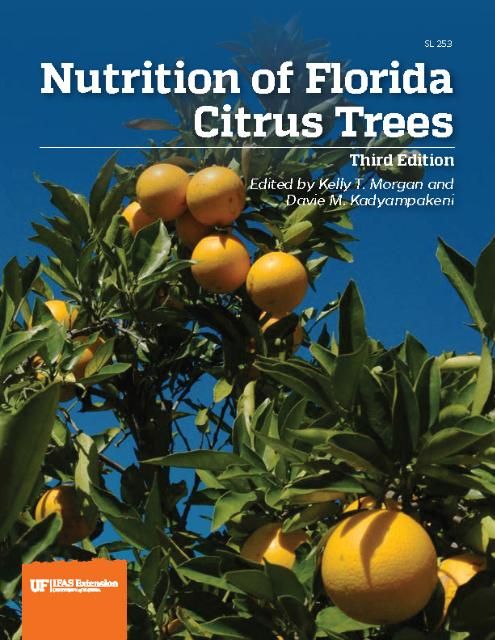
This publication is part of SL253, Nutrition of Florida Citrus Trees, 3rd Edition. For references, a glossary, and appendices, please refer to the full document at https://edis.ifas.ufl.edu/ss478.
Information in the box below applies to citrus trees affected by HLB. Other information in this chapter is valid for healthy citrus trees and trees with HLB.
Recommendations for HLB-affected trees:
Citrus Water Requirements—Kelly Morgan, Davie Kadyampakeni, Said Hamido, and Mongi Zekri
With HLB, irrigation scheduling is critical. HLB-affected trees cannot afford water stress or water excess. Current UF/IFAS citrus irrigation recommendations estimate citrus tree water requirements for mature trees based on data collected prior to introduction of HLB into Florida. Citrus trees affected by HLB are known to lose substantial foliage and up to 80% of the root mass depending on disease severity, thus negatively influencing water and nutrient uptake.
Premature fruit drop is increased if water stress is experienced by citrus trees and canopy size is reduced as is the number of fruit and fruit size. Benefits of proper irrigation scheduling include reduced loss of nutrients through leaching due to excess water applications and reduced pollution of groundwater or surface waters.
A study was conducted in a Florida commercial citrus grove from 2011 to 2015 with the objective of determining irrigation requirements of HLB-affected citrus trees compared with healthy trees. Results from the field study indicated that healthy trees consumed approximately 25% more water than HLB-affected trees. Reduced water uptake by HLB-affected trees resulted in significantly greater soil water content. The relationship between leaf area and water uptake indicated that diseased trees with lower canopy density and corresponding lower leaf area index take up less water and consequently less nutrients from the soil. The elevated soil water content may partially explain higher rates of root infection with Phytophthora spp. observed in some HLB-affected trees.
Improvements in Scheduling and Soil Moisture Measurement—Kelly Morgan, Davie Kadyampakeni, Rhuanito Soranz Ferrarezi, and Mongi Zekri
Irrigation must be managed to allow growers to maintain or increase crop production without depletion of water resources. Increase in water-use efficiency is achieved by selecting a proper irrigation scheduling method and application timing. Proper irrigation scheduling applies an appropriate volume of water to a citrus grove at the appropriate time based on tree need, soil properties, and weather conditions.
Two three-year studies were conducted in three commercial citrus groves to compare soil moisture sensor and ET-based irrigation schedules on tree growth, yield, and water use. Results from the first study indicated water use managed with soil moisture sensors and ET-based models were similar and reduced average monthly water use by approximately 14% of the conventional irrigation practice without reducing yields. The second study aimed to determine proper irrigation scheduling for HLB-affected trees. The second experiment was conducted in three commercial groves on Ridge and Flatwoods soils. Irrigation schedules consisted of current UF/IFAS ET-based recommendations, daily irrigation, and an intermediate schedule, all using the same amount of water on a monthly and annual basis. The UF/IFAS schedule was determined weekly using the Citrus Irrigation Scheduler found at the Florida Automated Weather Network (FAWN) website (https://fawn.ifas.ufl.edu/tools/irrigation/citrus/scheduler/), and resulted in irrigation schedules ranging from daily in May to every 10–14 days in the winter months from November to February. Daily irrigation schedules were determined by dividing the UF/IFAS irrigation duration by the number of days between irrigations. "Intermediate" irrigation was half the UF/IFAS interval for half the time.
Daily irrigation increased tree water uptake and soil water content compared with intermediate and UF/IFAS schedules. Daily and intermediate irrigation increased canopy density (as measured by leaf area index) compared with the UF/IFAS schedule. Fruit drop per square foot of the under-canopy area was lower for daily irrigation schedules in the second year of the study, but yields were similar among all irrigation schedules.
These studies show that for HLB-affected trees, irrigation frequency needs to be increased and amounts of irrigation water per application decreased to minimize water stress from drought or excess water, while ensuring optimal water availability in the root zone at all times. It is recommended that growers maintain soil moisture in the root zone (top 3 feet for Ridge and 18 inches for Flatwoods soils) using soil moisture sensors or irrigation apps. The FAWN and SmartIrrigation apps provide the option of daily irrigation schedules. As noted above, HLB-affected trees with lower canopies use less water than do healthy trees. Therefore, if the irrigation scheduling app is used, the irrigation time should be reduced by 10% to 20%. For example, if the app suggests an irrigation time of 1 hour, this time could be reduced by 6 to 12 minutes for HLB-affected trees.
References
Hamido, S. A., K. T. Morgan, and R. C. Ebel. 2017. "Improved Irrigation Management of Sweet Orange with Huanglongbing." HortScience 52: 916–921.
Hamido, S. A., K. T. Morgan, and D. M Kadyampakeni. 2017. "The Effect of Huanglongbing on Young Citrus Tree Water Use." HortTechnology 27: 659–665.
Kadyampakeni, D. M., and K. T. Morgan. 2017. "Irrigation Scheduling and Soil Moisture Dynamics Influence Water Uptake by Huanglongbing Affected Trees." Scientia Horticulturae 224: 272–279.
Water Supply
Competition for water is increasing in all citrus production areas. Florida's expanding population increases water demand in the urban sector, which reduces water availability for agriculture. Growing a high-quality citrus crop is water-intensive; however, growers do have options to remain competitive. By increasing water uptake efficiency (the amount of water taken up by the trees compared with the amount of water applied to the grove), growers can continue to achieve normal production while reducing water withdrawals.
If more water is applied than the soil can hold, it drains below the root zone and is wasted. Nutrients, especially N, move with water as it passes through the soil (leaching), either downward to groundwater or laterally toward ditches and canals. When nutrients are leached, they are no longer available to the trees and may become an environmental concern. Understanding how water and nutrients move through the soil is important in improving their use.
Production Region Characteristics Important to Irrigation Management
The central Florida Ridge (Chapter 2, Fig. 7) features well-drained, sandy Entisols (Appendix A). These soils permit rapid infiltration of rain and irrigation water, making them vulnerable to nutrient leaching. Nitrate leaching is a major concern to citrus producers on the Ridge. The maximum irrigation depth to wet the majority of the root zone in these soils is 3 to 4 ft.
The Gulf, Peace River, and Indian River citrus production areas are dominated by poorly drained Flatwoods soils (Chapter 2, Fig. 7) that require artificial drainage to produce high-quality citrus. Nitrate leaching is greatly reduced in Flatwoods soils compared with the Ridge. The citrus root zone in these soils is typically 18 inches or less.
Nutrient Uptake Efficiency
Improving water uptake efficiency will also improve nutrient uptake efficiency (the amount of nutrients taken up by the plant compared with the amount of nutrients applied as fertilizer). In Florida's sandy soils, nutrient and water uptake efficiencies are linked. Management methods that improve irrigation water uptake efficiency will increase the proportion of applied nutrients that are taken up by the tree, potentially leading to increased growth and yield.
Allowable Soil Water Depletion
The following terms are important for developing a workable and efficient irrigation schedule:
- Field Capacity
This is the amount of water remaining in saturated soils 2–3 days after free drainage water has been removed by the downward forces of gravity. This value of field capacity assumes that the water removed from the soil profile is only removed by gravity, not through plant transpiration or soil evaporation. The matric potential at this soil water content is around -1/10 bar.
2. Permanent Wilting Point
This is the water content of a soil when plants growing in that soil wilt and fail to recover upon watering. It is when the volumetric water content is too low for the plant to remove water from the soil. The matric potential at this soil water content is commonly estimated at -15 bars.
3. Plant-Available Water
This is the portion of water that can be absorbed by plant roots. It is the amount of water available between field capacity and the permanent wilting point.
As soil dries out, water becomes increasingly more difficult for trees to remove, which can eventually cause water stress. Tree health and yield will suffer if the soil is allowed to get too dry. To provide adequate water for flowering, fruit set, and vegetative growth, maximum soil water depletion should be no more than 25% to 33% of available water from February to June. Once the rainy season starts, the maximum depletion level can be increased to 50% of available water. This additional allowable depletion increases the capacity of the soil to hold rainfall without leaching nutrients. The same depletion levels in the fall and winter months will save water without reducing yield.
Irrigation Scheduling
Despite our large yearly rainfall of 50–60 inches, which exceeds the citrus water requirement or evapotranspiration (ET), Florida citrus growers and production managers should keep in mind that they cannot grow citrus successfully and competitively without supplemental irrigation. Through research and field experience, we know that irrigation is necessary because of the non-uniform distribution of the rainfall and the very limited water-holding capacity of Florida sandy soils. Irrigation is of particular importance during the dry period (February–May), which coincides with the critical stages of leaf expansion, bloom, fruit set, and fruit enlargement. Citrus production managers should accurately determine when and for how long to irrigate. With proper irrigation scheduling, yield will not be limited by water stress. Any degree of water stress or imbalance can produce a deleterious change in physiological activity of growth and production of citrus trees. The number of fruit, fruit size, and tree canopy are reduced, and premature fruit drop is increased with water stress. Extension growth in shoots and roots and leaf expansion are all negatively impacted by water stress. Other benefits of proper irrigation scheduling include reduced loss of nutrients from leaching as a result of excess water applications and reduced pollution of groundwater or surface waters from the leaching of nutrients and other chemicals.
Successful irrigation management maintains sufficient water and nutrients in the root zone to maximize plant growth and health. Growers who focus on improving water and nutrient uptake efficiency will reduce N and P losses and decrease environmental impacts at the same time. While some nutrient loss is unavoidable due to excess rainfall, loss due to management decisions can be minimized.
Proper irrigation scheduling applies an appropriate volume of water to a citrus grove at the appropriate time based on tree need, soil properties, and weather conditions. Scheduling methods include:
- Experience
- Calendar method (e.g., 0.8 inches every 4th day)
- Monitoring soil water status
- Calculating a water budget
Soil Water Measurement
Experience or the calendar method can provide a reasonably good irrigation schedule, but they are not accurate enough to maximize water uptake efficiency and prevent nutrient leaching. Using soil moisture sensors improves accuracy because they quantify changes in soil water status. These devices may be fixed in one location, portable, or handheld. They may measure soil moisture at one depth or at multiple depths. General categories include:
- Tensiometers
- Electrical resistance blocks
- Time domain reflectometry (TDR) probes
- Capacitance probes
Tensiometers are simple, easy to manage, and inexpensive devices that determine water status in terms of the soil-water tension the plants are experiencing. They are used successfully in determining the need for irrigation when the soil water content is being kept near field capacity. It has been shown that their use could result in avoiding tree stress and excessive water applications. A tensiometer consists of a porous cup, generally of ceramic material, connected through a water-filled tube to a vacuum gauge. When the cup is placed in the soil where the suction measurement is to be made, the bulk water inside the cup comes into hydraulic contact and tends to equilibrate with the soil water through the pores of the ceramic walls. The suction is indicated by the vacuum gauge. As soil water is depleted by drainage or plant uptake, or as it is replenished by rainfall or irrigation, corresponding changes on the tensiometer's gauge occur. Tensiometers cease to function at soil suctions above 0.85 bar due to air entering the system. Tensiometers are easy to use but may give faulty readings if they are not installed properly and maintained regularly.
Electrical resistance blocks. The most common indirect field device for metric potential or metric pressure measurement is the porous conductivity block. This method is based on the fact that the electrical resistance of certain porous materials, such as gypsum, nylon, and fiberglass, is related to their water content. It involves burying a small block containing a pair of electrodes surrounded by a porous matrix and running the lead wires to a resistance bridge. The water in the block reaches matric potential equilibrium with that in the soil, and the resistance measured at the bridge gives the electrical conductivity of the solution between the electrodes. Because of the pore size of the material used in most electrical resistance blocks, particularly those made of gypsum, the water content and thus the electrical resistance of the block does not change dramatically at suctions less than 0.5 bar. Therefore, resistance blocks are best suited for use in fine-textured soils, such as silts and clays. Electrical resistance blocks are not reliable for scheduling irrigation in sandy soils. These devices also need to be recalibrated more frequently because they are sensitive to temperature and can deteriorate in the soil.
Time-Domain Reflectometry (TDR) is widely used to measure soil water content and bulk electrical conductivity. A TDR instrument has a device capable of producing a series of precisely timed electrical pulses with a wide range of high frequencies used by different devices, which travel along a transmission line (TL) that is built with a coaxial cable and a probe. The TDR probe usually consists of 2 parallel metal rods that are inserted into the soil acting as waveguides. At the same time, the TDR instrument uses a device for measuring and digitizing the energy (voltage) level of the TL. When the electromagnetic pulse traveling along the TL finds a discontinuity (i.e., probe-waveguides surrounded by soil), part of the pulse is reflected. This produces a change in the energy level of the TL. The advantages of TDR over other soil water content measurement methods are high accuracy, minimal soil disturbances, no need for soil-specific calibration, a lack of radiation hazard associated with neutron probe or gamma-attenuation techniques, and automation and multiplexing, which allow it to provide continuous measurement. TDR systems are relatively expensive.
Capacitance probe systems consist of a solar-powered, central logging facility connected by cable to probes fitted with capacitance sensors. The sensors utilize electrical capacitance to measure the complex dielectric constant of the soil and water medium. The changing ratio of air to water at each soil depth can be measured very quickly and accurately. The sensor readings are converted to volumetric soil water depth content using calibration equations. Soil water content data are downloaded to a computer and displayed in easy-to-read graphs that directly reflect crop water use and irrigation needs. Volumetric soil water content at each sensor is expressed as either a percentage or a depth of water in mm water per 10 cm of soil. Multisensor capacitance probes were demonstrated to be a highly successful and accurate technique in measuring real-time soil water dynamics for irrigation scheduling. Capacitance probe systems are relatively expensive.
Considerations when using soil moisture sensors to schedule irrigation include:
- Knowing the soil water-holding capacity and tree root zone depth (Appendix A).
- Placing sensors where the majority of roots are located, such as at the dripline of the tree.
- Using multiple sensors, both across the grove and with depth, to fully characterize the tree root zone.
- Moving sensors to follow root growth as the tree canopy expands in developing groves.
- Basing irrigation on the soil depth containing the greatest root density.
- Managing root zone soil moisture between field capacity and the maximum allowable available water depletion (one-fourth to two-thirds depletion, depending on time of year).
Figure 1 shows an example of how a multilevel capacitance probe could be used to adjust an irrigation schedule to maximize water uptake efficiency and minimize nutrient leaching. The four graphed lines represent soil moisture content at 4-inch (red), 8-inch (blue), 12-inch (purple), and 20-inch (green) depths in the soil and the x-axis shows a 16-day time period separated into 2-day increments.
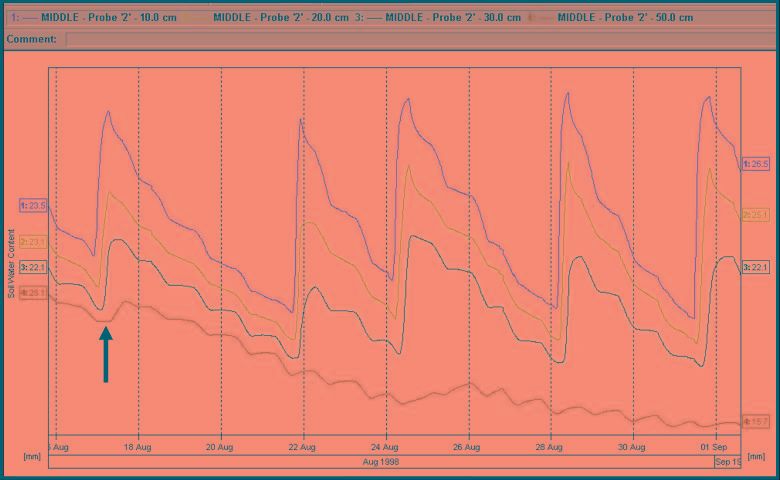
The effect of irrigation is easily observed as sharp increases in soil moisture at the 4-, 8-, and 12-inch depths. However, note that the first irrigation increased soil moisture at the 20-inch depth as well (black arrow), which is below the zone of highest root density. Because the goal was to keep the irrigation water in the top 18 inches of soil, the grower reduced the duration of subsequent irrigations. The steadily decreasing water content at 20 inches during the following 2-week period shows that the grower had attained optimum irrigation water management.
Water Budgeting
An alternative method to schedule irrigation uses a computer program that estimates tree water consumption (evapotranspiration, or ET) from weather data. Reference ET and convenient irrigation scheduling management tools for all Florida citrus production regions can be found on the Florida Automated Weather Network website at https://fawn.ifas.ufl.edu/. The computer program uses the soil water-holding capacity of specific soil series to determine field capacity. Irrigation schedules are determined using the strategies and equations described below.
Smartphone Apps
Mobile smart devices (e.g., smartphones, tablets) have become popular because of convenience and ease of use, making them ideal for disseminating information on a regular basis with real-time data. Tools developed for use on mobile smart devices are typically called "apps" and are available for a variety of functions.
Due to the increasing popularity of smartphones and apps, FAWN developed an app for the iPhone and Android platforms that allows users to view data from grower-owned weather stations, provided as a cost share from the Florida Department of Agriculture and Consumer Services, on their smartphones, in much the same way that the data can be viewed on the FAWN webpage.
UF/IFAS has also developed smartphone apps for crop irrigation scheduling using FAWN weather data. The FAWN and irrigation scheduling apps are available to download in the App Store and Play Store at no cost. The goal is to provide users with an easy-to-use mobile app to access information to improve irrigation scheduling for a wide range of crops, including citrus. By using the app instead of a set time-based schedule for irrigation, users achieve accurate irrigation. The irrigation scheduling app has the potential of reducing water and fertilizer use, resulting in reduced irrigation and fertilizer costs and possibly reduced nutrient leaching.
Irrigation Strategies to Improve Nutrient Uptake and Reduce Leaching
Developing an irrigation strategy to reduce nutrient leaching has the objective of not applying more irrigation water than the root zone can hold. Considering the low water-holding capacity of citrus grove soils, this objective is very difficult to accomplish, even for the most experienced and diligent irrigation managers. The major questions to be answered in this procedure are:
- How much water can the root zone hold?
- What is the maximum irrigation system run time before leaching occurs?
Example
A central Ridge citrus grove has the following characteristics:
- Tree spacing: 12½ ft within the row and 25 ft between rows.
- Tree canopy diameter: 17½ ft
- Root zone depth: 3 ft.
- One 16 gal/hr microsprinkler per tree with a 16-foot diameter wetted pattern.
- The citrus root zone is continuous from tree to tree, existing both inside and outside of the wetted pattern.
- The irrigated system wets approximately 60% of the total root zone (Figure 2).
Nutrient leaching risk in this grove is higher within the wetted pattern due to potential overirrigation plus the fact that most fertilizers are applied to that zone (Figure 3). A good irrigation manager will control this risk with careful water management.
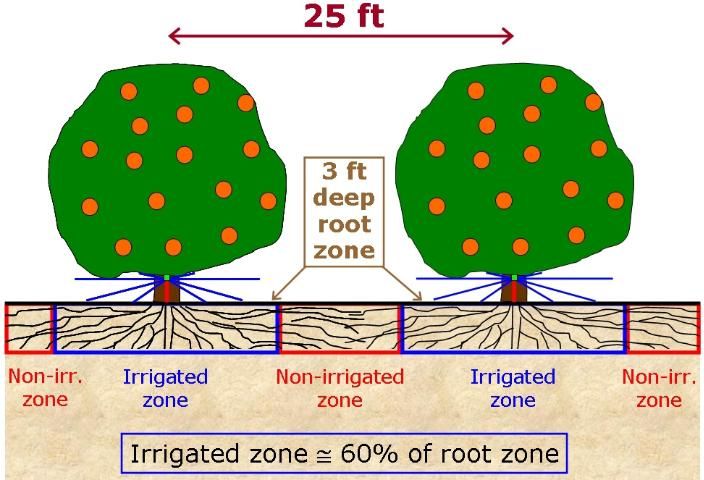
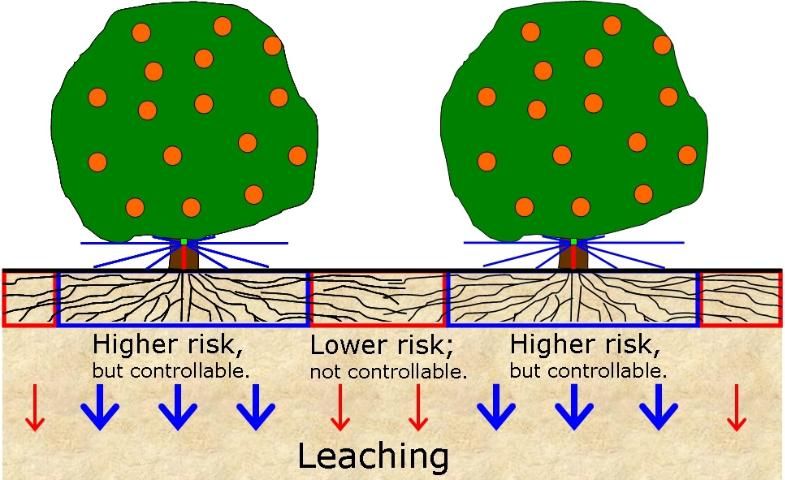
Note: The following depictions of water content changes in the citrus tree root zone (Figures 4 to 7) do not represent the actual water extraction pattern. The blue shading shows 1) approximately where water extraction occurs beneath the canopy, and 2) the relative soil water content with respect to available soil water-holding capacity.
This example starts with the entire grove at field capacity moisture content following a heavy rain (Figure 4). The citrus trees begin to remove water from the soil in response to the atmospheric ET demand. After several days have passed (depending on time of year), the water content in the root zone decreases to 50% of available water capacity (Figure 5).
At this point, the grove manager turns on the irrigation system and operates it long enough to return the soil in the wetted pattern back to field capacity (Figure 6). From this point until the next significant rainfall, only the soil water content in the irrigated zone can be influenced by the irrigation manager. The water content in the non-irrigated zone rapidly decreases to the point where little to no soil water can be extracted by the tree.
If the grove manager operates the irrigation system too long and applies more water than the soil can hold, water will move beneath citrus tree roots. If water-soluble nutrients like nitrate or potassium are present in the irrigated zone during the irrigation period, a portion will leach (Figure 7).
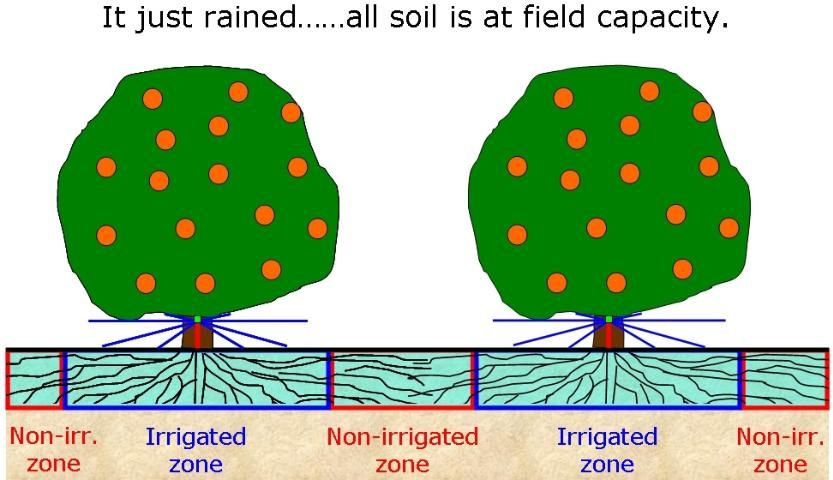
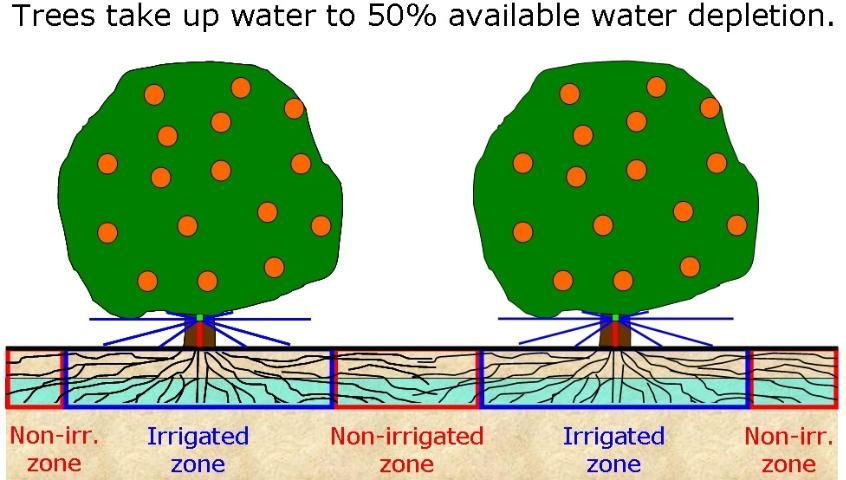
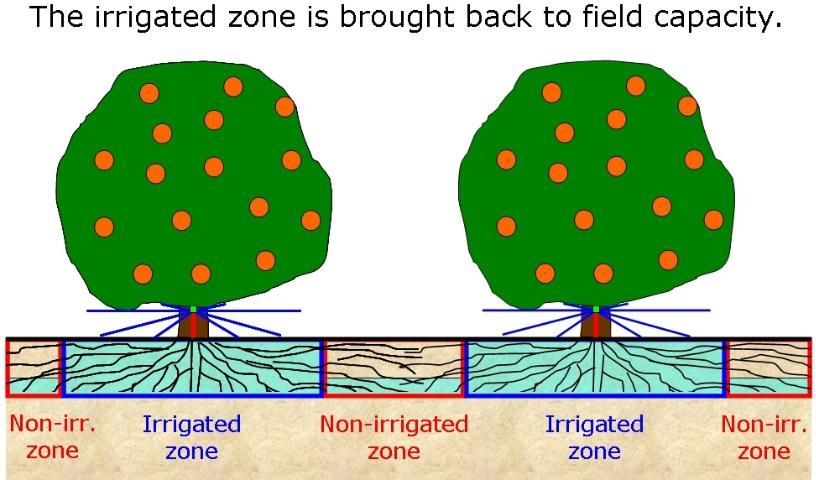
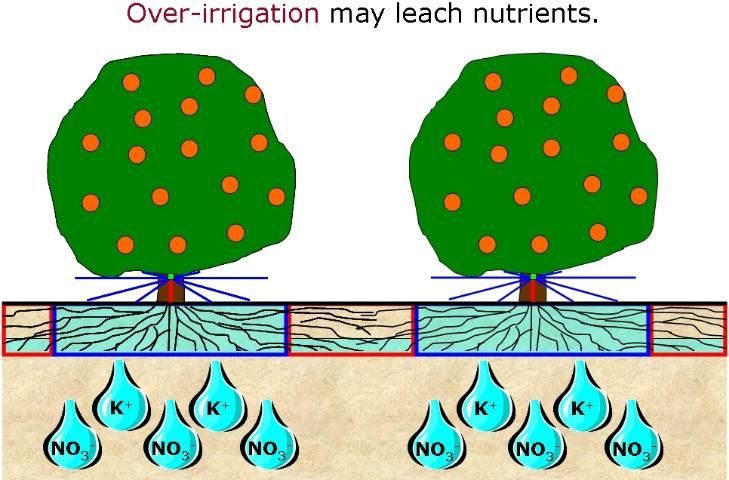
How much water can the root zone hold?
From Appendix A, Table 26:
- Central Ridge soils: 0.3 to 0.7 inches/ft
- Flatwoods soils: 0.3 to 1.2 inches/ft
Calculations
- Volume of water the root zone can hold: 0.6 inches/ft x 3 ft deep root zone = 1.8 inches
- Volume of water to refill at maximum depletion: 1.8 inches x 50% = 0.9 inches
- Volume of water this represents per tree space: 0.9 inches/tree x 1 ft/12 in x (25 ft x 12½ ft) x 7.5 gal/cu ft x 60% coverage = 105 gal/tree
- Maximum system run time: 105 gal ÷ 16 gal/hr emitter flow rate = 6.6 hr
- Adjust for system delivery efficiency of 90%: 6.6 hr ÷ 0.9 = 7.3 hr
Therefore, the irrigation system should never be run longer than about 7 hours for any single cycle, provided that the available soil water is at least 50% depleted when the irrigation begins. If the soil is less than 50% depleted of available water, then the maximum run time decreases accordingly.
Considerations
Ideal maximum system run time vs. practical field management. There may be management limitations that prevent stopping irrigation at or before the ideal maximum run time, such as limitations of the irrigation system design or lack of sufficient personnel. Growers should evaluate their overall irrigation management and take corrective action if possible.
Theory vs. reality. Calculating maximum run time from grove and irrigation system characteristics provides a starting point, but the irrigation system or soil may behave differently than the model situation. Thus, growers should fine-tune the maximum run time in the field.