The Fate of Nitrogen Applied to Florida Turfgrass
The quality of Florida's surface and ground waters are of utmost importance to flora and fauna living in these waters. The growth of flora and fauna is directly related to the amount of available nutrients in these waters. Additionally, we use these waters as the primary source of drinking water for ourselves and our families. A wide range of compounds may be found in these waters, the most common of which may be nitrate (NO3-) (Pye et al. 1983). The sources of nitrogen (N) may include, but are not limited to, atmospheric deposition (National Atmospheric Deposition Program 2015), septic tanks (Katz et al. 2010), effluent water disposal (Warneke et al. 2011), agricultural fertilization (Schmidt and Clark 2012), or landscape fertilization (Erickson et al. 2008). Despite evidence to the contrary, some Floridians assume that N applied to turfgrass is a major contributor to water pollution (Shaddox et al. 2016a; Shaddox et al. 2016b; Telenko et al. 2015; Trenholm and Unruh 2005). In order to make informed decisions regarding N applications to turfgrass, it is important to understand the N cycle in the soil/turfgrass system. Therefore, the objective of this publication is to identify and describe the sources and potential fates of N applied to Florida turfgrass.
This discussion will include five paths N may take after being applied to turfgrass: conversion to atmospheric gas, turfgrass uptake, soil storage, leaching, and runoff. However, it is important to first understand turfgrass' contribution to Florida's fertilizer consumption. When discussing Florida's water quality, in particular N contamination, we must consider all the potential sources of N and their relative contributions to groundwater contamination. Florida is uniquely positioned in an environment with optimal sunlight, rainfall, and temperature, which allows for year-round plant growth and crop production. Increased plant growth is often a function of N applications. Nitrogen applications are regarded as essential to sustain the food production necessary to support our population. When all the N fertilizer applied in Florida is considered, the amount applied to turfgrass is comparatively low, contributing only 11% to the total N applied in Florida (FDACS 2017). Although that percentage is low relative to other markets, it is still crucial that we understand the paths that it may take in a turfgrass system. Understanding these fates will help to protect Florida's ecosystem and enhance decisions regarding best management practices.
Atmospheric Nitrogen
More than 99% of all N on planet earth exists in the atmosphere (Havlin et al. 1999) and is chemically and biologically unavailable to plants, except those which are capable of biological N fixation. Approximately 78% of the air we breathe is N2 gas, which can be converted into a useable form (i.e. fertilizer) via the Haber-Bosch process or by biological N fixation. Approximately 80% of the N manufactured via the Haber-Bosch process is used for agricultural fertilizers (Galloway et al. 2008), and it is estimated that the Haber-Bosch process is responsible for supplying the dietary needs of 50% of the human population or 3 billion people (Smil 2001). Thus, second to photosynthesis, the Haber-Bosch process may be the most important process influencing human development over the past century (Erisman et al. 2008). Once harvested from the atmosphere, N applied to turfgrass easily coverts back to a gas either via volatilization or denitrification (Figure 1).
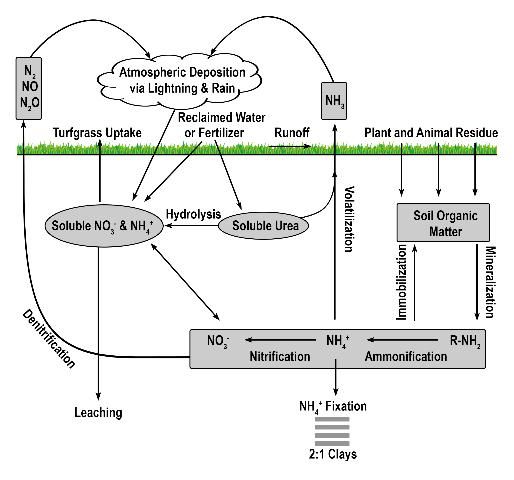
Credit: Travis Shaddox, UF/IFAS
Volatilization is the conversion of N to ammonia (Figure 2). The factors influencing conversion of N to a gas include quantity of soluble N as urea or ammonium, temperature, high soil pH, low soil moisture, and low cation exchange capacity. Nitrogen converted to ammonia is lost to the atmosphere and is no longer available for turfgrass uptake. While volatilization is a distinct disadvantage to the turfgrass, the loss of N as ammonia decreases the amount of N available to move into nearby water bodies via leaching or runoff. However, N volatilization may increase the amount of N returned to the earth via rainfall and atmospheric deposition. Because N is commonly applied to turfgrass as urea, volatilization can be a major contributor to N lost from turfgrass systems, with losses ranging from <1% to as high as 60% of applied N (Goos 2011). This percentage can be reduced by using slow-release urea, urease inhibitors, or by irrigating the turf immediately after fertilization (Franzen et al. 2011). Slow-release N sources are defined as any N source that releases its N at a slower rate compared with a reference soluble N source (AAPFCO 2017). Urease inhibitors slow the conversion of urea to NH4+ by inhibiting urease, the enzyme necessary for urea hydrolysis to occur. In so doing, the rate of volatilization can be reduced by as much as half (Goos 2011). Urease inhibitors may be marketed as "nitrogen stabilizers". Numerous products marketed as urease inhibitors have been tested by land-grant institutions. Only the "nitrogen stabilizers" containing N-(n-butyl) thiophosphoric acid triamide (NBPT) or N-(n-propyl) thiophosphoric acid triamide (NPPT) have consistently reduced volatilization compared with urea alone (Franzen et al. 2011; Goos 2011). Slow-release N fertilizer also reduced volatilization not through urease inhibition, but by delaying the release of urea into the N cycle.
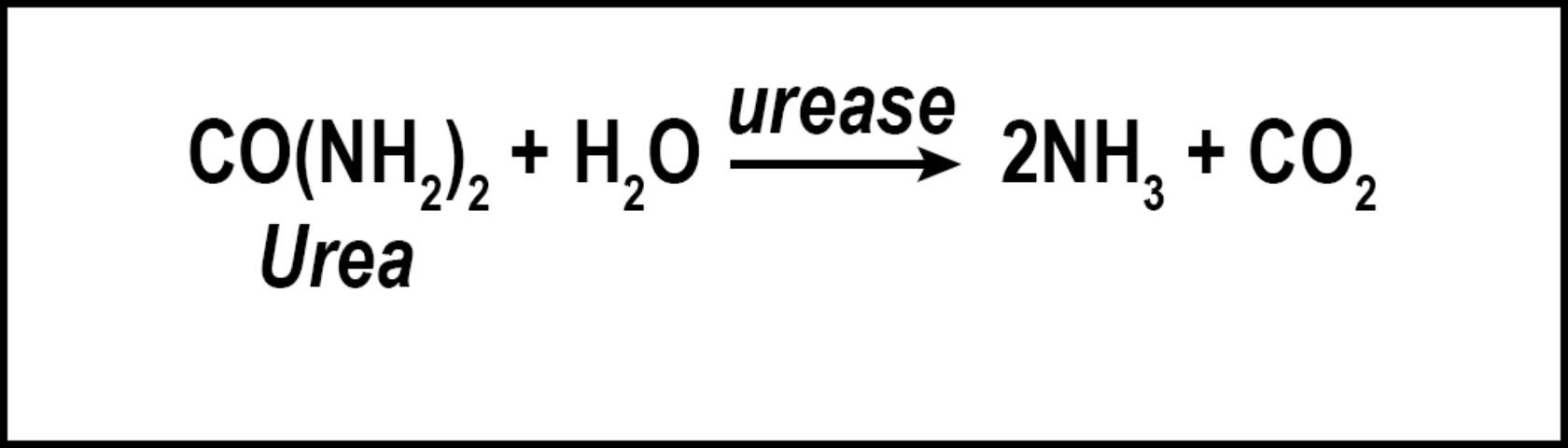
Credit: Travis Shaddox, UF/IFAS
Denitrification is the microbial conversion of NO3- to N2 gas (Figure 3). The conditions that favor denitrification are wet, organic soils containing NO3- (Galloway et al. 2004.)Similar to volatilization, denitrification converts N into one of several N species: nitrite (NO2-), nitric oxide (NO), nitrous oxide (N2O), or gaseous N (N2), reducing the amount of plant-available N and the amount of N available to move to non-target locations. Denitrification requires N to be in the NO3- form, which is then reduced as oxygen is removed. Denitrification is greatly influenced by increased soil moisture, which results in an oxygen-deprived soil and hastens the removal of oxygen from NO3- by denitrifying bacteria. When soil oxygen levels drop below 2%, denitrification is increased. However, denitrification may still occur in aerated soils due to the saturation of internal soil microsites (Carrow et al. 2001). Turfgrass studies designed to determine denitrification rates in Florida are limited. However, in sandy, well-drained soils, denitrification is normally low and accounts for <1% to 5% of applied N, but could approach 94% when temperature exceeds 30°C (Mancino et al. 1988). Denitrification in turfgrass systems compares with other agroecosystems in which 10–40% of applied N may be denitrified (Galloway et al. 2004). Although already low in Florida turfgrass systems, denitrification may be further reduced by using nitrification inhibitors or slow-release N, which may reduce the amounts of NO3-N in the soil. Nitrification inhibitors should contain either 2-chloro-6(trichloromethyl) pyridine (Nitrapyrin) or dicyandiamide (DCD), as these are the only two compounds that have reduced denitrification in field and laboratory studies (Janzen and Bettany 1986; Malzer 1989; Malzer et al. 1989). Similar to their effect on volatilization, slow-release N fertilizers may reduce denitrification by delaying the release of their N into the N cycle. Additional research aimed at determining denitrification rates in Florida turfgrass systems is needed.
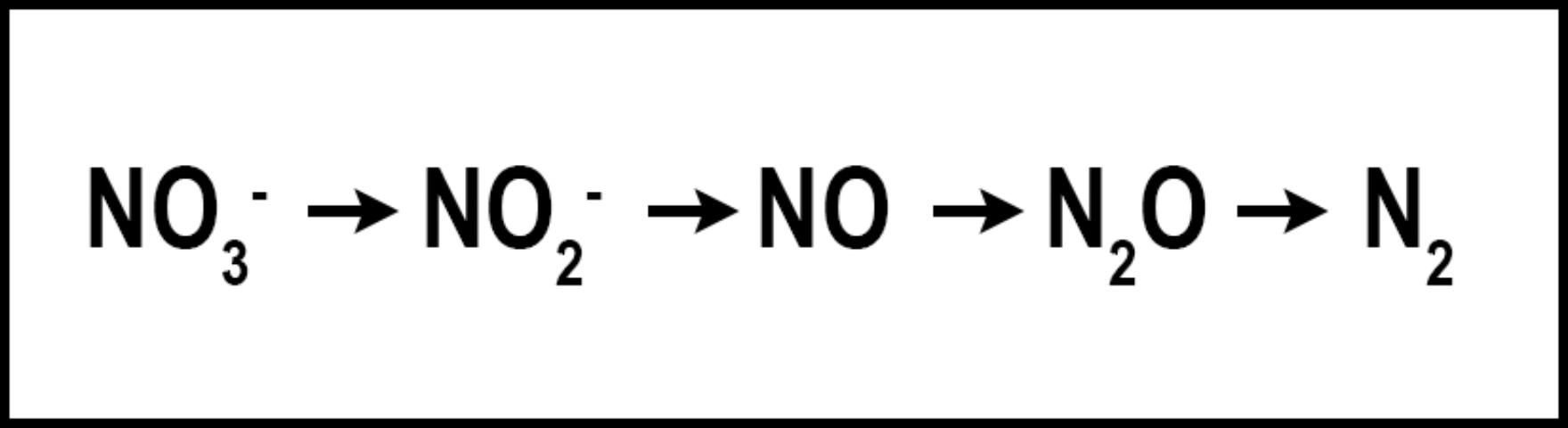
Credit: Travis Shaddox, UF/IFAS
Turfgrass Uptake
The objective of all N applications to turfgrass is sustainable plant uptake and the resulting increase in turfgrass growth or quality. Numerous factors may influence turfgrass uptake of N, including (but not limited to) turfgrass species, season, N type, N rate, and moisture management.
The percent of applied N recovered in turfgrass tissue can vary depending upon turfgrass species. Species that possess a greater density of roots deeper in the soil profile tend to take up greater amounts of applied N compared with turfgrasses with less dense root systems (Bowman et al. 2002). Clearly, greater uptake occurs because a turfgrass with a greater quantity of roots has an increased chance for its roots to intercept and uptake N (Sullivan et al. 2000). St. Augustinegrass and bermudagrass (two of the most common turfgrasses in Florida) have been documented to utilize greater percentages of applied N than centipedegrass, bahiagrass, and zoysiagrass (Bowman et al. 2002). Even cultivars within the same species may also differ in their ability to consume applied N (Young 2015). Although the exact cause is not fully known, it is reasonable to postulate that the differences among cultivars is due to different levels of evapotranspiration (ET), dry matter production, or root masses, which would result in different amounts of applied N being consumed.
Like most plants, the change in climatic seasons can have a dramatic influence on plant growth and nutrient uptake. During the winter in northern Florida, most warm-season turfgrasses will exhibit a decrease in growth and may enter dormancy (a natural turfgrass phase in which the plant is alive, but no cell division or elongation occurs). Even in southern Florida, reduced turfgrass growth will occur during the winter, but true dormancy has not been reported. As turfgrass growth declines, the amount of N needed by the turfgrass also declines. Thus, consumption of applied N can be lower in the winter than in the summer (Wherley et al. 2009). N applications to dormant or semi-dormant turfgrass have not resulted in N leaching unless excessive rainfall occurs (Shaddox et al. 2016a); thus, the applied N will remain in the soil until the plant consumes it or until rainfall/irrigation moves the N beyond the rootzone (Wherley et al. 2009). However, the agronomic advantages to applying N to dormant turfgrasses are low relative to the environmental risk. Thus, N applications to dormant turfgrasses in Florida are not recommended.
Nitrogen fertilizers differ in their form of N and their release characteristics. These differences can lead to different quantities of N absorbed by turfgrass. Nitrogen applied as NH4+ may result in less N uptake than N applied as NO3- due to the tendencies of NH4+ to volatilize and be lost from the soil/turfgrass system (Brown 2003). A larger percentage of N from slow-release N fertilizers may be taken up by the turfgrass compared with soluble N sources (Shaddox 2001). Soluble N is immediately available to follow any of the potential paths in the soil/turfgrass system, including leaching and volatilization, whereas only small portions of N from slow-release N fertilizers become soluble at any given time. To this end, slow-release N fertilizers can increase N uptake by as much as 300% compared with soluble N sources (Shaddox 2001).
A driving factor behind UF/IFAS nutrient recommendations to turfgrass is to apply the amount of N necessary to achieve a desired turfgrass response without applying more N than the turfgrass can consume at any given time. When UF/IFAS recommended N rates are followed, turfgrass uptake of applied N ranges from 40–68% (Brown 2003; Sartain 1985; Shaddox 2001; Stiegler et al. 2011), whereas research conducted outside of Florida indicates the uptake percentage may approach 80% (Bowman et al. 2002). When small quantities of N are applied, very little N has an opportunity to escape turfgrass assimilation. As rates of soluble N increase, the percentage of applied N recovered in turfgrass tissues decreases (Ashley et al. 1965). However, slow-release N sources often require higher application rates compared with soluble N sources in order to achieve the same desired turfgrass response, because only a small portion of the slow-release N will become soluble on a daily basis. Consequently, higher rates of slow-release N sources may result in greater percent uptake of applied N than lower rates (Sartain 1985). Additionally, a single application of slow-release N at a high rate may result in the same N uptake as soluble N applied as a split application (Sartain 2008). Therefore, slow-release N sources may be applied at higher rates than soluble N sources so long as the single application rate and total annual N applied do not exceed UF/IFAS recommendations.
Moisture management greatly influences plant uptake of applied N. Most N is taken up by the plant via the soil solution. Thus, when the soil water content exceeds the soil water holding capacity, N in the soil solution may be moved below the rootzone, which results in reduced plant uptake (Shaddox, 2001). On the other hand, when insufficient water is applied, the turfgrass may enter a state of drought-induced dormancy in which the turfgrass reduces water and N uptake in order to survive (Ashley et al. 1965). Thus, careful consideration should be given to applying sufficient water to maintain acceptable turfgrass, but not applying more water than can be retained by the soil. Generally, rain sensor, soil water sensor, and evapotranspiration controllers apply water more effectively than automatically timed controllers (Dobbs et al. 2014).
Soil Retention, Immobilization, and NH4+ Fixation
The amount of N stored in the soil is dependent upon many factors, particularly fertilizer type, fertilizer rate, time of year, soil moisture, soil pH, and rainfall. The majority of soil N exists as organic N in the form of organic matter or as N that has not been released from slow-release fertilizer granules. Technically, fertilizer granules are not a component of soil-stored N. However, the process of measuring soil N (combustion or digestion) will also measure N from any fertilizer granules that have not yet been released. The type and amount of slow-release fertilizer will directly influence this value. Once released from the slow-release form, N may remain in the soil via anion or cation exchange. The cation exchange capacity of most Florida soils is normally less than 3 milliequivalents of positive charge, and the anion exchange capacity is normally too low to measure. In Florida soils, mineralized N, N applied as urea, or N applied as NH4+ can rapidly convert to NO3- and, because NO3- is an anion, it is not retained by the soil. Thus, soil storage of N via cation exchange is commonly less than 10% of applied N (Shaddox 2001) and can be less than 2% (Brown 2003).
Nitrogen immobilization occurs when inorganic N is converted to organic N via microbial activity. An organic form of N is simply any form of N that is bound with carbon. Like plants, microbes require N to survive and some portion of applied N will be consumed by microbes and converted into amino acids, proteins, or some other organic form used for growth by the microbes. While in an organic form, N is not soluble and therefore is unavailable for plant uptake or loss to a water body. Organic N will remain unavailable for plant uptake until the environmental conditions change to favor N mineralization. The percentage of applied N that becomes microbially immobilized in Florida turfgrass systems will vary according to numerous factors including soil moisture, pH, and soil temperature. Little, if any, research has been conducted to determine immobilization of applied N in Florida turfgrass systems. Thus, providing an estimation is difficult. However, research conducted on turfgrass in cooler climates (Connecticut) reports that N immobilization may range from 15 to 26% of applied N (Starr and Deroo 1981). Because Florida receives more rainfall and is warmer than Connecticut, N immobilization in Florida may be lower than previous reports indicate because of increased microbial activity.
Ammonium fixation occurs when NH4+ enters the layer (lattice) of a 2:1 clay (Figure 4), becoming unavailable for plant uptake. Ammonium fixation in Florida soils is believed to contribute very little to the overall fate of applied N for two reasons. First, the content of 2:1 clays in Florida soils is normally very low and, second, NH4+ normally converts to NO3- very rapidly. The exact percentage of applied N to Florida turfgrasses that eventually is fixed by 2:1 clay minerals is unknown. However, evidence from other agronomic systems indicates the percentage is less than 5% (Nieder et al. 2011).
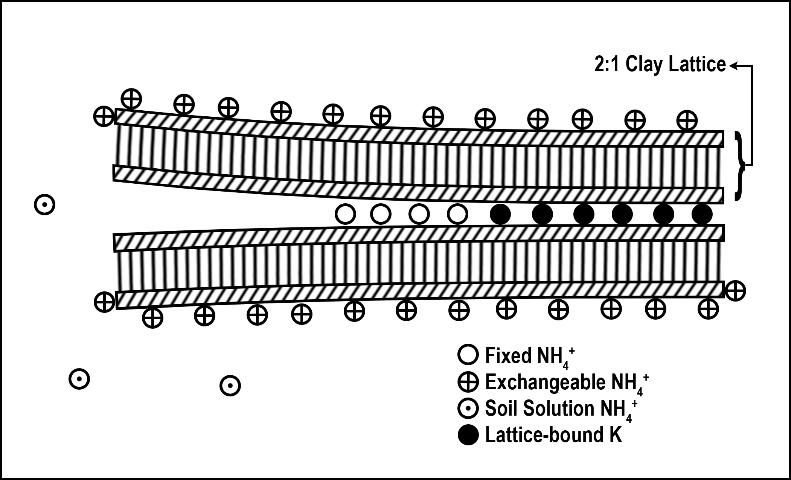
Credit: Modified from Strand (1998)
Leaching
Leaching is the process that moves soluble N below the rooting zone. Nitrogen leaching in turfgrass systems occurs at the moment soluble N moves below the deepest root. When turfgrass is fertilized according to UF/IFAS recommendations, N leaching is normally low and comparable to, or less than, other landscape plants (Erickson et al. 2008). As with other fates of applied N, the exact amount of N that will leach is difficult to determine. However, it is possible that 0 to 55% of applied N could be leached, with the higher percentages occurring when UF/IFAS recommendations are not followed. When N leaching does occur, it is usually a factor of the turfgrass species, irrigation management, N source, N rate, or stressed turfgrass.
Direct comparisons of N lost through leaching related to turfgrass species indicate that less N leaches through 'Raleigh' St. Augustinegrass than 'Empire' zoysiagrass, 'Meyer' zoysiagrass, 'Emerald' zoysiagrass, centipedegrass, 'Tifway' bermudagrass, and common bermudagrass (Bowman et al. 2002; Telenko et al. 2015; Trenholm et al. 2012). The influence of turfgrass species on N leaching losses is largely a factor of the turfgrass root system. Deeper-rooted turfgrasses tend to reduce N leaching losses compared to shallow-rooted turfgrasses (Bowman et al. 1998). Management practices that encourage deep rooting, such as deep, infrequent irrigation, are factors that shape UF/IFAS recommendations. Increased N leaching has been documented when N is applied within the first 60 days of planting sod (Telenko et al. 2015). After the sod has been planted for 60 days, N leaching is reduced and is a result of increased root growth. Based upon these results, UF/IFAS recommends N applications to newly sodded turf commence 60 days after the sod has been planted. This recommendation allows the sod to develop a root system prior to fertilization and thus minimizes the risk of N leaching.
The movement of water through the soil has a profound influence on N leaching. Once any nutrient becomes soluble in the soil solution, that nutrient is subject to the movement of water. Therefore, it is crucial to minimize any movement of water beyond the turfgrass rootzone. Increased water movement may be a result of excessive irrigation or fluctuations in rainfall due to changing seasons, which may result in more water being applied to the soil than the soil can retain. Moisture sensor or ET-based irrigation is more effective than daily irrigation at applying the amount of water the turfgrass needs without exceeding the rootzone's water holding capacity. Throughout the year, N leaching can be highest in February–March, reduced in April–May, and the lowest in June–July (Snyder et al. 1984). The reduction in N leaching from winter to summer is largely a factor of increased plant growth and increased ET, which reduce the amount of N in the soil solution and the amount of moisture in the rootzone, respectively. In each season, sensor-based irrigation can reduce N leaching by 2–28 times that of daily irrigation. Thus, UF/IFAS recommends refraining from applying any N when the National Weather Service has issued a flood, tropical storm, or hurricane watch or warning, or if heavy rains are likely. These recommendations reduce the risk of exceeding the soil's water retention capacity and, in turn, reduce N leaching.
When applied according to UF/IFAS recommendations, soluble N may not leach more N compared with N lost naturally from unfertilized turfgrass (Shaddox et al. 2016a). Additionally, slow-release N sources further reduce N leaching losses compared with soluble N sources. Essentially, slow-release N sources delay the release of N into the N cycle (Figure 1). Over time, small portions of N are released, which increases the likelihood of plant uptake of applied N and decreases potential for N leaching losses (Guillard and Kopp 2004). Blending soluble N sources with slow-release N sources also results in reduced N leaching losses (Shaddox 2001). Generally, differences in N leaching losses among slow-release N sources are negligible assuming they are applied at the same time and rate. However, organic N sources and polymer-coated N sources may result in the least amount of N leaching losses compared with other slow-release sources (Petrovic 2004). Enhanced efficiency fertilizers, such as nitrification and urease inhibitors, do not delay the release of N into the N cycle and thus result in similar N leaching losses as other soluble N sources (Guertal and Howe 2012).
Increasing the rate of applied N beyond the rate recommended by UF/IFAS (https://edis.ifas.ufl.edu/publication/ep353) can increase the risk of N leaching losses (Trenholm et al. 2012). UF/IFAS turfgrass nutrient recommendations take into account the turfgrass need for N and the potential impact on the environment. UF/IFAS nutrient recommendations are often 50–75% less than the amount of N necessary to increase N leaching losses above the natural environment (McGroary et al. 2017; Trenholm et al. 2012). Thus, current rates are considered conservative, and exceeding these rates is unnecessary because any further increase in turfgrass growth or quality is minimal and could come at a cost to the environment.
As previously mentioned, N applied according to UF/IFAS recommendations to healthy, growing turfgrass has a low probability of leaching. However, when turfgrass is stressed, N leaching can increase (Telenko et al. 2015). Normally, stresses manifest themselves as reductions in turfgrass density and growth, which correspond to a reduction in N uptake. These stresses are largely environmental caused by pests, late-season frosts, and changes in season. However, stresses can also be anthropogenic caused by misapplications of nutrients or pest control products. When stresses occur, further applications of N may not cure the problem and may, in fact, exacerbate the problem and increase N leaching. Further research regarding how to manage nutrient applications to stressed turf is needed.
Runoff
Runoff is defined as the lateral movement of N beyond the target location. Runoff may occur above or below the soil surface but always occurs above the deepest root. At the moment that N moves below the deepest root, further movement of N is defined as leaching. Leached N may then runoff if the leached N encounters a subsurface barrier, but the N lost from the turfgrass system is considered leached if the N moved vertically beyond the rootzone. Nitrogen lost via runoff may be influenced by topography, soil type, soil compaction, soil moisture, rainfall, and fertilizer type. Because Florida soils are predominantly sand-based and have a high water infiltration capacity, the movement of water across the soil surface is far less common than the movement of water into the soil. Thus, in Florida, runoff studies are less common than leaching studies because the few runoff studies that do exist report that little to no runoff occurs. In Florida, when N is applied on steep slopes subject to intense irrigation rates, N found in runoff has been reported to be less than 0.1% of that applied (Shaddox and Sartain 2001). This evidence does not discount the probability that runoff could occur under different conditions. However, even on less permeable soils than many soils found in Florida, N runoff from turfgrass is commonly 0% of applied N, but may approach 7% on topographies and environments that are uncommon in Florida (Brown et al. 1977; Morton et al. 1988).
Summary
The fate of N applied to Florida turfgrass may vary greatly depending upon numerous factors. Essentially all N used in turfgrass management originated from the atmosphere and will eventually return to the atmosphere. During this cycle, ranges of the potential fates of applied N to Florida turfgrasses are:
-
Volatilization—<1%–60%
-
Denitrification—<1%–5%
-
Plant uptake —40%–68%
-
Soil Storage—7%–15%
-
Leaching—<1%–55%
-
Runoff—<1%–7%
References
Ashley, D.A., O.L. Bennett, B.D. Doss, and C.E. Scarsbrook. 1965. "Effect of nitrogen rate and irrigation on yield and residual nitrogen recovery by warm-season grasses." Agron. J. 57:370–372.
Association of American Plant Food Control Officials. 2017. Official Publication. Association of American Plant Food Control Officials, Inc., West Lafayette, IN.
Bowman, D.C., C.T. Cherney, and T.W. Rufty. 2002. "Fate and transport of nitrogen applied to six warm-season turfgrasses." Crop Sci. 42:833–841.
Bowman, D.C., D.A. Devitt, M.C. Engelke, and T.W. Rufty. 1998. "Root architecture affects nitrate leaching from bentgrass turf." Crop Sci. 38:1633–1639.
Brown, E.A. 2003. "Differential nitrate leaching and mass balance of 15N-labeled nitrogen sources applied to turfgrass and citrus." Ph.D. diss., University of Florida, Gainesville, FL.
Brown, K.W., R.L. Duble, and J.C. Thomas. 1977. "Influence of management and season on fate of N applied to golf greens." Agron. J. 69:667–671.
Carrow, R.N., D.V. Waddington, and P.E. Rieke. 2001. Turfgrass soil fertility and chemical problems: assessment and management. Chelsea, Michigan: Ann Arbor Press.
Dobbs, N.A., K.W. Migliaccio, Y.C. Li, M.D. Dukes, and K.T. Morgan. 2014. "Evaluating irrigation applied and nitrogen leached using different smart irrigation technologies on bahiagrass (Paspalum notatum)." Irrig. Sci. 32:193–203.
Erickson, J.E., J.L. Cisar, G.H. Snyder, D.M. Park, and K.E. Williams. 2008. "Does a mixed-species landscape reduce inorganic-nitrogen leaching compared to a conventional St. Augustinegrass lawn?" Crop Sci. 48:1586–1594.
Erisman, J.W., M.A. Sutton, J. Galloway, Z. Klimont and W. Winiwarter. 2008. "How a century of ammonia synthesis changed the world." Nat. Geosci. 1:636-639. doi:10.1038/ngeo325.
Florida Department of Agriculture and Consumer Services. 2017. "Archive fertilizer tonnage data." Accessed March 13, 2017. http://www.freshfromflorida.com/Divisions-Offices/Agricultural-Environmental-Services/Business-Services/Fertilizer/Fertilizer-Manufacturers/Fertilizer-Tonnage-Data/Archive-Fertilizer-Tonnage-Data
Franzen, D., R.J. Goos, R.J. Norman, T.W. Walker, T.L. Roberts, N.A. Slaton, G. Endres, R. Ashley, J. Staricka, and J. Lukach. 2011. "Field and laboratory studies comparing nutrisphere-nitrogen urea with urea in North Dakota, Arkansas, and Mississippi." J. Plant Nutr. 34:1198–1222. doi:10.1080/01904167.2011.558162.
Galloway, J.N., F.J. Dentener, D.G. Capone, E.W. Boyer, R.W. Howarth, S.P. Seitzinger, G.P. Asner, C.C. Cleveland, P.A. Green, E.A. Holland, D.M. Karl, A.F. Michaels, J.H. Porter, A.R. Townsend, and C.J. Vorosmarty. 2004. "Nitrogen cycles: past, present, and future." Biogeochemistry 70:153–226. doi:DOI 10.1007/s10533-004-0370-0.
Galloway, J.N., A.R. Townsend, J.W. Erisman, M. Bekunda, Z.C. Cai, J.R. Freney, L.A. Martinelli, S.P. Seitzinger, and M.A. Sutton. 2008. "Transformation of the nitrogen cycle: Recent trends, questions, and potential solutions." Science 320: 889–892. doi:10.1126/science.1136674.
Goos, R.J. 2011. Nitrogen fertilizer additives, which ones work? North central extension-industry soil fertility conference, Des Moines, IA. Nov. 16–17.
Guertal, E.A., and J.A. Howe. 2012. "Nitrate, ammonium, and urea leaching in hybrid bermudagrass as affected by nitrogen source." Agron. J. 104:344–352.
Guillard, K., and K.L. Kopp. 2004. "Nitrogen fertilizer form and associated nitrate leaching from cool-season lawn turf." J. Environ. Qual. 33:1822–1827.
Havlin, J.L., J.D. Beaton, S.L. Tisdale, and W.L. Nelson. 1999. Soil fertility and fertilizers: an introduction to nutrient management. 6th ed. Upper Saddle river, NJ: Prentice Hall.
Janzen, H.H., and J.R. Bettany. 1986. "Influence of thiosulfate on nitrification of ammonium in soil." Soil Sci. Soc. Am. J. 50:803–806.
Katz, B.G., D.W. Griffin, P.B. McMahon, H.S. Harden, E. Wade, R.W. Hicks, and J.P. Chanton. 2010. "Fate of effluent-borne contaminants beneath septic tank drainfields overlying a Karst aquifer." J. Environ. Qual. 39:1181–1195. doi:10.2134/jeq2009.0244.
Malzer, G.L. 1989. "Incorporation of nitrification inhibitors with urea and urea-ammonium nitrate for irrigated corn." Fert. Res. 18:141–151.
Malzer, G.L., K.A. Kelling, M.A. Schmitt, R.G. Hoeft, and G.W. Randall. 1989. "Performance of dicyandiamide in the north central states." Commun. Soil. Sci. Plant Anal. 20:2001–2022. doi:Doi 10.1080/00103628909368198.
Mancino, C.F., W.A. Torello, and D.J. Wehner. 1988. "Denitrification losses from Kentucky bluegrass sod." Agron. J. 80:148–153.
McGroary, P., T.W. Shaddox, J.L. Cisar, J.B. Unruh, and L.E. Trenholm. 2017. "Annual nitrogen requirements of bahiagrass lawns maintained in subtropical climates." Int. Turf. Soc. Res. J. 13:1–9. doi:10.2134/itsrj2016.05.0420.
Morton, T.G., A.J. Gold, and W.M. Sullivan. 1988. "Influence of overwatering and fertilization on nitrogen losses from home lawns." J. Environ. Qual. 17:124–130.
National Atmospheric Deposition Program. 2015. https://nadp.slh.wisc.edu. Accessed 10 Feb. 2023.
Nieder, R., D.K. Benbi, and H.W. Scherer. 2011. "Fixation and defixation of ammonium in soils: a review." Biol. Fert. Soils 47: 1–14. doi:10.1007/s00374-010-0506-4.
Petrovic, A.M. 2004. "Nitrogen source and timing impact on nitrate leaching from turf." Acta Hortic. 661: 427–432.
Pye, V.I., R. Partrick, and J. Quales. 1983. Groundwater contamination in the United States. Philadelphia, PA: Univ. of Pennsylvania Press.
Sartain, J.B. 1985. "Effect of acidity and N-Source on the growth and thatch accumulation of tifgreen bermudagrass and on soil nutrient retention." Agron. J. 77:33–36.
Sartain, J.B. 2008. "Comparative influence of N source on N leaching and St. Augustinegrass quality, growth and N uptake." Soil Crop Sci. Soc. Fl. 67:43–47.
Schmidt, C.A., and M.W. Clark. 2012. "Efficacy of a denitrification wall to treat continuously high nitrate loads." Ecol. Eng. 42: 203–211. doi:10.1016/j.ecoleng.2012.02.006.
Shaddox, T.W. 2001. "Fate of nitrogen during grow-in of a golf course fairway under different nitrogen management practices and irrigation intensities." Master's thesis, University of Florida, Gainesville, FL.
Shaddox, T.W. and J.B. Sartain. 2001. "Fate of nitrogen during grow-in of a golf course fairway under different nitrogen management practices." Soil Crop Sci. Soc. Fl. 60:59–63.
Shaddox, T.W., J.B. Unruh, and L.E. Trenholm. 2016a. "Nitrate leaching from soluble nitrogen applied to 'Floratam' St. Augustinegrass and common centipedegrass during dormancy." Crop Sci. 56:837–844. doi:10.2135/cropsci2015.02.0104.
Shaddox, T.W., J.B. Unruh, L.E. Trenholm, P.C. McGroary, and J.L. Cisar. 2016b. "Nitrogen rate required for acceptable St. Augustinegrass and associated nitrate leaching." Crop Sci. 56:439–451. doi:10.2135/cropsci2015.04.0226.
Smil, V. 2001. Enriching the earth : Fritz Haber, Carl Bosch, and the transformation of world food production. Cambridge, Massachusetts: MIT Press.
Snyder, G.H., B.J. Augustin, and J.M. Davidson. 1984. "Moisture sensor-controlled irrigation for reducing N leaching in bermudagrass turf." Agron. J. 76:964–969.
Starr, J.L., and H.C. Deroo. 1981. "The fate of nitrogen-fertilizer applied to turfgrass." Crop Sci. 21:531–536.
Stiegler, J.C., M.D. Richardson, and D.E. Karcher. 2011. "Foliar nitrogen uptake following ureaa application to putting green turfgrass species." Crop Sci. 51:1253–1260. doi:10.2135/cropsci2010.06.0377.
Strand, L. 1998. "Remineralisation and defixation of soil nitrogen." Accessed 21 Nov 2017. http://www.vaxteko.nu/html/sll/slu/ex_arb_vaxtnaringslara/EVN108/EVN108.HTM
Sullivan, W.M., Z.C. Jiang, and R.J. Hull. 2000. "Root morphology and its relationship with nitrate uptake in Kentucky bluegrass." Crop Sci. 40: 765–772.
Telenko, D.E.P., T.W. Shaddox, J.B. Unruh, and L.E. Trenholm. 2015. "Nitrate leaching, turf quality, and growth rate of 'Floratam' St. Augustinegrass and common centipedegrass." Crop Sci. 55: 1320–1328.
Trenholm, L.E., and J.B. Unruh. 2005. "Warm-season turfgrass response to fertilizer rates and sources." J. Plant Nutr. 28: 991–999.
Trenholm, L.E., J.B. Unruh, and J.B. Sartain. 2012. "Nitrate leaching and turf quality in established 'Floratam' St. Augustinegrass and 'Empire' zoysiagrass." J. Environ. Qual. 41:793–799.
Warneke, S., L.A. Schipper, M.G. Matiasek, K.M. Scow, S. Cameron, D.A. Bruesewitz, and I.R. McDonald. 2011. "Nitrate removal, communities of denitrifiers and adverse effects in different carbon substrates for use in denitrification beds." Water Res. 45:5463–5475. doi:10.1016/j.watres.2011.08.007.
Wherley, B.G., W. Shi, D.C. Bowman, and T.W. Rufty. 2009. "Fate of (15)N-nitrate applied to a bermudagrass system: assimilation profiles in different seasons." Crop Sci. 49:2291–2301.
Young, N.G. 2015. "Drought avoidance acclimation of warm season turfgrass in response to mild-reoccuring soil water deficit and N fertilization." Ph.D. Diss., University of Florida, Gainesville, FL.