This publication is part of a series titled Onsite Sewage Treatment and Disposal Systems, commonly referred to as septic systems. This series is intended to give state and local government officials, soil scientists, consulting engineers, Extension agents, and citizens a basic understanding of onsite wastewater treatment and the behavior of different wastewater-borne contaminants coming from septic systems.
Introduction and Purpose
Characterizing the behavior and transport of nitrogen (N) in onsite sewage treatment and disposal systems (hereafter referred to as septic systems) is important because N present in wastewater can result in groundwater and surface water pollution. In the United States, about 4,800 water bodies are impaired due to excess N (U.S. EPA 2000). Septic systems are recognized as one source of N pollution (Hossain et al. 2010; Oakley et al. 2010).
In Florida, groundwater is vulnerable to N pollution in many areas because of limited confining layers, shallow water tables, and numerous sinkholes and caves that can rapidly transport N and other contaminants to groundwater (Arthur et al. 2007; Meeroff et al. 2008). Nearly 40% of Florida's septic systems are located in coastal areas, where the wet season water table is likely to be shallow and where porous sandy soils allow rapid transport of contaminants to groundwater. Statewide, septic systems discharge more than 426 million gallons of wastewater per day into the subsurface soil environment (Meeroff et al. 2008). Knowledge about the nature of contaminants like N in septic systems is a necessary first step to protect groundwater quality.
The purpose of this publication is to describe the behavior and transport of N from a conventional septic system as these are the most common onsite systems in the United States. Here we summarize the (1) sources of N in sewage, (2) forms and behavior of N in the septic tank, (3) forms and behavior of N in the drain field (also called the soil treatment unit), and (4) fate and transport of N in groundwater. For an overview of wastewater flow and quality, consult Toor et al. (2011), EDIS article "Onsite Sewage Treatment and Disposal Systems: An Overview" available at https://edis.ifas.ufl.edu/ss549.
Sources of Nitrogen in Sewage Wastes
The main sources of N in septic tank effluent are human body wastes and food materials from kitchen sinks and dishwashers. Estimates of mass loadings of nitrogen in septic systems can be calculated by combining flow and N concentration data. The US EPA (1992) estimated that one person typically discharges 11.2 grams of total N per day (Table 1). Of total N, approximately 8.7 grams (78%) comes from toilets, 1.9 grams (17%) from baths, sinks, and appliances, and 0.6 grams (5%) from kitchen sinks (US EPA 1992). This input of N waste products in household wastewater results in total N concentrations of 30–170 mg/L (average = 60 mg/L) in the septic tank effluent (Lowe et al. 2007). Lowe et al. (2009) found that septic tank effluent at four sites in North Florida had a median total N concentration of 65 mg/L (average = 61 mg/L).
Nitrogen Input with Septic Systems
To place N input from septic systems in context with other sources, such as atmospheric deposition and agricultural application of fertilizers, the following can be calculated:
An average subdivision of 200 homes (with four people per house) generates about 3,270 kg of total N per square kilometer (1 square kilometer has about 247 acres) each year [= 200 homes x 4 people x 11.2 grams total N per day x 365 days in a year].
In comparison, assuming 2.43–4.86 kg of total N atmospheric deposition per acre per year, total N loading from atmospheric deposition can add 600–1,200 kg each year in 1 square kilometer (247 acres).
Likewise, assuming a fertilizer application rate of 40–80 kg total N per acre per year for agricultural crops, fertilizer can add 10,000–20,000 kg of total N in 1 square kilometer (247 acres). It is important to note that most of the fertilizer N will be taken up by plants.
Nonetheless, conventional septic systems should be considered as potential contributors of N to groundwater, especially in Florida where only 30%–40% of effluent N is typically removed in the septic tank and drain field. This means 60%–70% of N can reach groundwater. The drinking-water standard for nitrate-N in groundwater is 10 mg/L, but concentrations above that have been observed under septic systems, particularly in areas with porous sandy soils (Wilhelm et al. 1994; Robertson and Cherry 1992).
Nitrogen Forms and Transformations in the Septic Systems
Nitrogen takes many forms, both organic and inorganic, and various natural processes transform N from one form to another in both the septic tank and the drain field of a septic system. The N cycle (Figure 1) demonstrates these natural processes that drive N transformations, and they are the same processes that take place in a septic system. How these processes occur in the septic tank effluent and the drain field are discussed below.
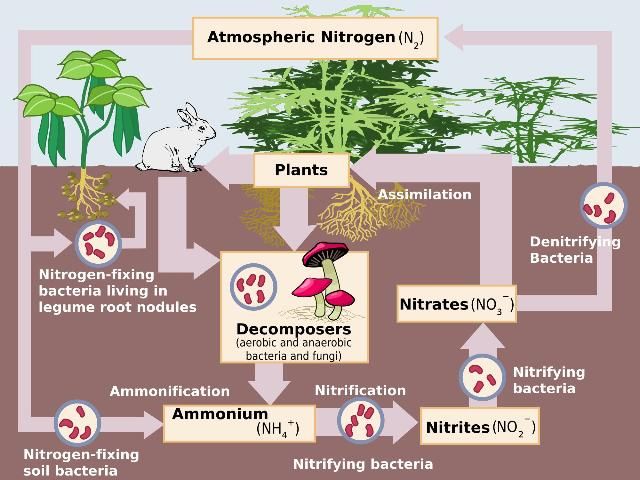
Credit: Wikimedia Commons, http://en.wikipedia.org/wiki/File:Nitrogen_Cycle.svg
Nitrogen in the Septic Tank of a Septic System: In raw wastewater (i.e., wastewater that has not yet entered a septic tank), approximately 73% of N is present as organic-N and 24% exists as ammonium-N (Table 2; Lowe et al. 2007). Due to anaerobic (lack of oxygen) conditions in the conventional septic tank, raw wastewater is partially treated in the septic tank. The resulting fluid is called septic tank effluent (i.e., wastewater that has passed through the septic tank but has not entered the drain field). This partial or primary treatment in the septic tank results in settling of solids at the bottom of the tank and degradation and separation of solids from liquids. During this process, much of the organic N is mineralized (converted) to ammonium; this process is called ammonification. As a result, N in the final septic tank effluent is 70%–90% ammonium-N and 10%–30% organic-N (Heatwole and McCray 2007; Lowe et al. 2007).
Nitrogen in the Drain Field of a Septic System: The effluent that flows from the septic tank into a drain field contains most of the N as ammonium or in organic forms, as described above. It is important to note that soils have very little capacity to adsorb N, so transformations that remove N from the soil are important. In the unsaturated part of the drain field, forms of N present in septic tank effluent undergo several possible transformations, as described below and illustrated in Figure 2:
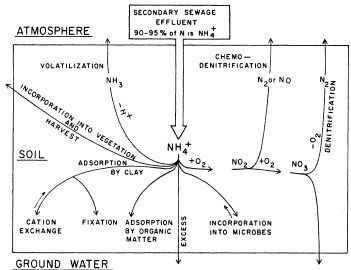
Credit: Lance (1972)
Organic-N. Organic-N added with the effluent is typically converted to ammonium-N through the process of ammonification, which may take place in either aerobic or anaerobic soil conditions. Once organic-N has been converted to ammonium-N, the fate of the ammonium-N will occur along one or more of the pathways described below.
Ammonium-N. Ammonium in the drain field environment will most likely be converted to nitrate-N through nitrification, though it may undergo any of the following transformations (Figure 2):
- Nitrification—This process is the conversion of ammonium to nitrate; it is most likely to occur as long as oxygen is present, such as in the unsaturated zone of soil, where water does not yet fill all of the soil pores such that some pores are still air-filled. Nitrification occurs in the initial 1 foot (30 cm) of unsaturated soil below the drain field and proceeds to completion or near completion as long as at least 2 feet of unsaturated soil is present. Once the soil becomes saturated (all soil pores are filled with water), then the oxygen-rich (aerobic) conditions necessary for nitrification are no longer present.
- Plant uptake—This is limited as most drain fields are placed below the root zone of plants.
- Volatilization—This is the conversion to ammonia (NH3) gas that escapes to the atmosphere, and it only occurs in alkaline (pH >8) soils. It can also occur in the septic tank.
- Adsorption—Ammonium can be adsorbed on to negatively charged sites on soil particles or organic matter.
- Microbial uptake—This is a continuous, steady state process whereby microorganisms use N for their metabolism and incorporate N into their biomass.
Nitrate-N. Nitrate forms in the soil through nitrification and has the following possible fates in the soil environment:
- Leaching—Because nitrate ions have a negative charge, they are not held by soil particles. As a result, nitrate is highly mobile in soil, so it moves easily downward through the soil toward groundwater.
- Denitrification—This process is the conversion to nitrogen gas (N2) and requires anoxic conditions (low oxygen). It generally occurs when the soil is nearly saturated with water (i.e., at or near the water table) so that low-oxygen conditions prevail.
- Plant or microbial uptake—Nitrate can accumulate in microbial biomass or can be taken up by plants; plant uptake is limited since most drain fields are located below the root zone of plants.
Note that the last transformation—denitrification—will occur only if nitrification of ammonium-N happens first and if soil conditions allow it. This highlights the importance of at least 2 feet of unsaturated soil below the drain field. While plant uptake of ammonium and/or nitrate is not a major N removal pathway, the chances of this process taking place can be increased if the drain field is closer to the surface (i.e., within the plant root zone).
Nitrogen and Water Quality
The fate of N in septic systems is important because of the role of N as a potential contaminant in groundwater and surface water. A quantity as little as 1 mg/L of total N has been shown to lead to algae growth in Florida's springs (Hazen and Sawyer 2009). If concentrations are greater than 10 mg/L, nitrate-N is a drinking water concern because of its negative impacts on public health. Thus, knowing the contribution of septic systems as a source of N in groundwater and surface water pollution is important.
Research shows that approximately 10%–50% percent of septic tank effluent total N may be adsorbed or otherwise removed during flow through water-unsaturated soil before the effluent reaches groundwater (Hazen and Sawyer 2009). Most often, however, N derived from septic systems is converted to nitrate by the process of nitrification. Unless denitrification takes place, the most likely fate of this nitrate is leaching to groundwater. Moreover, as nitrate leaches through the soil, it does not interact with soil components under aerobic conditions. It can travel through the unsaturated soil zone to groundwater, and water quality surveys throughout the United States have identified local and regional contamination of groundwater and surface water by nitrate derived from septic systems. In some cases, these studies have detected nitrate-N concentrations exceeding the allowable groundwater level of 10 mg/L at considerable distances from septic systems' drain fields (Beal et al. 2005).
Removal of total N from an unmodified conventional drain field is not usually efficient. In a study in a Florida Karst area, Harden et al. (2008) observed that septic tanks were especially limited in their ability to remove or attenuate nitrate due to unsaturated sandy surface soils, little opportunity for denitrification, and the subsequent gaseous loss of N from the system. In an earlier study, Giblin and Gaines (1999) found that conventional septic systems were the leading cause of groundwater nitrate contamination in a coastal community with sandy soils. They also attributed elevated groundwater nitrate-N to limited opportunities for denitrification in the low-organic matter sandy soil.
In another study in the Florida Keys, Lapointe et al. (1990) reported a 400-fold increase in inorganic N (ammonium, nitrate, and nitrite) concentrations in groundwater beneath septic systems as compared to groundwater under a control area (no septic systems). These authors also noted that season of the year controlled the fate of septic tank-derived N. There was a twofold increase in N transport from groundwater to surface water during the summer, likely because of increased hydraulic head (more water) during the rainy season. Likewise, Meeroff et al. (2008) observed increased wastewater-derived nitrate concentrations in both groundwater and surface water during months of a seasonal high water table, indicating that the septic systems were less efficient at removing N during certain times of the year.
Anderson (1998) studied nitrate-N fate and transport in the Indian River Lagoon Basin of Florida and found that nitrate levels under septic systems were significantly elevated over background levels, but that by 15 meters down gradient of the septic system, nitrate levels were at or near background levels. He attributes nitrate reductions to natural denitrification in the groundwater and adds that this level of denitrification can be expected in typical Florida soils with a high groundwater table. If this is the case, proper siting of the drain field can be a major determining factor in how well nearby surface waters and groundwater can be protected.
Where groundwater recharges stream flow, nitrate-N-enriched groundwater can contribute to eutrophication, a process that increases algae growth, especially of the blue-green type. Accumulated algal biomass can lead to the death of aquatic life because of excessive oxygen demand. While not directly toxic to fish, nitrate can have an indirect effect by contributing to eutrophication.
Summary
Nitrogen is a major constituent of household wastewater that is discharged in septic systems. Total N concentration in septic tank effluent is about 60 mg/L. Because excess N can impair water quality and human health, it is important to monitor and characterize N transport from septic systems. Transformations, retention, loss, and movement of N in natural soil systems are governed by the mechanisms of ammonification, nitrification, denitrification, adsorption, biological uptake, and volatilization. Research shows that approximately 10%–50% of septic tank effluent N may be removed during flow through water-unsaturated soil before the effluent reaches groundwater. Most often, however, N is converted to nitrate during either the treatment phase or in the soil drain field by the process of nitrification. Soils cannot adsorb nitrate, so it can migrate to groundwater, where it is a documented source of groundwater contamination. Denitrification can permanently remove nitrate from the system, making it the best means of removing N from septic systems. However, it only occurs in soil and groundwater under proper conditions.
References
Anderson, D.L. "Natural Denitrification in Groundwater Impacted by Onsite Wastewater Treatment Systems." Proceedings of the Eighth National Symposium on Individual and Small Community Sewage Systems, Orlando, FL, March 8–10, 1998.
Arthur, J.D., H.A.R. Wood, A.E. Baker, J.R. Cichon, and G.L. Raines. "Development and Implementation of a Bayesian-based Aquifer Vulnerability Assessment in Florida." Natural Resources Research 16 (2007):93–107.
Beal, C.D., E.A. Gardner, and N.W. Menzies. "Process Performance and Pollution Potential: A Review of Septic Tank-soil Absorption Systems." Australian Journal of Soil Research 43 (2005):781–802.
Florida Department of Health (FDOH). "Basic Concepts in Wastewater Treatment." Accessed June 6, 2011. http://www.floridahealth.gov/environmental-health/onsite-sewage/training/_documents/a-basic-concepts.pdf.
Giblin, A.E., and A.G. Gaines. "Nitrogen Inputs to a Marine Embayment: The Importance of Groundwater." Biodegradation 10 (1999):309–28.
Harden, H.S., E. Roder, M. Hooks, and J.P. Chanton. "Evaluation of Onsite Sewage Treatment and Disposal Systems in Shallow Karst Terrain." Water Research 42 (2008):2585–97.
Hazen and Sawyer Environmental Engineers and Scientists. "Literature Review of Nitrogen Reduction Technologies for Onsite Sewage Treatment Systems." Task A.2 Final Report prepared for the Florida Department of Health (2009). Accessed June 6, 2011. http://www.floridahealth.gov/environmental-health/onsite-sewage/research/_documents/nitrogen/task-a-lit-review.pdf.
Heatwole, K.K., and J.E. McCray. "Modeling Potential Vadose-zone Transport of Nitrogen from Onsite Wastewater Systems at the Development Scale." Journal of Contaminant Hydrology 91 (2007):184–201.
Hossain, F., N. Chang, M. Wanielista, Z. Xuan, and A. Daranpob. "Nitrification and Denitrification in a Passive On-site Wastewater Treatment with a Recirculation Filtration Tank." Water Quality, Exposure, and Health 2 (2010):31–46.
Lance, J. C. "Nitrogen Removal by Soil Mechanisms." Water Pollution Control Federation 44 (1972):1352–61.
Lapointe, B.E., J.D. O'Connell, and G.S. Garrett. "Nutrient Couplings between On-site Sewage Disposal Systems, Groundwaters, and Nearshore Surface Waters of the Florida Keys." Biodegradation 10 (1990):289–307.
Lowe, K.S., M.B. Tucholke, J.M.B. Tomaras, K. Conn, C. Hoppe, J.E. Drewes, J.E. McCray, and J. Munakata-Marr. "Influent Constituent Characteristics of the Modern Waste Stream from Single Sources." Water Environment Research Foundation. Technical Report 04-DEC-01, 2009.
Lowe, K.S., N.K. Rothe, J.M.B. Tomaras, K. DeJong, M.B. Tucholke, J.E. Drewes, J.E. McCray, and J. Munakata-Marr. "Influent Constituent Characteristics of the Modern Waste Stream from Single Sources: Literature Review." Water Environment Research Foundation. Technical Report 04-DEC-1a, 2007.
Meeroff, D.E., F. Bloetscher, T. Bocca, and F. Morin. "Evaluation of Water Quality Impacts of On-site Treatment and Disposal Systems on Urban Coastal Waters." Water Air Soil Pollution 192 (2008): 11–24.
Oakley, S.M., A.J. Gold, and A.J. Oczkowski. "Nitrogen Control through Decentralized Wastewater Treatment: Process Performance and Alternative Management Strategies." Ecological Engineering 36 (2010):1520–31.
Robertson, W.D., and J. Cherry. "Hydrogeology of an Unconfined Sand Aquifer and its Effect on the Behavior of Nitrogen from a Large-flux Septic System." Hydrogeology Journal 1 (1992):32–44.
Toor, G.S., M. Lusk, and T. Obreza. Onsite Sewage Treatment and Disposal Systems: An Overview. Gainesville: University of Florida Institute of Food and Agricultural Sciences. https://edis.ifas.ufl.edu/ss549 (April 2017)
US EPA. "Water Treatment/Disposal for Small Communities." EPA/625/R-92/005 U.S. Environmental Protection Agency, Office of Research and Development, Center for Environmental Research Information, Cincinnati, OH, 1992.
US EPA. "EPA Guidelines for Management of Onsite-decentralized Wastewater Systems." USEPA Report 832-F-00-012, 2000.
Wilhelm, S.R., S.L. Schiff, and W.D. Robertson. "Chemical Fate and Transport in a Domestic Septic System: Unsaturated and Saturated Zone Geochemistry." Environmental Toxicology and Chemistry 13 (1994):193–203.