Background
Many of Florida's nutrient-enriched lakes and reservoirs experience a proliferation of phytoplankton, or microscopic algae, in their water during summer months. Most often these bloom events are caused by a certain kind of phytoplankton—cyanobacteria, also referred to as blue-green algae. Cyanobacteria are the most common kind of phytoplankton in Florida lakes and reservoirs, and their occurrence is natural. However, when they grow to high levels and bloom, they can cause problems with aesthetics, emit an odor if they wash up on shore and die, and occasionally be toxic to aquatic organisms. Before undertaking costly remediation projects, or evaluating the effectiveness of nutrient reduction strategies, it is important for resource managers to understand how natural variation in weather and climate affect cyanobacteria blooms.
Cyanobacteria blooms generally are linked to high levels of nutrients in the lake water, and in Florida those nutrient levels vary tremendously. This variance is in part because the level of nutrients in Florida rocks and soils is highly variable, resulting in variation of nutrient levels in the overlying lake water (Bachmann et al. 2012). Humans can also cause higher nutrient inputs. One example is Lake Tohopekaliga, located south of Orlando. It once received high levels of nutrients from sewage treatment plants, which caused intense algae blooms (James et al 1994). The lake recovered after sewage diversion.
The concentration of phytoplankton in the water of a Florida lake or reservoir also can vary considerably over time and even within different areas of the same water body. The changes in concentration can be due to either local differences in environmental conditions or to buoyant algae being concentrated along a shoreline when blown there by the wind. Rainfall and drought can cause large-scale changes in the concentration of phytoplankton and the occurrence of algae blooms in Florida lakes. Rainfall over the Florida peninsula is linked to distant ocean cycles, including the multi-year cycle in the Pacific known as the ENSO or El Niño Southern Oscillation (Abtew and Trimble 2010). In El Niño years, there is considerably more rainfall from late fall to early spring, whereas during La Niña years, rainfall is considerably reduced and winter droughts can occur. Hoyer et al. (2005) examined data from 84 lakes in Florida to determine how variability in rainfall affects the concentration of chlorophyll in the water. Chlorophyll (Chl) is a major pigment in algae and can serve as a proxy for the amount of phytoplankton in the water column. Hoyer et al. (2005) found that in some lakes there was a higher concentration of Chl in years of high rainfall, in some lakes it was higher in years of low rainfall, and in a third group of lakes there was no rainfall effect. They concluded that it is important to understand this relationship for lake management purposes, and that a careful lake-by-lake study might be needed in order to identify that relationship, its underlying causes, and its effects.
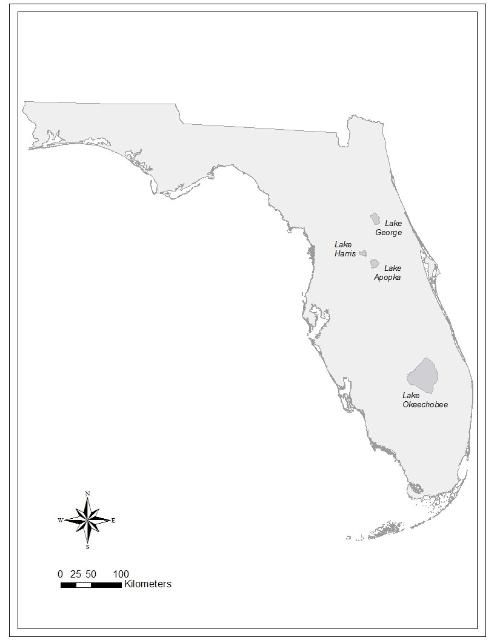
Credit: Corina Guevara, Florida Sea Grant
Here, we present results from three large, nutrient-rich lakes in Florida that have summer blooms of cyanobacteria: Lake Harris in the Ocklawaha Chain of Lakes north of Orlando; Lake George in the headwaters of the St. Johns River south of Jacksonville; and Lake Okeechobee in south Florida (Figure 1). All three lakes have long-term data-collection programs that include monitoring water quality and cyanobacteria, complemented by extensive research programs. The extensive data allowed us to (a) determine the relationship between rainfall and cyanobacteria blooms; and (b) develop hypotheses about what causes year-to-year bloom variability.
Research Methods
Havens et al. (2016a) assembled a 15-year data set on Lake Harris including monthly measurements of rainfall, water depth, water color, flushing rate (the rate at which water in the lake is being replaced by inflow water), and the biomass of cyanobacteria, calculated from counts and measurements of algal cells. Biomass, expressed in units of micrograms per liter, is a standard measure of the mass of algae in a unit of lake water.
A 15-year dataset from Lake George was assembled by Havens et al. (2016b), and included monthly measurements of water level, discharge rates at the lake's outlet (a measure of the rate at which water is flowing through the system), water color, and cyanobacteria biomass, determined in the same manner as in Lake Harris.
Lake Okeechobee data were obtained from several research projects done between 1993 and 2016 that included measurements of biomass of cyanobacteria using the same methods as in the other two lakes. The data also included studies in the lake's deeper central region overlying mud sediment and a shallow region overlying sand and peat sediment where, unlike the other two lakes, a vast area of submerged plants can grow (Havens et al. 2002).
Findings
Lake Harris
The yearly averaged water levels in Lake Harris are highly correlated with the amount of rain that falls over the watershed in the preceding year. This reflects the fact that water must first flow off a large land area, then into lakes upstream in the chain, before finally entering Lake Harris. As is the case elsewhere in the Florida peninsula, the amount of rainfall, particularly between November and May, is strongly correlated with the condition of the El Niño Southern Oscillation (Abtew and Trimble 2010).
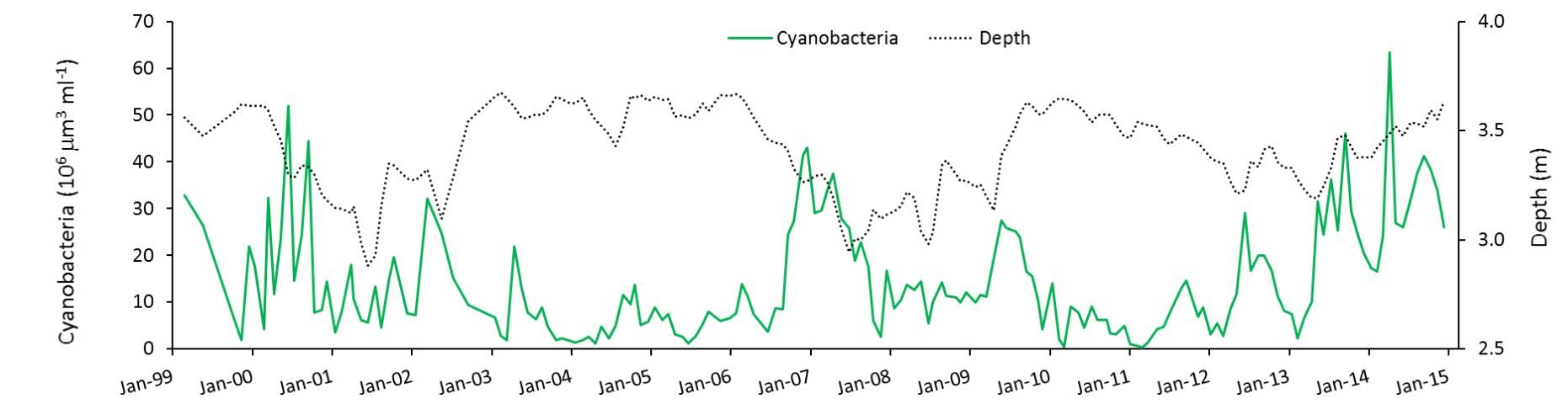
During the 15 years in which Lake Harris was studied, there were two periods of high water and two periods of low water. Cyanobacteria blooms waxed and waned with season, and there was a pattern of summer blooms being less intense in high-water years (Figure 2).
How is this explained? A statistical analysis of the results indicates that summer blooms either are suppressed by something that happens when water depth is higher, or they are stimulated by something that happens when water depth is lower—or both. Havens et al. (2016a) found no evidence of a connection between nutrient inputs and blooms.
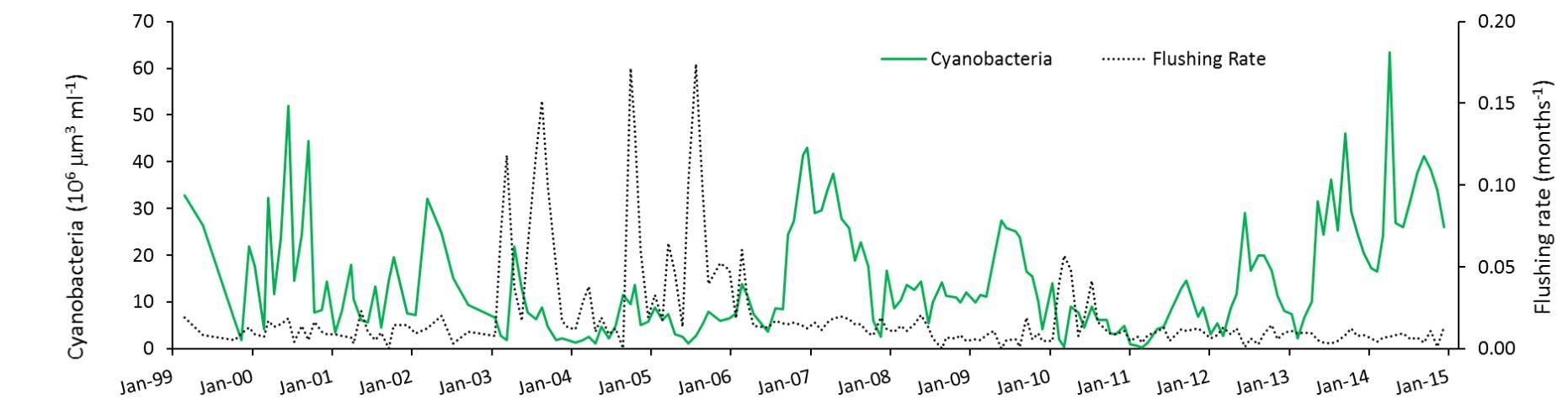
However, they observed that two factors associated with water level and inflow might be at play when the lake is deep compared to when it is shallower. The first factor is flushing rate. During times when the lake had little water flowing through it and was nearly stagnant (flushing rate near zero in Figure 3), blooms in summer were able to develop to a high level, presumably because of uninterrupted growth. In contrast, in deep-water years, when a large volume of the lake water was periodically flushed out, blooms were greatly reduced, perhaps indicating that the balance between growth and loss of algae becomes negative during those events.
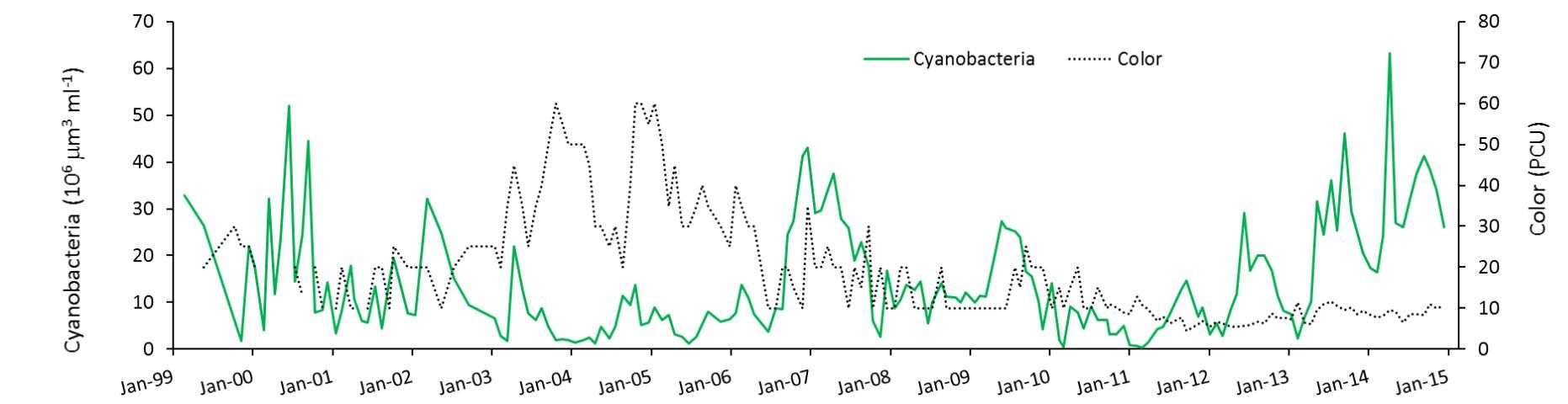
Havens et al. (2016a) found that during the high-water periods, the color of the water was elevated (Figure 4). Color is a measure of dissolved organic material in the water. The organic material that changes water color is sometimes formed within the lake, and sometimes comes into the lake from decomposing plants or leaches from soils in the watershed. In this case, knowing that source water to the lake comes from a highly colored river, it was suggested that the increased inflow contributed to elevated color. Colored dissolved organic matter absorbs a considerable amount of light, in the same part of the spectrum that algae require for growth. Thus, higher color also could have reduced blooms in the high-water periods. We conclude that two co-occurring factors (flushing and color) may have been at play in reducing blooms, especially when the lake's water column was deeper.
Lake Harris, like nearly all other Florida lakes is shallow, with a maximal depth less than 5 m. It has mud sediments at its bottom that contain high levels of nutrients that have accumulated over time, and it has a dense population of a particular kind of fish, gizzard shad, that feed on the lake bottom. Shad are known from other studies to mobilize large amounts of nutrients from the sediments into the water. These factors suggest another hypothesis, one which needs to be tested in future research, namely that during low-water periods, the normal array of chemical and biological processes (including fish) are at work releasing nutrients from the sediments into the water. With a lesser amount of water, the concentration of nutrients is higher, which provides more nutrient supplies for algae, and the potential for larger blooms.
We can summarize these effects using a simple conceptual diagram (Figure 5) of hypothetical controlling factors. To resolve the relative importance of the various processes requires a focused research project. At this point we only can say that normal variations in water depth that are linked with a climate cycle can have a major impact on the intensity of potentially toxic cyanobacteria blooms, and there are several explanations that suggest it is more than a coincidental occurrence.
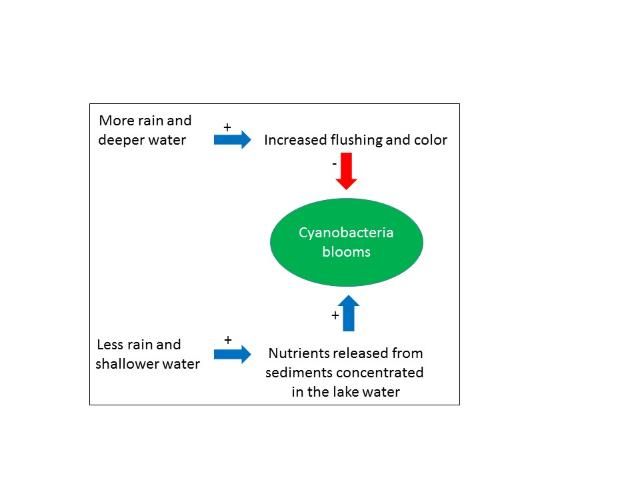
Lake George
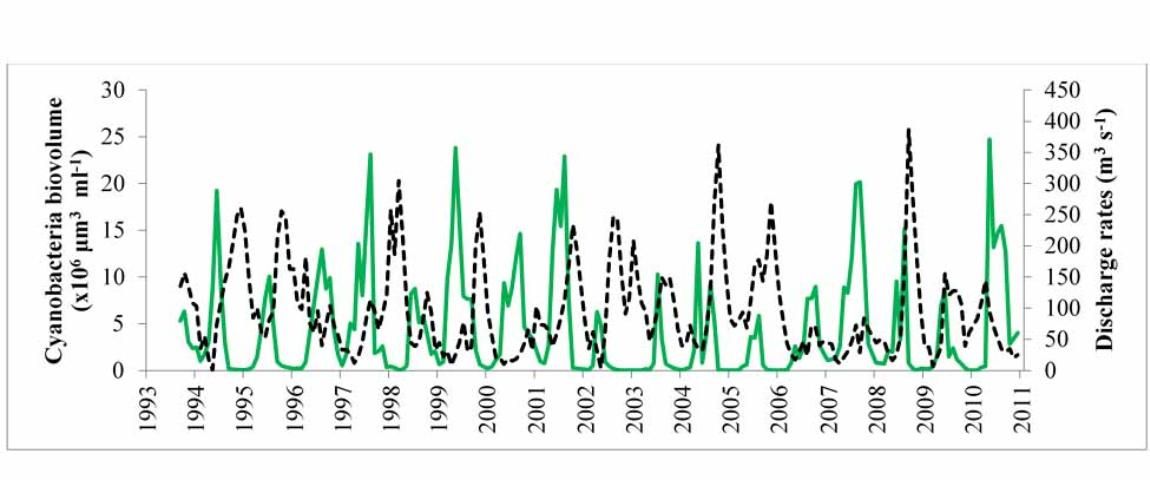
In this lake, there also is a 15-year, monthly record of the biomass of phytoplankton (Figure 6), and it can be observed that the maximal summer biomass is lower in some years than in others. A statistical analysis of these data (Havens et al. 2016b) revealed a similar result to what was found in Lake Harris. In years of below-average rainfall and lower water levels, the most intense summer blooms of cyanobacteria occurred. Blooms were reduced in years of high rainfall and higher water, which coincided with more water flowing through the lake into the St. Johns River and with much higher color. Recall that colored water absorbs light and reduces phytoplankton growth potential (Srifa et al. 2016). It also may be the case that the stimulatory processes identified in Figure 4 were at play in lower-water years and favored blooms of cyanobacteria.
Lake Okeechobee
In the open-water region of Lake Okeechobee, blooms of cyanobacteria generally are suppressed by a high amount of inorganic solids in the water created by wind and waves suspending the muddy bottom sediments. The suspended solids reduce light penetration (Phlips et al. 1993; Aldridge et al. 1995; Phlips et al. 1995). However, there are occasional intense summer cyanobacteria blooms. Havens et al. (1998) examined physical, chemical and biological data from the open-water region and by using multi-variate statistics, found that blooms are not necessarily stimulated by elevated levels of nutrients, but rather, by periods of time when there is a combination of unusually low rainfall, hot and calm conditions, and a water column that is stable for many days (Figure 7). These conditions allow cyanobacteria to grow, float to the water surface, and form intense blooms. The process starts with dormant cyanobacteria near the lake sediments. The most recent lake-wide bloom was in summer 2005. In that case, there was a perfect storm where large rain and wind events introduced high levels of nutrients into the water and then a hot, calm period provided favorable conditions for cyanobacteria blooms to occur. This response is not unlike that observed in Lakes Harris and George—i.e., cyanobacteria have a preference for periods of tranquil water and hot mid-summer conditions. Intense rainfall and mixing of the water by wind during hurricanes ends Okeechobee's cyanobacteria blooms. Hurricane Irene in autumn 2005, for instance, immediately disrupted the cyanobacteria bloom in Okeechobee.
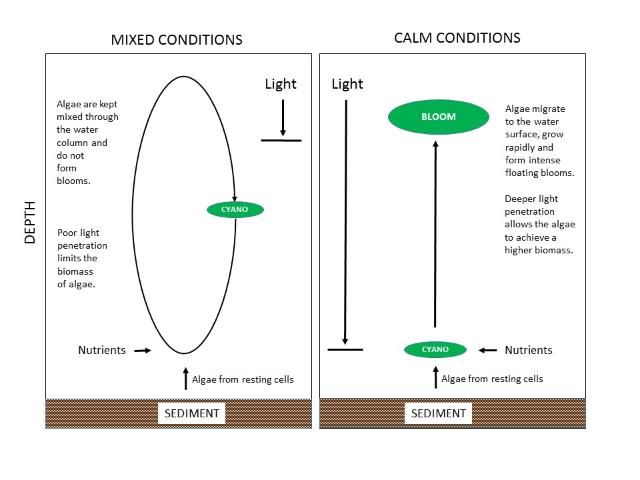
Lake Okeechobee is unique among the three lakes in also having a vast area along the west and south shore that can support submerged plants—up to 50,000 acres (Havens et al. 2002). In this particular lake region, water level has the opposite effect from what was described for Lake Harris, Lake George, and the open-water region of Lake Okeechobee. The response is akin to what is observed in shallow lakes in the Netherlands (Scheffer et al. 1993). When the lake is deep, insufficient light gets to the bottom to support growth of plants. Phytoplankton is the dominant form of primary producers and blooms can occur. When the lake is shallow and depth is less than one meter in the shoreline area, light reaches the bottom and there is dense growth of submerged plants that compete with the phytoplankton for nutrients (Hwang et al. 1998), keeping blooms in check. There no doubt are other lakes in Florida that behave in this manner, but to our knowledge, none with such a comprehensive history of monitoring and research.
Practical Implications for Lake Management
The results presented here illustrate how variation in rainfall, which results in wet and dry periods with high and low lake levels, respectively, can have profound effects on blooms of cyanobacteria in Florida lakes and reservoirs. Large-scale changes in the maximal summer level of cyanobacteria were observed in three large Florida lakes—Harris, George, and also the open-water region of Okeechobee. In all three cases, years of increased bloom intensity can be explained by physical and chemical factors related to variation in weather and climate. In the nearshore region of Lake Okeechobee, which can support submerged plants, there is a different relationship, and blooms are suppressed in low-water years.
In order to protect lakes and reservoirs from over-enrichment by nutrients (eutrophication), the Environmental Protection Agency requires the states establish nutrient concentration and input thresholds. In Florida, this must be done in the context of natural background variation caused by differences in bedrock and soils. The results presented here demonstrate that nutrient standards also need to consider how lakes and reservoirs respond to natural variation in weather, including variation that is related to climate cycles such as the El Niño / La Niña. In the future, effects of climate change on our weather may also need to be considered. At the present time, climate models consistently project that the future will be warmer, with a greater loss of water from lakes and reservoirs by evapotranspiration. However, the climate models do not agree about future trends in rainfall (Obeysekera et al. 2015). As new research emerges, managers will need to consider whether or not climate change is a substantive issue for lake rehabilitation planning.
There is another reason why, from a lake-management standpoint, it is critical to understand natural variation in cyanobacteria blooms: because without being aware of natural environmental controls, we could reach wrong conclusions and take wrong actions. For example, if an agency is monitoring a lake or reservoir where nutrient inputs have been reduced, and this coincides with the onset of a multi-year drought, there might be intensified cyanobacteria blooms simply due to the lower water. If the agency doesn't understand the relationship between lower water levels and cyanobacteria blooms, it might conclude that nutrient-reduction strategies were not sufficiently aggressive, and additional, unnecessary projects might be planned, possibly wasting tax dollars. Alternatively, an agency might implement a nutrient-reduction program at a time when a lake or reservoir transitions from a drought to a wet period and observe a large suppression of cyanobacteria as happened in Lake Harris and George. The agency might wrongfully attribute the suppression to its nutrient-reduction program and decide to terminate rehabilitation efforts, when in fact they might still be needed.
The bottom line is that periods of extremes in rainfall (wet and dry) can significantly impact cyanobacteria blooms along with the nutrients discharged during these events. Agencies charged with setting water quality standards and monitoring and enforcing compliance with those standards must consider these naturally occurring events. If either water-quality standards or compliance are determined and evaluated in only periods of extremes, invalid conclusions will almost necessarily be made. This is why several authors have suggested the need for multiple years of data ranging from 6 to 20 years to evaluate true trends in lake water chemistry (Knowlton and Jones 2006) and responses to nutrient-reduction strategies.
Literature Cited
Abtew, W., and P. Trimble. 2010. "El Niño-Southern Oscillation link to south Florida hydrology and water management applications." Water Resources Management 24: 4255–4271.
Aldridge, F. J., E. J. Phlips, and C. L. Schelske. 1995. "The use of nutrient enrichment bioassays to test for spatial and temporal distribution of limiting factors affecting phytoplankton dynamics of Lake Okeechobee, Florida." Archiv für Hydrobiologie, Advances in Limnology 45: 177–190.
Bachmann, R. W., D. L. Bigham, M. V. Hoyer, and D. E. Canfield, Jr. 2012. "Factors determining the distributions of total phosphorus, total nitrogen and chlorophyll a in Florida lakes." Lake and Reservoir Management 28: 10–26.
Havens, K. E., R. S. Fulton III, J. R. Beaver, E. E. Samples, and J. Collee. 2016a. "Effects of climate variability on cladoceran zooplankton and cyanobacteria in a shallow subtropical lake." Journal of Plankton Research, DOI 10.1093/plankt/fwb009.
Havens, K. E., M. C. Harwell, M. A. Brady, B. Sharfstein, T. L. East, A. J. Rodusky, D. Anson, and R. P. Maki. 2002. "Large-scale mapping and predictive modeling of submerged aquatic vegetation in a shallow eutrophic lake." The Scientific World Journal 2: 949–965.
Havens, K. E., H. Paerl, E. J. Phlips, M. Zhu, J. R. Beaver, and A. Srifa. 2016b. "Extreme weather events and climate variability provide a lens into how shallow lakes may respond to climate change." Water 8:229 (DOI:10.3390/w8060229).
Havens, K. E., E. J. Phlips, M. F. Cichra, and B. L. Li. 1998. "Light availability as a possible regulator of cyanobacteria species composition in a shallow subtropical lake." Hydrobiologia 39: 547–556.
Hoyer, M. V., C. A. Horsbaugh, D. E. Canfield, and R. W. Bachmann. 2005. "Lake level and trophic state variables among a population of shallow Florida lakes and within individual lakes." Canadian Journal of Fisheries and Aquatic Sciences 62: 2760–2769.
Hwang, S. J., K. E. Havens, and A. D. Steinman. 1998. "Phosphorus kinetics of planktonic and benthic assemblages in a shallow subtropical lake." Freshwater Biology 40: 729–745.
James, R. T., K. O'Dell, and V. H. Smith. 1994. "Water quality trends in Lake Tohopekaliga, Florida, USA: responses to watershed management." Journal of the American Water Resources Association 30: 531–546.
Knowlton, M. F., and J. R. Jones. 2006. "Temporal variation and assessment of trophic state indicators in Missouri reservoirs: implication for lake monitoring and management." Lake and Reservoir Management 23: 261–271.
Obeysekera, J., J. Barnes, and M. Nungesser. 2015. "Climate sensitivity runs and regional hydrologic modeling for predicting the response of the greater Florida Everglades ecosystem to climate changes." Environmental Management 55: 749–762. (DOI 10.1007/s00267-014-0315-x.)
Phlips, E. J., F. J. Aldridge, P. Hansen, P. V. Zimba, J. Ihnat, M. Conroy, and P. Ritter. 1993. "Spatial and temporal variability of trophic state parameters in a shallow subtropical lake (Lake Okeechobee, Florida, USA)." Archives Hydrobiologia 128:437–458.
Phlips, E. J., F. J. Aldridge, C. L. Schelske, and T. L. Crisman. 1995. "Relationship between light availability, chlorophyll a and tripton in a large shallow sub-tropical lake." Limnology and Oceanography 40:416–421.
Scheffer, M., S. H. Hosper, M. L. Meijer, B. Moss, and E. Jeppesen. 1993. "Alternative equilibria in shallow lakes." Trends Ecol. Evol. 8: 275–279.
Srifa, A., E. J. Phlips, M. F. Cichra, and J. C. Hendrickson. 2016. "Phytoplankton dynamics in a sub-tropical lake dominated by cyanobacteria: Cyanobacteria 'like it hot and sometimes dry." Aquatic Ecology 50:163–174 (http://dx.DOI.org/10.1007/s10452-016-9565-4).