crop in Central and North Florida, with an incidence in fields of up to 100%. Infection early in the season results in yield loss and reduced fruit quality because of blemishes, particularly rings and spots on the watermelon rind. However, if the virus does not enter a field until the time of fruit set, little to no yield difference is likely (Webb et al. 1994; Webb and Linda 1993).
The virus is transmitted in a nonpersistent manner by numerous species of aphids, including Myzus persicae, Aphis spiraecola, Aphis middletonii, Aphis illinoisensis, and Uroleucon pseudambrosiae (Webb and KokYokomi 1993; Adlerz 1978; Adlerz 1987). During the summer and fall, watermelon mosaic virus 2 builds up in many cucurbit and leguminous summer annuals, including hairy indigo (Indigofera hirsuta), showy crotalaria (Crotalaria spectabilis), alyceclover (Alysicarpus vaginalis), and Florida beggarweed (Desmodium tortuosum).
These hosts serve as sources of inoculum for the fall watermelon crop, and their presence is probably the reason that fewer aphids are necessary to initiate virus epidemics on the fall crop than on the spring crop. The source of inoculum for the spring watermelon crop has not yet been identified. The major factor in the appearance of spring epidemics of WMV2 in Florida is a secondary spread of the virus because primary infections in watermelon fields are limited (Webb and Linda 1993).
ZUCCHINI YELLOW MOSAIC VIRUS
Zucchini yellow mosaic virus (ZYMV), the third potyvirus affecting watermelon production in the state, was first observed in Florida in 1981 and identified in 1984. At that time, researchers demonstrated that it caused mosaic symptoms in watermelons and other cucurbits and could be transmitted by the aphids Myzus persicae and Aphis spiraecola (Purcifull et al. 1984). The incidence of zucchini yellow mosaic virus is generally lower than the other two potyviruses that affect watermelons. In Central Florida, the zucchini yellow mosaic virus is most common in late spring and fall. The virus causes more severe symptoms than the watermelon mosaic virus 2, with resulting discoloration and distortion of fruits (Webb and Linda 1993).
GUMMY STEM BLIGHT
Gummy stem blight (caused by Stagonosporopsis spp. previously known as Didymella bryoniae/Phoma cucurbitacearum) is one of the most important diseases of watermelons in Florida. It can infect all aboveground parts of the watermelon plant. Symptoms may be difficult to distinguish from other foliar diseases and include brown to black leaf spots, stem cankers, or fruit spots. Lesions eventually develop black spots, which are the fruiting bodies of the fungus (pycnidia). On watermelon fruit, the disease is referred to as black rot. It produces watersoaked spots on the fruit, which leak gummy material (Maynard and Hopkins 1999; Newark et al. 2014; Roberts and Kucharek 2005).
The fungus that causes gummy stem blight can be found on crop debris, in the air (as airborne spores), in and on contaminated seed, on volunteer and wild cucurbits, and on transplants. The principal source of inoculum for the disease is infected plant material from transplants and other cucurbit plants. Airborne spores (ascospores) are important in the field-to-field spread of the fungus, and they have been detected throughout the year in Florida, with a peak during June and July. The conidiospores, produced in the pycnidia, spread the pathogen from plant to plant within a field, principally through splashing rain. At least one hour of free moisture on leaves is required for infection to occur, and lesions continue to expand only when leaves are wet. Disease development is most rapid when frequent rains occur (Maynard and Hopkins 1999; Newark et al. 2014; Roberts and Kucharek 2005).
DOWNY MILDEW
Downy mildew (caused by Pseudoperonospora cubensis) is another key fungal disease of watermelons in Florida. It occurs principally in South Florida, and its likelihood of occurrence in the state decreases with distance to the north. It occurs every year in South Florida, while in North Florida its presence tends to be more sporadic. Ideal conditions for the development of downy mildew include nighttime temperatures of 55°F– 75°F (12.8°C–23.9°C) and relative humidity above 90%. It is possible for watermelons planted in the fall and spring may be infected with downy mildew as early as the appearance of the first true leaves if conditions are right (Newark et al. 2016; Roberts and Kucharek 2005).
Downy mildew attacks foliage, producing lesions on the leaves that can cause the plants to wilt. The lesions can kill them if they are severely infected early in their development. Initial symptoms include small yellow spots, which may be angular, grow to onehalf inch (1.3 cm) or more in diameter, and turn brownish. Leaves of watermelon plants may curl upward dramatically as a result of the lesions. The disease can reduce yield and lower fruit quality. The principal source of inoculum in South Florida is probably volunteer watermelon plants. Spores are produced mainly on the underside of the leaves or in the lesions and are dispersed by wind to other plants, primarily from late morning to midday. In the presence of moisture, spores that land on a leaf germinate and enter the leaf tissue. New lesions are produced in 4–7 days, and downy mildew spreads rapidly if not controlled (Newark et al. 2016; Roberts and Kucharek 2005)).
FUSARIUM WILT
The fungus responsible for Fusarium wilt of watermelons is soilborne and is widespread in many fields. Fusarium wilt (caused by Fusarium oxysporum f.sp. niveum) occurs commonly in Florida especially in the northcentral region and is composed of four races (0,1,2 and 3). The disease causes wilting and decline, which may occur in entire plants or in individual runners. Most commonly, the entire plant wilts quickly without yellowing and then turns brown and dies. A longitudinal cut in the lower stem of infected plants will reveal yellow, orange, or brownish streaks in the vascular tissue. In Florida, the disease usually occurs before fruit set. Infection rarely occurs at temperatures above 86°F (30°C), and optimum temperature for infection is about 80°F (27°C) but these values can vary between populations of the pathogen in the state (Roberts and Kucharek 2005; Kucharek et al. 1992).
Resistance to Fusarium wilt is only available for races 0, 1, and 2. However, a very limited number of varieties have resistance to race 2. There have also been indications that race 3 is present in Florida production areas as well. Long rotations of 5 to 10 years generally with a grass crop are required to manage Fusarium wilt, even when using resistant varieties are used (Hopkins, Lobinske, and Larkin 1992). Fungicides, such as prothioconazole, can be used to limit infections of the fungus, but results are highly inconsistent as the spatial distribution and development of the disease are often sporadic in many fields. Currently, the best management techniques for Fusarium wilt are the use of soil fumigants and grafting to resistant rootstocks integrated with resistant varieties and crop rotations. However, both techniques are relatively expensive and further work is being done to improve the incorporation of these techniques into current management strategies.
Some unique ways to reduce Fusarium wilt in Florida watermelon include maintaining soil pH between 6.5 and 7.5 by liming, using nitrate rather than ammonium forms of nitrogen fertilizer, using healthy transplants, removing or turning under crop residues after harvest, and delayed thinning (Bertelsen et al. 1994; Hochmuth et al. 1997; Spreen et al. 1995; Mitchell, Martin, and Charudattan 1994).
POWDERY MILDEW
Powdery mildew (caused by Podosphaera xnathii or Erysiphe cichoracearum) is a common and serious disease of watermelons in Florida. Since no resistant cultivars are available for this disease, fungicides are the primary means for management. Typical symptoms are the production of white, powder-like colonies on the leaf surface which are found on the leaves and petioles. Yellow spots can also be noticed on the leaf, which can be confused with other diseases, such as, downy mildew or bacterial leaf spots. In severe cases, the fungus will colonize the whole leaf, leading to death.
Dry conditions, usually followed by periods of free moisture at night, tend to promote the reproduction and spread of this pathogen. Spores are produced in chains on the leaf surface and are removed from the leaf by the wind. These spores then infect watermelon leaves and the cycle repeats multiple times throughout the season. The pathogen can develop and spread quickly under favorable conditions with symptoms appearing three days after infection.
Fungicides have been proven to save yields when powdery mildew is present. However, this pathogen is a high risk for fungicide resistance to develop within a population, thus rotation of products for this disease is critical (Dufault and Paret 2017).
PHYTOPHTHORA BLIGHT
Phytophthora blight (caused by Phytophthora capsici) occurs sporadically in Florida, but the disease can spread rapidly if the weather is favorable to the fungus, causing serious losses. In 1998, the disease was widespread and severe on several vegetable crops in Florida. In the southwest region of the state (Lee, Collier, and Hendry Counties), 25% of watermelon plants in some surveyed fields were found to have the disease, and disease incidence on watermelons in Manatee County was 36%. Previously, phytophthora blight had affected only the fruit of watermelons, but during the 1998 outbreak, many watermelon plants were affected and died in Manatee County, regardless of plant age. In untreated test plots at the UF/IFAS Southwest Research and Education Center in Immokalee, 100% plant mortality occurred on watermelon plants of the variety ‘Regency’ (Roberts and Kucharek 2018; Roberts and McGovern 1998).
The fungus causes seed rot and seedling blight (dampingoff). Seedlings may become discolored at the base and fall over. Under moist conditions, white fungal growth can be observed. In mature watermelon plants, foliar symptoms appear as watersoaked (greasy looking) blotches, which later dry out and turn brown. Some runners may experience dieback. Although such symptoms may appear under highly favorable conditions, as occurred in 1998, phytophthora blight primarily produces a fruit rot on watermelons. Irregular brown lesions on the fruit expand rapidly to round or oval lesions that may contain concentric rings. The fungal body (mycelium) appears as a cottony, white or gray growth in the center of the rotted tissue, surrounded by brown, watersoaked areas. The disease causes the entire fruit to eventually decay (Roberts and Kucharek 2018; Roberts and McGovern 1998).
Phytophthora capsici spreads from plant to plant by wind or water in the form of swimming spores (zoospores), which require sufficient surface moisture to infect host tissue. Disease development is most rapid during warm, wet weather (75°F–90°F or 24°C–32°C) and in low, waterlogged areas of the field or during excessive rainfall. During the 1998 epidemic, disease development continued rapidly even after rainfall ended, suggesting that moisture on leaf surfaces from dew and fog is sufficient for the spread of spores. When ideal conditions are present, symptoms of phytophthora blight may be observed in 3–4 days after infection. The fungus can survive on seed and in the soil on host plant debris in the form of thickwalled spores (oospore). These survival structures can survive in the soil for at least two years and serve as the source of inoculum for later crops (Maynard and Hopkins 1999; Roberts and Kucharek 2018; Roberts and McGovern 1998).
BACTERIAL FRUIT BLOTCH
Bacterial fruit blotch (caused by Acidovorax avenae subsp. citrulli) occurs sporadically, affecting only a limited number of fields, but it can cause severe losses where it occurs. Also called greasy fruit spot or watermelon fruit blotch, this bacterial disease is a relatively new problem in Florida watermelon production. In 1989, the first year it was observed in the state, losses of 50%–90% of marketable fruit occurred in some fields. When an outbreak occurs early in the season, no marketable fruit may be harvested (Roberts and Kucharek; Somodi et al. 1991; Hopkins, Cucuzza, and Watterson 1996; Latin and Hopkins 1995).
The pathogen produces both a leafspot and a fruit spot, and symptoms can occur on seedlings, leaves, and fruit. When it attacks seedlings, watersoaked lesions form, and the seedling can collapse and die. Found principally along the major veins, lesions on the leaves are light to reddish-brown and can occur throughout the season. Although they are generally inconspicuous and do not usually contribute to defoliation, leaf lesions serve as reservoirs of the bacteria that later infect the fruit. On the watermelon fruit, lesions start as very small, watersoaked areas that usually do not appear until close to harvest. Later, they enlarge, and within two weeks, they may cover the entire upper surface of the fruit. Cracks may later appear on the rind, which may show internal discoloration, and the whole fruit may rot within, as secondary pathogens enter the open lesions (Roberts and Kucharek 2005; Somodi et al. 1991; Hopkins, Cucuzza, and Watterson 1996; Hopkins 1991; Frankle, Hopkins, and Stall 1993).
Bacterial fruit blotch is transmitted by seed, the principal source of epidemics in Florida. In addition to infected seeds, the disease can enter watermelon fields through infected transplants, infected volunteer watermelons, and possibly from infected wild cucurbit plants. For example, wild citron, a common weed throughout the southeastern United States, is highly susceptible to the disease. Conditions in transplant production facilities (such as the warm, humid environment and the use of overhead irrigation that can splash bacteria to nearby seedlings) can contribute to a high level of secondary spread. In the field, the pathogen spreads among plants and from leaf lesions to watermelon fruit. Seeds from infected fruit that rot in the field can fall to the soil, producing infected volunteer plants that are a source of local inoculum during the next season (Latin and Hopkins 1995; Hopkins 1991).
ALTERNARIA LEAF SPOT
Alternaria leaf spot (caused by Alternaria cucumerina) is a minor disease in Florida watermelons. Symptoms begin on the upper surface of older leaves as very small yellow or tan spots that may be surrounded by light green or yellow halos or by a watersoaked area. The spots later grow up to ¾ of an inch (2 cm) in diameter and turn brown. Similar in appearance to gummy stem blight, the lesions are the source of spores spread primarily by the wind. Under severe infestations, the disease produces leaf curling, defoliation (which leaves the fruit susceptible to sunscald), and premature ripening. Lower yields, lower fruit sugar, and fruit deformity may occur (Roberts and Kucharek 2005).
The fungus can survive on or in crop debris, with debris on the surface more likely to spread spores because of exposure to the wind. Volunteer cucurbit plants and weeds, such as balsam apple, are also sources of inoculum. When watermelons are planted successively for multiple harvest dates, older infested plants located upwind can also contribute to disease spread. Although wind is the main vehicle for spore dispersal, movement by rain splash and mechanical means can also occur. As in other fungal diseases, spores require moisture to germinate and enter the leaf tissue, while spore release from the plant is best achieved under dry conditions. The optimum temperature for infection is 68°F (20°C), and in 3–12 days from spore penetration, the next group of spores is released.
SEEDLING BLIGHT
Seedling blight (caused by Pythium spp., Rhizoctonia solani, and Fusarium spp.) can kill seedlings before or after they emerge. Rot symptoms, either wet or dry, are observed in the presence of this disease. The lesions resulting from seedling blight caused by Rhizoctonia solani are reddishbrown to orange and appear sunken. Pythium spp. cause shoots or roots to appear gray and water-soaked. The incidence of seedling blight is higher when watermelons are planted in cool soils because seedlings that emerge slowly are more susceptible to pathogenic fungi (Roberts and Kucharek 2005).
ANTHRACNOSE
In the past, anthracnose (caused by Colletotrichum orbiculare) was a serious disease in Florida watermelon production, but the use of resistant varieties has limited its impact. Three races of anthracnose are known (races 1, 2, and 3), and some varieties show resistance to some races but not others. Anthracnose race 2 has caused serious damage to watermelons in the southeastern United States. When it does occur, anthracnose can destroy the entire field if not controlled, particularly after several days of warm, rainy weather (Bertelsen et al. 1994; Roberts and Kucharek 2005). All aboveground plant parts may be affected by this fungal disease, and infected plants may die under severe conditions. Early symptoms of the disease include angular, brown to black leaf spots on older leaves, similar in appearance to those of gummy stem blight or downy mildew. Tan, ovalshaped lesions may appear on the stems. Spores from leaf and stem lesions later infect the fruit, producing sunken, watersoaked spots. Disease development is greatest during humid, rainy weather. Spores are spread by wind, splashing rain, people, and machinery. The fungus, which can be seedborne, survives between crops on infected plant debris and volunteer plants (Bertelsen et al. 1994; Roberts and Kucharek 2005; Maynard and Hopkins 1999).
Chemical Control
In 2014, Florida growers applied fungicides totaling 294,800 pounds of active ingredient to 97% of the state’s watermelon acreage. Specific fungicides usage data is available through the National Agriculture Statistical Service. Fungicides labeled for use on Florida’s watermelon include 1,3-dichloropropene, chloropicrin, acibenzolar-s-methyl, ametoctradin, dimethomorph, azoxystrobin, benzovindiflupyr, difenoconazole, chlorothalonil, boscalid, basic copper sulfate, copper oxychloride, copper diammonia diacetate complex, copper hydroxide, copper octanoate, copper sulfate pentahydrate, cuprous oxide, cyazofamid, cyflufenamid, cymoxanil, cyprodinil, difenoconazole, dimethomorph, famoxadone, fenamidone, fluazinam, fludioxonil, fluopicolide, fluopyram, fluoxastrobin, flutriafol, fluxapyroxad, fosetyl-Al, hydrogen peroxide, peroxyacetic acid, kresoxim-methyl, laminarin, mancozeb, mandipropamid, mefenoxam, metalaxyl, metrafenone, myclobutanil, oxathiapiprolin, penthiopyrad, phosphorous acid, mono- and dibasic sodium, mono- and dipotassium salts, potassium, ammonium salts, polyoxin D zinc salt, potassium bicarbonate, potassium phosphate, potassium phosphite, potassium silicate, propamocarb hydrochloride, prothioconazole, pyraclostrobin, quinoxyfen, sulfur, tebuconazole, thiabendazole, thiophanate-methyl, thiram, trifloxystrobin, triflumizole, zoxamide, corn oil, cottonseed oil, garlic oil, neem oil, extract of Reynoutria sachalinensis, Bacillus amyloliquefaciens, Bacillus mycoides, Bacillus pumilus, Bacillus subtilis, Coniothyrium minitans strain CON/M/91-08, Gliocladium virens Gl-21, Streptomyces lydicus, Trichoderma asperellum (ICC 012), Trichoderma gamsii (ICC 080), Trichoderma harzianum, and Trichoderma virens (USDA/NASS 2021; CDMS 2017). For disease management recommendations refer to the “Watermelon Spray guide 2017” (Dufault and Paret 2017).
MANCOZEB
Mancozeb is an ethylene (bis) dithiocarbamate (EBDC; FRAC group M3) fungicide used prophylactically primarily for managing downy mildew. It may also be effective in managing gummy stem blight, anthracnose, alternaria leaf spot, and cercospora leaf spot (Vallad et al. 2010). Mancozeb may be applied up to 5 days before harvest (preharvest interval = 5 days), and the REI under the WPS is 24 hours. A maximum of 19.2 pounds of active ingredient (or EBDC fungicide in general) may be applied per acre for each crop (CDMS 2017)
CHLOROTHALONIL
Chlorothalonil is a broadspectrum chloronitrile fungicide (FRAC group M5) used as a protectant on Florida watermelons. Growers primarily apply it to manage gummy stem blight, downy mildew, and leaf spot, although it may also be effective in managing anthracnose and alternaria leaf spot (Vallad et al. 2010). Chlorothalonil may be applied up to harvest, and the REI under the WPS is 12 hours. It should not be applied to watermelons under conditions conducive to sunburn (e.g., high levels of heat and sunlight, drought, or poor vine canopy).
AZOXYSTROBIN
Azoxystrobin is a member of the strobilurin class (FRAC group 11) of fungicides. It is used to manage anthracnose as well as gummy stem blight, downy mildew, and leaf spot (Vallad et al. 2010). Azoxystrobin may be applied up to one day before harvest, and the REI under the WPS is four hours.
TEBUCONAZOLE
Tebuconazole is a member of the triazole class (FRAC group 3) of fungicides. It is used as a generalist fungicide for managing fungal diseases such as gummy stem blight and powdery mildew. It can be applied up to seven days before harvest and REI is 12 hours.
COPPER HYDROXIDE
Watermelon growers in Florida use copper hydroxide (FRAC Group M1) to manage downy mildew and bacterial fruit blotch (Vallad et al. 2010). Copper hydroxide may be applied up to the day of harvest, but the REI under the WPS is 24 hours.
Cultural Control
Incidence of aphid transmitted viral diseases can be reduced on fallplanted watermelons by planting as far as possible from fields planted to cucurbits the preceding spring (Hochmuth and Elmstrom 1992). In addition, removing volunteer watermelon and other cucurbit plants from around the field can further reduce the spread of viruses (Roberts and Kucharek 2005). Weeds should be removed before watermelons are planted because weed senescence during the growing season may cause insect pests, such as aphids, thrips, and whiteflies, to move into the crop. This practice is useful principally in South Florida. Researchers have been investigating the use of reflective mulches to repel aphids from landing on watermelons. Additionally, some growers are using purified mineral oil sprays (stylet oil) to prevent virus transmission.
In addition to reducing potential sources of viruses, weed management can improve the efficiency of fungicide sprays because weed presence can interfere with adequate spray coverage. Weeds near watermelon plants tend to increase crop moisture, which is a critical component for many fungal and bacterial disease infections and development. Therefore, properly managing weeds in and around the field is an essential component of overall disease management in watermelons (Roberts and Kucharek 2005).
Using resistant varieties and rotating land that has been out of watermelon production for at least eight years have been the primary means of managing Fusarium wilt of watermelon in Florida. Rotation also helps to manage gummy stem blight, seedling blights, and other fungal pathogens of watermelon. The best rotation crops for watermelons are grasses, such as corn or pasture grasses. Growers had been using land that was previously used for pasture for watermelon production, but the declining availability of land, particularly in South Florida, has made this practice more difficult especially for 8 to 10-year rotations (Bertelsen et al. 1994; Hochmuth et al. 1997; Spreen et al. 1995; Mitchell, Martin, and Charudattan 1994).
The minimum recommended rotation time for gummy stem blight is at least two and preferably four years. In addition to crop rotation, turning under old crop debris helps to manage gummy stem blight. Spores serving as the primary inoculum of the disease survive on watermelon, muskmelon, cucumber, squash, and pumpkin debris, and deep plowing prevents those spores from being spread by the wind into active watermelon fields. Deep plowing also helps to manage southern blight and leaf spots because it buries the pathogens that cause these diseases, inhibiting their movement. Furthermore, using drip irrigation and planting on a well-drained field with good air circulation can minimize periods of leaf wetness that intensify disease development (Roberts and Kucharek 2005).
Phytophthora blight is most effectively managed by controlling excess water (planting in well-drained fields and employing appropriate irrigation practices). Because the fungus can spread in contaminated soil, workers should wash hands and shoes before moving from one field to another (Roberts and McGovern 1998).
Bacterial fruit blotch can be seedborne, so it is important to use only seed and transplants free of bacterial fruit blotch inoculum. Researchers evaluating several seed treatments to eliminate the fruit blotch bacterium determined that fermentation of seeds in watermelon juice and debris followed by washing and drying reduced seed transmission to less than 1%. The most effective treatment was found to be the fermentation of seeds for 24–48 hours, followed by soaking of washed seeds in 1% hydrogen chloride or calcium hypochlorite for 15 minutes before washing and drying (Hopkins, Cucuzza, and Watterson 1996). Most companies selling watermelon seed now test their seed lots to declare them free of the fruit blotch pathogen but do not guarantee the complete absence of the pathogen. Changes in the ways seeds are handled and tested have led to great increases in the price of watermelon seeds for the grower (Latin and Hopkins 1995).
Several cultural practices other than crop rotation can also help to manage damping-off (seedling blight), including turning under or composting crop residues, avoiding planting in cool soils, and using healthy transplants or seed treatments. Furthermore, transplants should not be set too deeply, and they should not be exposed to production fields during transport, which can be avoided by using covers on transport vehicles (Roberts and Kucharek 2005).
Biological Control
There are many biological control agents available for watermelon disease management. Many of the products registered have indicated some disease control for the pathogens listed on the labels, however, this control often varies between regions and years in the state. Biological controls can be a useful component of an integrated disease management strategy and should be considered primarily as a protectant application. Consult your local extension office for more information on biological control options that are available (Paret et al. 2021).
A unique biological control situation was noted in Florida watermelon production for the management of Fusarium wilt over 20 years ago. Research founds that suppressive soils could develop in monocultures of the watermelon cultivar ‘Crimson Sweet’ (Mitchell, Martin, and Charudattan 1994). It is believed that the development of nonpathogenic populations of indigenous F. oxysporum and overall populations of nonpathogenic bacteria lead to the suppressive nature of the soils (Larkin, Hopkins, and Martin 1993). It also observed that the suppressive soils were sensitive to fumigation with methyl bromide and 30-minute treatment with moist heat (158°F or 70°C) (Hopkins, Larkin, and Elmstrom 1987). Some biological agents were identified as possible antagonists from these suppressive soils (Larkin, Hopkins, and Martin 1996), however, no effective biological product was developed from these antagonists. Further work is needed to understand how these suppressive soils manage the disease and how the cultivar ‘Crimson Sweet’ changes the phytobiome.
Postharvest Decays and Their Management
When postharvest diseases of watermelons occur, the most common are black rot and stem end rot. Bruising during shipment can reduce quality and contribute to postharvest decay. Decay organisms require entry points, such as mechanical damage or weakened tissue. They can also enter the fruit through contaminated water or other infected plant parts. Postharvest decay is minimized by following appropriate cultural practices, minimizing handling during harvest and shipping, and managing temperature during shipping and storage (Rushing 2016; Watson et al. 2015).
Black rot is a significant postharvest disease of watermelons. By the time infested fruits arrive at the market, they have developed green to black spots on the rind, which enlarge as the decay enters the watermelon interior. Black rot can leave the fruit susceptible to secondary bacterial and fungal infections (Rushing 2016). After infection occurs in the field, the disease can develop during shipment, and it is more severe under warm, humid conditions (Maynard and Hopkins 1999).
Stem end rot begins as the stem shrivels and turns brown, and the infection enters the fruit at the point of attachment with the stem. The watermelon tissue becomes watersoaked and softened, with accompanying browning and shriveling. When the disease is severe, black pycnidia (spores) and the gray mycelium (body of the fungus) can be seen (Rushing 2016).
These diseases can occur, but generally, postharvest disease problems on watermelons in Florida are minimal unless mechanical damage occurs. The move to ship watermelons in palletsized bins, rather than loading in bulk, should reduce postharvest disease incidence because bulk loading entails a greater potential for bruising and cracking (Rushing 2016).
Nematode Management
Nematode Pests
Nematodes are microscopic roundworms living in the soil that feed on the plants’ roots and damage the tissue. Watermelons are highly susceptible to nematode injury, as are all cucurbits. Damage can be particularly severe on sandy soil, the predominant soil type in Florida watermelon production. Typical aboveground symptoms of nematode feeding on watermelon roots include stunting, premature wilting, leaf yellowing, and related symptoms characteristic of nutrient deficiencies. Stunting and poor stand developments tend to occur in patches throughout the field as a result of the irregular distribution of nematodes in the soil. Damage and yield loss are directly related to the level of infestation by nematodes. The principal nematode pests on watermelons in Florida are rootknot (Meloidogyne spp.) and sting (Belonolaimus longicaudatus). Reniform nematodes (Rotylenchulus spp.) can be a problem in South Florida (Noling 2012a; Noling 2012b).
ROOTKNOT NEMATODE
All of the common species of rootknot nematodes (Meloidogyne spp.), including M. incognita, M. javanica, and M. arenaria, can damage watermelons in Florida. However, rootknot damage has historically been low in North and Central Florida because of growing watermelons in old pasture land or after long grass rotations to reduce damage from fusarium wilt (Noling 2012a; Noling 2012b). In southwest Florida, the lack of reported damage from rootknot nematodes is attributed to the use of methyl bromide.
Rootknot nematodes enter the host plant root as second-stage juveniles and settle within the root to establish a feeding site. At the feeding site, secretions from the nematode cause the surrounding plant cells to enlarge and multiply, producing the characteristic galls associated with rootknot attack. On watermelons, galls measuring from 1/8 to 1/4 inch (0.3–0.6 cm) in diameter begin to form as soon as the roots are infested. As more nematodes enter the root and feeding continues, the galls fuse to form large tumors on the roots. The developing female molts several times in the root before developing into a swollen, pearshaped adult. The adult may live in the host plant for several months, laying hundreds to several thousand eggs that are released into the soil. Low temperatures or very dry soil conditions can cause eggs to hatch more slowly (Noling 2012b; Stokes 1972; Crow and Dunn 2012).
Root deformation and injury caused by rootknot nematodes reduce root area and interfere with water and nutrient uptake. Resulting symptoms include stunting, wilting, chlorosis, and yield loss. In addition to expending the plant’s resources, the gall tissue is more susceptible to secondary infections such as root rots (Stokes 1972).
STING NEMATODE
Sting nematodes (Belonolaimus spp.) are ectoparasites, remaining outside the plant root and feeding superficially at or near the root tip by penetrating the root deeply with their long needlelike mouthparts (stylets). Affected root tips turn yellow and later necrotic, with cavities forming and the root tip swelling slightly. Damage from sting nematode feeding inhibits root elongation and causes roots to form tight mats and appear swollen, resulting in a "stubby root" or "coarse root" appearance. Under severe infestations, new root growth is killed in a way that resembles fertilizer salt burn (Noling 2012b; Crown and Dunn 2012; Esser 1976).
Sting nematodes are especially damaging to seedlings and transplants. In North Florida, they are most abundant in April and May. Sting nematodes prefer sandy soils (with 84%–94% sand) and are most abundant in the upper 12 inches (30 cm). The optimum soil temperature for this nematode is 77°F–90°F (25°C–32°C), and optimum soil moisture is about 7% (Noling 2012b; Crow and Dunn 2012; Esser 1976).
RENIFORM NEMATODE
Reniform nematodes (Rotylenchulus spp.) feed in the plant root, entering as secondstage juveniles and settling at a feeding site. By releasing growth regulators into the surrounding tissue, the nematodes cause the plant to redirect nutrients to the cells around the feeding site, using energy and disrupting the vascular system. However, they do not produce galls on the root tissue (Crow and Dunn 2012; Esser 1976).
Chemical Control
Oxamyl, 1,3-dichloropropene, and chloropicrin are the principal pesticides used to manage nematodes on Florida watermelons. Additional fumigant nematicides registered for use on watermelons include metam sodium and metam potassium. Fluensulfone and floupyram are non-fumigant nematicides that can be applied pre-plant incorporated or be drip applied pre-plant. Fluensulfone is not labeled for post plant application but floupyram does have a post plant label. Other nematicides registered for use on Florida’s watermelon include allyl isothiocyanate, azadirachtin, Burkholderia spp., Myrothecium verrucaria strain AARC-0255, Paecilomyces lilacinus strain 251, saponins of Quillaja saponaria, neem oil, sesame oil, and thyme Oil (USDA/NASS 2021; CDMS 2017).
Watermelon is usually grown as a double crop behind tomatoes or peppers in south Florida, and fumigants (1,3dichloropropene and chloropicrin) are applied to the first crop and not to the watermelons. However, growers might apply reduced rates of those fumigants to the watermelon when nematodes are a problem under new plastic mulch (Noling 2013).
Cultural Control
Crop rotation is an important cultural management tool and is frequently used by North Florida watermelon growers. These growers often plant watermelons on land after a long rotation of poor or nonhost pasture grass. However, as new land or pasture land is increasingly unavailable, the rotation time is correspondingly shortened or eliminated, and increased nematode problems have been observed. When the use of cover crops or rotational crops is considered, the choice of the crop depends on which nematode populations are present. Several types of grass, including corn, sorghum, bahiagrass, bermudagrass, and pangola digitgrass, have successfully reduced populations of rootknot nematodes, but other nematode species may increase on those crops. A nematode assay should be performed to determine the species of nematodes in the field (Spreen et al. 1995).
Several other cultural measures also help to manage nematodes in watermelon production. Rapidly destroying or removing crop roots after harvest reduces the material for nematodes to feed and reproduce on. In addition, maintaining weed-free fields between crops and practicing frequent cultivations during the season to reduce nematode harboring weeds further eliminate the source of continued infestation. In most instances, it is not possible to manage nematodes without simultaneously implementing an effective weed management program. Also, using strong, nematode free transplants gives watermelon plants a healthy start and may enhance their ability to tolerate nematode attacks (Spreen et al. 1995).
Biological Control
Numerous predators and parasites of nematodes are known, including fungi, bacteria, other nematodes, and mites. Many of these have been found in most of the surveyed soils. Attempts have been made to use organic amendments to create soil conditions more conducive to developing these nematode antagonists. Results of organic amendment studies have been controversial, in part because the amendments can affect plant growth directly, exclusive of any effect on the nematode population. The effectiveness of organic amendments depends on numerous factors, and these combined effects need further study (McSorley 1999; Dickson et al. 1994).
Mass production and field release of nematode antagonists have also been investigated. The bacterium Pasteuria penetrans is the most promising biological control agent, particularly for rootknot nematodes. Studies of the Pasteuria species biology have shown its potential as an effective management tool for nematodes, but two major problems make it impractical for commercial use. Most Pasteuria spp. isolates are very host-specific, so numerous products would have to be developed for use against the range of nematode pests affecting agricultural crops. In addition, methods for lowcost mass rearing of the bacteria, which require a full understanding of its nutrient requirements, have not yet been developed. P. penetrans is commonly found in soils throughout Florida, and researchers are also investigating its soil ecology to find ways to increase its presence in soils. While Pasteuria has shown promise in reducing nematode populations, its use as a biological control agent for nematode management is still in the research stage (McSorley 1999; Dickson et al. 1994; Hewlett et al. 1994; Chen and Dickson 1998).
Weed Management
Weeds
Competition from weeds can be severe in watermelon production because of the slow growth rate of the crop early in the season, its low planting density, and low vining habit. Early-season weed management is essential. Weeds late in the season can reduce the efficiency of harvest and incur losses, but yield loss from competition does not occur when weeds emerge later in the growth of the watermelon crop. When weed populations are high, watermelon yield can be reduced by 50%–100% without weed management. When weeds are managed, the potential yield loss from weed competition still reaches an estimated 15% (Hochmuth et al. 1997; Stall 1992; Dittmar and Boyd 2019; Locascio et al. 1989).
A variety of weeds are problematic for Florida watermelon producers, including nutsedges (yellow and purple), grasses (for example, crabgrass, goosegrass, and Texas panicum), and broadleaf weeds, such as bristly starbur, Florida pusley, and purslane. Amaranths are particularly troublesome, and nutsedge is becoming a major problem. The specific weeds present will vary by region in the state and by previous land use. For example, on recently cleared land or land in grass production for a long period, weed pressure is usually low. On the other hand, pressure from broadleaf and grass weeds can be high on recently cultivated land (Stall 1992).
NUTSEDGE
Two nutsedges (Cyperus spp.), yellow nutsedge (C. esculentus), and purple nutsedge (C. rotundus) are among the greatest weed problems in Florida watermelon production and will pose a greater dilemma without the use of methyl bromide. Both of these perennial sedges are found in disturbed habitats throughout Florida and the southeast United States. Yellow nutsedge may produce some seed but reproduces primarily by rhizomes and tubers. The parental plant develops rhizomes, which end in bulbs or tubers that produce new plants. Tuber production is favored by low nitrogen levels and high temperatures (80°F–91°F or 27°C–33°C). The plant is tolerant of high soil moisture but does not tolerate shade. Purple nutsedge can reproduce from tubers when conditions are harsh, making it difficult to control. Unlike yellow nutsedge rhizomes, purple nutsedge rhizomes growing off the parent plant produce new plants in a series (called "tuber chains"). The plant also reproduces by seed to a limited degree. Although purple nutsedge is also intolerant of shade, it can survive a wide range of environmental conditions. It can grow well in nearly all soil types and in a range of soil moisture, soil pH, and elevation. Purple nutsedge is also able to survive extremely high temperatures (Miller et al. 1975).
Recent research in Florida has shown that the presence of 25 yellow nutsedge plants per square meter (2.32 per square foot) in the watermelon bed throughout the season reduces yield by 98%, and six yellow nutsedge plants per square meter (0.56 per square foot) reduces yield by 20%. Nutsedge plants emerging at least five weeks after watermelons have no effect on watermelon yield (Stall 1999).
AMARANTH
Amaranths (Amaranthus spp.), called pigweeds, are summer annual broadleaf herbs with erect stems that can grow to 6.5 feet (2 m) tall. Several species of amaranth are present in Florida, but the main amaranth weeds in watermelons are smooth pigweed (Amaranthus hybridus) and spiny amaranth (Amaranthus spinosus). Amaranths or pigweeds reproduce solely by seed, producing very small, dark seeds. Smooth pigweed flowers from July to November, and spiny amaranth flowers from June to October. They prefer open areas with bright sunlight (Miller et al. 1975; Lorenzi and Jeffery 1987).
Research in Florida has shown that the presence of six smooth pigweed plants between watermelon plants throughout the whole season will reduce watermelon yield by 100%. However, watermelon plants maintained free of emerging smooth pigweed for four weeks suffer no yield loss as a result of competition from smooth pigweed plants emerging after that time. Also, a weed-free period of approximately three weeks after the crop's emergence results in a 10%–20% yield loss. In addition, 10% yield loss results if smooth pigweed that emerges at the same time as the watermelon is removed after five days, and watermelon suffers 20% yield loss after two weeks (Stall 1999; Terry et al. 1997).
CRABGRASS
Crabgrasses (Digitaria spp.) are annual grass plants that reproduce mainly by seed, but also by spreading and rooting of stems at the base. They germinate during the summer, flowering from June or July to October, and quickly establish clumps. Crabgrass thrives in moist soil (Miller et al. 1975; Lorenzi and Jeffery 1987). A study of competition from large crabgrass in watermelon production demonstrated that when the weed emerged at least six weeks after watermelon emergence, no reduction of yield or fruit quality occurred. However, watermelon yield was reduced by 7% for every week once large crabgrass was present, and yield loss from competition throughout the whole growing season reached more than 70% (Stall 1999).
GOOSEGRASS
Goosegrass (Eleusine indica) is similar in appearance to crabgrass but grows more densely. It is also a summer annual and prefers sunny, moist conditions. Reproducing by seed, it flowers from July to October (Miller et al. 1975; Lorenzi and Jeffery 1987). As few as 24 goosegrass plants per 30 feet (9.1 m) of row can reduce watermelon yield (Stall 1999).
TEXAS PANICUM
Texas panicum (Panicum texanum) is primarily a problem in South Florida. A summer annual with erect stems, the plant produces large seeds and roots at the nodes (Miller et al. 1975).
BRISTLY STARBUR
Bristly starbur (Acanthospermum hispidum) is present only in the northern part of the state, and it is becoming a major problem for watermelon producers in that region. This highly competitive weed also appears to be spreading southward. Bristly starbur received its common name because of the bristly appearance of the flat, triangular fruits, several of which are clumped at each head. Fruits, stems, and leaves are all densely covered with hairs. Bristly starbur produces abundant seeds until the plant freezes in the fall. Deep plowing is thought to aid in reducing its population because seeds buried below 3 inches (7.5 cm) in the soil have been found to lose viability after three years (Dittmar and Boyd 2019).
FLORIDA PUSLEY
Florida pusley (Richardia scabra) is a loosely branched annual that stands erect or lies flat on the ground. Its hairy stems and oppositely arranged leaves are often rough in texture, particularly along the main veins. The plant is only found in Central and North Florida and is often mixed with Brazilian pusley (R. brasiliensis). Florida pusley reproduces by seed and blooms in any month in the absence of frost (Dittmar and Boyd 2019).
PURSLANE
Purslane (Portulaca oleracea) is a broadleaf summer annual with a single taproot. Multiple branched, purplishred stems arise from the taproot and often form large mats. Clusters of small leaves are found at the end of its branches. The plant reproduces by seed, flowering from August to October. Being resistant to drought, it is difficult to kill. However, it is susceptible to frost injury (Miller et al. 1975; Lorenzi and Jeffery 1987).
Chemical Control
In 2014, Florida growers applied herbicides totaling 8,900 pounds of active ingredient to 48% of the state’s watermelon acreage. Herbicides registered for use on Florida’s watermelon include ammoniated soap of fatty acids, ammonium nonanoate, bensulide, benzovindiflupyr, difenoconazole, capric acid, caprylic acid, carfentrazone-ethyl, clethodim, clomazone, ethalfluralin, glyphosate, diammonium salt, dimethylamine salt, isopropylamine salt, monoammonium salt, potassium salt, indole-3-butyric acid, kinetin, halosulfuron-methyl, imazosulfuron, metam-potassium, metam-sodium, oxyfluorfen, paraquat dichloride, pelargonic acid, pendimethalin, pyraflufen-ethyl, sethoxydim, sulfentrazone, terbacil, trifluralin, cinnamon oil, and clove oil (USDA/NASS 2021; CDMS 2017).
When applying herbicides, it is essential to follow label directions. Watermelons have a narrow range of tolerance for most herbicides, and incorrect application can easily damage the crop (Dittmar and Boyd 2019).
Cultural Control
Adequate management of weeds in Florida watermelon production cannot be obtained with herbicides alone. Weed management in watermelon requires a combination of tactics, including good field preparation and cultivation (Hochmuth et al. 1997; Locascio et al. 1989). Because of the wider row spacing in watermelon production, between-row cultivation is more common for watermelons than for other cucurbits such as cucumbers and cantaloupe. Cultivation must be shallow to avoid injuring crop roots, and it is limited to the first 4–5 weeks after emergence. Mechanical cultivation is impractical after that time due to the running of vines, and it is no longer cost-effective because yields are reduced by weed competition primarily during the early weeks (Stall 1999; Terry et al. 1997). Used early in the season, mechanical control (disking, hoeing, mowing, or cultivation) is an important part of the overall weed management program for watermelons.
Using appropriate water and nutrient management practices to allow a healthy crop to better compete with weed species is also important (Spreen et al. 1995; Locascio et al. 1989). Fallow treatments before planting are also effective in reducing weed populations. Weeds are disked under several times after emergence but before propagation, and the mechanical fallowing is often combined with herbicide treatments applied two weeks before disking (Stall 1999). Using plastic mulch is an important management tool for all weeds except nutsedges, which can grow through the mulch (Spreen et al. 1995).
References
Adlerz, W. C. 1987. “Cucurbit Potyvirus Transmission by Alate Aphids (Homoptera: Aphididae) Trapped Alive.” Journal of Economic Entomology 80: 87–92.
Adlerz, W. C. 1978. “Secondary Spread of Watermelon Mosaic Virus 2 by Anuraphis middletonii.” Journal of Economic Entomology 71: 531–533.
Athearn, K., M. Burani-Arouca, B. Hochmouth, J. Freeman, N. Dufault, B. Broughton, T. Sanchez, L. Harlow, T. Pittman, and M. Warren. 2020. Watermelon Production Budget for North Florida. https://svaec.ifas.ufl.edu/agribusiness/farm-enterprise-budgets/
Bertelsen, D., J. Harwood, F. Hoff, H. Lee, A. Perez, S. Pollack, A. Somwaru, and G. Zepp. 1994. Watermelons: An Economic Assessment of the Feasibility of Providing MultiplePeril Crop Insurance. USDA Economic Research Service, in cooperation with the University of California for the Federal Crop Insurance Corporation.
Capinera, J. L. 2020. Banded Cucumber Beetle, Diabrotica balteata LeConte (Insecta: Coleoptera: Chrysomelidae). EENY093. Gainesville: University of Florida Institute of Food and Agricultural Sciences. https://edis.ifas.ufl.edu/in250
Castineiras, A., R. M. Baranowski, and H. Glenn. 1997. Distribution of Neoseiulus cucumeris (Acarina: Phytoseiidae) and Its Prey, Thrips palmi (Thysanoptera: Thripidae) within Eggplants in South Florida. Florida Entomologist 80 (2): 211–217.
Castineiras, A., R. M. Baranowski, and H. Glenn. 1996. Temperature Response of Two Strains of Ceranisus menes (Hymenoptera: Eulophidae) Reared on Thrips palmi (Thysanoptera: Thripidae). Florida Entomologist 79 (1): 13–19.
Castineiras, A., J. E. Peña, R. Duncan, and L. Osborne. 1996. Potential of Beauveria bassiana and Paecilomyces fumosoroseus (Deuteromycotina: Hyphomycetes) as Biological Control Agents of Thrips palmi (Thysanoptera: Thripidae). Florida Entomologist 79 (3): 458–461.
Chen, Z. X., and D. W. Dickson. 1998. “Review of Pasteuria penetrans: Biology, Ecology, and Biological Control Potential.” Journal of Nematology 30 (3): 313–340.
Crop Data Management Systems, Inc. (CDMS). CDMS Label Search. https://www.cdms.net/
Crow, W. T., and R. A. Dunn. 2012. Introduction to Plant Nematology. ENY016. Gainesville: University of Florida Institute of Food and Agricultural Sciences.
Dufault, N. S. and M. L. Paret. 2017. Watermelon Spray Guide 2017. Online publication accessed on June 2017. https://nwdistrict.ifas.ufl.edu/phag/files/2017/01/UF_Watermelon_Spray_Guide_2017_Final.pdf
Dickson, D. W., M. Oostendorp, R. M. GiblinDavis, and D. J. Mitchell. 1994. “Control of Plant Parasitic Nematodes by Biological Antagonists.” In Pest Management in the Subtropics, Biological Control: A Florida Perspective, edited by D. Rosen, F. D. Bennett, and J. L. Capinera, 575–601. Andover, UK: Intercept.
Dittmar, P. J., and N. S. Boyd. 2019. Weed Control in Cucurbit Crops (Muskmelon, Cucumber, Squash, and Watermelon). HS190. Gainesville: University of Florida Institute of Food and Agricultural Sciences. http://edis.ifas.ufl.edu/wg029
Dixon, W. N. 1988. White-Fringed Beetles, Graphognathus spp. Entomology Circular 309. Gainesville, FL: FDACS, Division of Plant Industry.
Esser, R. P. 1976. Sting Nematodes, Devastating Parasites of Florida Crops. Nematology Circular No. 18. Gainesville: FDACS, Division of Plant Industry.
Florida Department of Agriculture and Consumer Services (FDACS). 2012. Florida Agriculture by the Numbers. Tallahassee, FL: FDACS. https://florida-agriculture.com/brochures/P-01304.pdf
Frank, J. H. 1994. “Biological Control of Pest Mole Crickets.” In Pest Management in the Subtropics Biological Control: A Florida Perspective, edited by D. Rosen, F. D. Bennett, and J. L. Capinera, 343–352. Andover, UK: Intercept.
Frank, J. H., T. R. Fasulo, and D. E. Short. 1998. “Alternative Methods of Mole Cricket Control.” In Mole Cricket Knowledgebase. Gainesville: University of Florida Institute of Food and Agricultural Sciences.
Frankle, W. G., D. L. Hopkins, and R. E. Stall. 1993. “Ingress of the Watermelon Fruit Blotch Bacterium into Fruit.” Plant Disease 77: 1090– 1092.
Gray, D. J. n.d. Transgenic Seedless Watermelon Resistant to Viruses. TStar Caribbean Program in Tropical/Subtropical Agricultural Research.
Hewitt, T. D. 1996. “Economic Considerations for Direct Seeding vs. Transplanting of Watermelons.” Citrus & Vegetable Magazine 60 (7): 38–40.
Hewlett, T. E., R. Cox, D. W. Dickson, and R. A. Dunn. 1994. “Occurrence of Pasteuria spp. in Florida.” Supplement to the Journal of Nematology 26 (4S): 616–619.
Hochmuth, G. 1992. “Fertilizer Management for Watermelons.” In Watermelon Production Guide for Florida, edited by D. N. Maynard. SP113. Gainesville: University of Florida Institute of Food and Agricultural Sciences.
Hochmuth, G., and G. Elmstrom. 1992. “Cultural Practices.” In Watermelon Production Guide for Florida, edited by D. N. Maynard. SP113. Gainesville: University of Florida Institute of Food and Agricultural Sciences.
Hochmuth, G. J., W. M. Stall, T. D. Hewitt, and K. C. Ruppert. 1997. Alternative Opportunities for Small Farms: Watermelon Production Review. RFAC029. Gainesville: University of Florida Institute of Food and Agricultural Sciences.
Hochmuth, R. 2003. Vegetable Extension agent, UF/IFAS Suwannee Valley Research and Education Center, in communication with the author.
Hopkins, D. 1991. “Chemical Control of Bacterial Fruit Blotch of Watermelon.” Proceedings of the Florida State Horticultural Society 104: 270–272.
Hopkins, D. 2003. Plant pathologist, UF/IFAS Central Florida Research and Education Center, in communication with the author.
Hopkins, D., J. D. Cucuzza, and J. C. Watterson. 1996. “Wet Seed Treatment for the Control of Bacterial Fruit Blotch of Watermelon.” Plant Disease 80: 529–532.
Hopkins, D. L., R. P. Larkin, and G. W. Elmstrom. 1987. “Cultivar-Specific Induction of Soil Suppressiveness to Fusarium Wilt of Watermelon.” Phytopathology 77: 607–611.
Hopkins, D. L., R. J. Lobinske, and R. P. Larkin. 1992. “Selection for Fusarium oxysporum f.sp. niveum Race 2 in Monocultures of Watermelon Cultivars Resistant to Fusarium Wilt.” Phytopathology 82: 290–293.
Johnson, F. A. 1992. “Pollination.” In Watermelon Production Guide for Florida, edited by D. N. Maynard. SP113. Gainesville: University of Florida Institute of Food and Agricultural Sciences.
Johnson, F. A., D. E. Short, and J. L. Castner. 2005. Sweetpotato/Silverleaf Whitefly Life Stages and Damage. SP90. Gainesville: University of Florida Institute of Food and Agricultural Sciences.
Kucharek, T., J. P. Jones, D. Hopkins, and J. Strandberg. 1992. Some Diseases of Vegetable and Agronomic Crops Caused by Fusarium in Florida. Circular 1025. Gainesville: University of Florida Institute of Food and Agricultural Sciences.
Larkin, R. P., D. L. Hopkins, and F. N. Martin. 1993. “Effect of Successive Watermelon Plantings on Fusarium oxysporum and Other Microorganisms in Soils Suppressive and Conducive to Fusarium Wilt of Watermelon.” Phytopathology 83: 1097–1105.
Larkin, R. P., D. L. Hopkins, and F. N. Martin. 1996. “Suppression of Fusarium Wilt of Watermelon by Nonpathogenic Fusarium oxysporum and Other Microorganisms Recovered from a Disease-Suppressive Soil.” Phytopathology 86: 812–819.
Latin, R. X., and D. L. Hopkins. 1995. “Bacterial Fruit Blotch of Watermelon: The Hypothetical Exam Question Becomes Reality.” Plant Disease 79 (8): 761–765.
Locascio, S. J., W. M. Stall, S. M. Olson, and C. S. Vavrina. 1989. “Watermelon Production as Influenced by Herbicide Combination and Cultivation.” Proceedings of the Florida State Horticultural Society 102: 332–335.
Lorenzi, H. J., and L. S. Jeffery. 1987. Weeds of the United States and Their Control. New York: Van Nostrand Reinhold Company.
Maynard, D. 2003. Horticultural scientist, UF/IFAS Gulf Coast Research and Education Center, in communication with the author.
Maynard, D. N. 1996. Growing Seedless Watermelon. Gainesville: University of Florida Institute of Food and Agricultural Sciences.
Maynard, D. N. 1992. “Watermelon Varieties.” In Watermelon Production Guide for Florida, edited by D. N. Maynard, 3–4. Gainesville: University of Florida Institute of Food and Agricultural Sciences.
Maynard, D. N., and D. L. Hopkins. 1999. “Watermelon Fruit Disorders.” HortTechnology 9 (2): 155–161.
McAuslane, H. J., H. A. Smith. 2018. Sweetpotato Whitefly B biotype, Bemisia tabaci (Gennadius) (Insecta: Hemiptera: Aleroididae). EENY-129. University of Florida Institute of Food and Agricultural Sciences. https://edis.ifas.ufl.edu/in286
McSorley, R. 1999. “Nonchemical Management of PlantParasitic Nematodes.” The IPM Practitioner 21 (2): 1–7.
Miller, J. F., A. D. Worsham, L. L. McCormick, D. E. Davis, R. Cofer, and J. A. Smith. 1975. Weeds of the Southern United States. Gainesville: University of Florida Institute of Food and Agricultural Sciences.
Mitchell, D. J., F. N. Martin, and R. Charudattan. 1994. “Biological Control of Plant Pathogens and Weeds in Florida.” In Pest Management in the Subtropics, Biological Control: A Florida Perspective, edited by D. Rosen, F. D. Bennett, and J. L. Capinera, 549–574. Andover, UK; Intercept.
Newark, M., M. L. Paret, N. S. Dufault, and J. H. Freeman. 2014. Management of Gummy Stem Blight (Black Rot) on Cucurbits in Florida. PP280. Gainesville: University of Florida Institute of Food and Agricultural Sciences. https://edis.ifas.ufl.edu/pp280
Newark, M., M. L. Paret, N. S. Dufault, P. D. Roberts, S. Zhang, G. E. Vallad, J. H. Freeman, and G. McAvoy. 2016. Management of Cucurbit Downy Mildew in Florida. PP325. Gainesville: University of Florida Institute of Food and Agricultural Sciences. https://edis.ifas.ufl.edu/pp325
Nguyen, K. B., and G. C. Smart, Jr. 1990. “Steinernema scapterisci n.sp. (Rhabditida: Steinernematidae).” Journal of Nematology 22 (2): 187–199.
Noling, J. W. and J. P. Gilreath. 1999. “Alternatives to Methyl Bromide for Nematode Control: A South Florida Synopsis.” Proceedings of the 1998 Annual International Research Conference on Methyl Bromide Alternatives and Emissions Reductions. December 7–9, 1998, Orlando, Florida.
Noling, J.W. 2012a. Nematode Management in Cucurbits (Cucumber, Melons, Squash). ENY025. Gainesville: University of Florida Institute of Food and Agricultural Sciences. https://edis.ifas.ufl.edu/ng025
Noling, J.W. 2012b. Nematodes and Their Management. ENY625. Gainesville: University of Florida Institute of Food and Agricultural Sciences. https://doi.org/10.32473/edis-cv112-2006
Noling, J. W. 2013. Nematologist, UF/IFAS Citrus Research and Education Center, in communication with the author.
Norman, J. W., Jr., D. G. Riley, P. A. Stansly, P. C. Ellsworth, and N. C. Toscano. 1993. Management of Silverleaf Whitefly: A Comprehensive Manual on the Biology, Economic Impact and Control Tactics. Washington, DC: USDA/CSREES.
Nuessly, G. S., and M. Hentz. 1998. EREC Moth Pheromone Trap Data. Gainesville: University of Florida Institute of Food and Agricultural Sciences.
Olson, S. M., P. J. Dittmar, P. D. Roberts, S. E. Webb, and S. A. Smith. 2012. Cucurbit Production in Florida. HS725. Gainesville: University of Florida Institute of Food and Agricultural Sciences. https://edis.ifas.ufl.edu/cv123
Paret, M. L., P. J. Dittmar, S. Agehara, and H. A. Smith. 2021. Vegetable Production Handbook of Florida, 2021-2022. #VPH. University of Florida Institute of Food and Agricultural Sciences. https://edis.ifas.ufl.edu/cv292
Personal communication with Susan Webb, Entomologist, University of Florida, Entomology and Nematology Department, Gainesville. 2003.
Purcifull, D. E., W. C. Adlerz, G. W. Simone, E. Hiebert, and S. R. Christie. 1984. “Serological Relationships and Partial Characterization of Zucchini Yellow Mosaic Virus Isolated from Squash in Florida.” Plant Disease 68: 230–233.
Roberts P. D., and T. A. Kucharek. 2018. Vegetable Diseases Caused by Phytophthora capsici in Florida. SP159. Gainesville: University of Florida Institute of Food and Agricultural Sciences. https://edis.ifas.ufl.edu/vh045
Roberts, P., and T. Kucharek. 2005. 2006 Florida Plant Disease Management Guide: Watermelon. PDMGV355. Gainesville: University of Florida Institute of Food and Agricultural Sciences. https://ufdcimages.uflib.ufl.edu/UF/00/05/38/71/00027/PG06000.pdf
Roberts, P. D., and R. J. McGovern. 1998. “Phytophthora Blight Causes Significant Losses to Spring Vegetable Crops.” Citrus & Vegetable Magazine 62 (11): 8–11.
Rushing, J. W. 2016. “Watermelon.” In The Commercial Storage of Fruits, Vegetables, and Florist & Nursery Crops, 3rd edition, edited by K. Gross and M. Saltveit. USDA Handbook 66. Washington, DC: USDA/ARS. https://www.ars.usda.gov/ARSUserFiles/oc/np/CommercialStorage/CommercialStorage.pdf
Sakimura, K., L. M. Nakahara, and H. A. Denmark. 1986. A Thrips, Thrips palmi Karny (Thysanoptera: Thripidae). Entomology Circular No. 280. Gainesville, FL: FDACS Division of Plant Industry.
Sanford, M. T., and J. Ellis. 2011. Beekeeping: Watermelon Pollination. ENY154. Gainesville: University of Florida Institute of Food and Agricultural Sciences. https://edis.ifas.ufl.edu/aa091
Sargent, S. A. 1992. “Harvesting and Handling Watermelons.” In Watermelon Production Guide for Florida, edited by D. N. Maynard. SP113. Gainesville: University of Florida Institute of Food and Agricultural Sciences.
Schuster, D. J., and J. F. Price. 1992. “Seedling Feeding Damage and Preference of Scapteriscus spp. Mole Crickets (Orthoptera: Gryllotalpidae) Associated with Horticultural Crops in WestCentral Florida.” Florida Entomologist 75 (1): 115–119.
Somodi, G. C., J. B. Jones, D. L. Hopkins, R. E. Stall, T. A. Kucharek, N. C. Hodge, and J. C. Watterson. 1991. “Occurrence of a Bacterial Watermelon Fruit Blotch in Florida.” Plant Disease 75: 1053–1056.
South, L. 1991. “New Thrips Threatens South Florida.” American Vegetable Grower 39 (5): 30.
Spreen, T. H., J. J. VanSickle, A. E. Moseley, M. S. Deepak, and L. Mathers. 1995. Use of Methyl Bromide and the Economic Impact of Its Proposed Ban on the Florida Fruit and Vegetable Industry. Gainesville: University of Florida Institute of Food and Agricultural Sciences.
Stall, W. 1999. “Watermelon Weed Control.” Citrus & Vegetable Magazine 63 (7): 30–34.
Stall, W. M. 1992. “Weed Management.” In Watermelon Production Guide for Florida, edited by D. N. Maynard, 45–46. SP113. Gainesville: University of Florida Institute of Food and Agricultural Sciences.
Stansly, P. A. 2011. Insects That Affect Vegetable Crops. ENY450. Gainesville: University of Florida Institute of Food and Agricultural Sciences. https://ufdc.ufl.edu/IR00004249/00001/pdf
Stansly, P. A. 1995. “Seasonal Abundance of Silverleaf Whitefly in Southwest Florida Vegetable Fields.” Proceedings of the Florida State Horticultural Society 108: 234–242.
Stephens, J. M. 2012. Watermelon, Seedless Citrullus lanatus (Thunb.) Mansf. HS685. Gainesville: University of Florida Institute of Food and Agricultural Sciences. https://edis.ifas.ufl.edu/mv152
Stokes, D. E. 1972. Root Knot Nematodes (Meloidogyne spp.). Nematology Circular No. 11. Gainesville, FL: FDACS, Division of Plant Industry.
Terry, E.R., Jr., W. M. Stall, D. G. Shilling, T. A. Bewick, and S. R. Kostewicz. 1997. “Smooth Amaranth Inference with Watermelon and Muskmelon Production.” HortScience 32 (4): 630–632.
Tsai, J. H., B. Yue, S. E. Webb, J. E. Funderburk, and H. T. Hsu. 1995. “Effects of Host Plant and Temperature on Growth and Reproduction of Thrips palmi (Thysanoptera: Thripidae).” Environmental Entomology 24 (6): 1598–1603.
USDA/NASS. 2021. United States Department of Agriculture; National Agricultural Statistics Service. https://www.nass.usda.gov
Vallad, G., K. Pernezny, N. Peres, R. Raid, P. Roberts, and S. Zhang. 2010. 2010 Florida Plant Disease Management Guide: Chemical Control Guide for Diseases of Vegetables, Revision No. 21. PPP6. Gainesville: University of Florida Institute of Food and Agriculture Sciences.
Vavrina, C. S. 1992. “Watermelon Transplants.” In Watermelon Production Guide for Florida, edited by D. N. Maynard. SP113. Gainesville: University of Florida Institute of Food and Agricultural Sciences.
Watson, J. R., and A. N. Tissot. 1942. Insects and Other Pests of Florida Vegetables. Bulletin 370. Gainesville: University of Florida, Florida Agricultural Experiment Station.
Watson, J. A., D. Treadwell, S. A. Sargent, J. K. Brecht, and W. Pelletier. 2015. Postharvest Storage, Packing and Handling of Specialty Crops: A guide for Florida Small Farm Producers. HS1270. Gainesville: University of Florida Institute of Food and Agricultural Sciences. https://edis.ifas.ufl.edu/hs1270
Webb, S. E. 1995. Damage to watermelon seedlings caused by Frankliniella fusca (Thysanoptera: Thripidae). Florida Entomologist 78(1):178-179. https://journals.flvc.org/flaent/article/view/74676/72334
Webb, S. E. 1996. Management of melon aphid on muskmelon and watermelon with insecticides specific for Homoptera. Proc. Fla. State Hort. Soc. 109:202-205.
Webb, S. E. 2017. Insect Management for Cucurbits (Cucumber, Squash, Cantaloupe, and Watermelons). Entomology and Nematology Department ENY-460, Florida Cooperative Extension Service, Institute of Food and Agricultural Sciences, University of Florida. https://edis.ifas.ufl.edu/in168
Webb, S.E. and Kok-Yokomi, M.L. 1993. Transmission of cucurbit potyviruses by Uroleucon pseudambrosiae (Homoptera: Aphididae), an aphid trapped during epidemics of watermelon mosaic virus 2 in Florida. J. Econ. Entomol. 86(6):1786-1792.
Webb, S.E. and Linda, S.B. 1993. Effect of oil and insecticide on epidemics of potyviruses in watermelon in Florida. Plant Disease 77:869-874.
Webb, S. E., D. J. Schuster, P. A. Stansly, J. E. Polston, S. Adkins, C. A. Baker, P. Roberts, O. E. Liburd, T. Nyoike, E. McAvoy, and A. Whidden. 2015. Recommendations for Management of Whiteflies, Whitefly-Transmitted Viruses, and Insecticide Resistance for Production of Cucurbit crops of Florida. ENY-478. Gainesville: University of Florida Institute of Food and Agricultural Sciences. https://edis.ifas.ufl.edu/in871
Webb, S.E., Kok-Yokomi, M.L., and Voegtlin, D.J. (1994). Effect of trap color on species composition of alate aphids (Homoptera: Aphididae) caught over watermelon plants. Florida Entomologist 77(1):146-154. Available: https://journals.flvc.org/flaent/article/view/59026/56705