Water Hammer In Irrigation Systems
Irrigation system design includes pump sizing and selection, valve sizing and selection, and pipe sizing as well as the proper selection and placement of many other components. Good design involves selecting and sizing components to perform their intended tasks within the constraints of the system, to ensure an efficient, cost effective, and durable irrigation system. Cost effectiveness will result when oversizing is avoided. Undersizing of components will cut initial costs but often results in poor irrigation distribution uniformity or the complete or partial failure of the system through ruptured pipes, damaged valves, or pump damage.
Like any other moving fluid, flowing water has momentum. When subjected to a sudden change in flow, shock waves propagate through the system. This occurrence is referred to as "water hammer." Flow changes can occur due to operation of valves, starting and stopping of pumps, or directional changes caused by pipe fittings. The intensity of water hammer effects will depend upon the rate of change in the velocity or momentum. This publication discusses the causes of water hammer and the importance of proper system design and management to ensure a cost effective, long-lasting irrigation system.
Causes of Water Hammer
Water is slightly compressible. Because of this characteristic, shock waves can occur and propagate through confined water systems. Shock waves in pipe systems can result from sudden changes in flow such as:
- rapid opening of closing of control valves;
- starting and stopping of pumps;
- the recombination of water after water column separation; or
- the rapid exhaustion of all air from the system
When sudden changes in flow occur, the energy associated with the flowing water is suddenly transformed into pressure at that location. This excess pressure is known as surge pressure and is greater with large changes in velocity. Characteristics of the pipe such as the materials used in construction, the wall thickness, and the temperature of the pipe all affect the elastic properties of the pipe and how it will respond to surge pressures.
Pipe Characteristics
The ratio of the diameter of the pipe to the wall thickness (t) is referred to as the dimension ratio (DR). All polyvinyl chloride (PVC) and some polyethylene (PE) pipe use the outside diameter (OD) of the pipe in this ratio. Some pipe sizing is based on inside diameter (ID) and uses that diameter in the DR computation. Certain DR values have been designated to be standards and are referred to as Standard Dimension Ratios (SDR).
The DR relationship can be determined when pipe thickness (t) and either outside diameter (OD) or inside diameter (ID) are known. For outside diameter based pipe (PVC and most PE pipe), the DR is calculated as
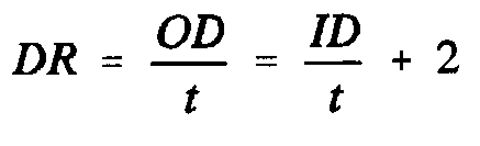
For inside diameter based pipe, the DR is calculated as Sometimes pipe is referred to as Class X pipe where X represents the pressure rating of the pipe in pounds per square inch (psi). For example, Class 160 PVC pipe is pressure rated for 160 psi. Table 1 shows the pressure ratings associated with certain SDR values for nonthreaded PVC (PVC 1120, PVC 1220, and PVC 2120) and PE 3408 pipe at a water temperature of 73.4°F.
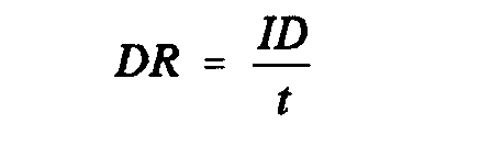
Temperature Effects On Plastic Pipe Properties
Water and environmental temperatures will affect the properties of the pipe material. As temperatures increase the pipe material will become more ductile (elastic). Therefore the pressure ratings shown in Table 1 must be derated (decreased) with a service factor for higher temperature conditions to provide for safe operation. Table 2 shows the service factors for PVC and PE pipes for temperatures higher than 73.4°F. The pressure rating of the pipe from Table 1 should be multiplied by the appropriate service factor from Table 2 to obtain the temperature compensated pressure rating of the pipe. For example SDR 26.0 PVC pipe has a 160 psi pressure rating at 73.4°F. When the water temperature is increased to 80°F (also increasing the pipe temperature), the pressure rating decreases to [(0.88)(160 psi) = ] 128 psi.
Most groundwater sources in Florida will have temperatures of 73.4°F or lower. However, surface water sources (ponds, lakes, canals, etc.) can have higher temperatures. Also, water traveling through long laterals will be heated by solar radiation. Therefore, pipe temperatures should be estimated and designs should consider the higher temperatures that will be encountered.
Surge Pressures
Surge pressures associated with changes in flow or velocity can be calculated. The change in velocity must be known as well as properties of the conduit or piping system. The equation for calculating surge pressure is discussed in detail in the Appendix and can be applied to many types of pipes.
Because PVC pipe is perhaps the most common construction material used in irrigation systems, it will be discussed in more detail in this bulletin. Table 3 shows the maximum or critical surge pressures associated with a sudden change in velocity for 400,000 psi modulus of elasticity (a measure of the stiffness of the material) (most PVC pipe) and 100,000 psi modulus of elasticity (most PE pipe) pipe material. Figure 1 displays the surge pressures associated with an abrupt valve closure (a final velocity of zero) for two of the PVC pipe classes shown in Table 3 . These pressure levels represent the additional pressure imposed on the system above the normal operating pressure of the system. For example, consider an irrigation system which has a normal operating pressure of 75 psi, and has a water velocity of 7 feet per second in a Class 160 (SDR 26) PVC conduit. Figure 1 shows that a surge pressure of about 100 psi can result from a sudden valve closure with an initial velocity of 7 feet per second (Table 3 can be used to calculate this: 7 x 14.4 = 100.8 psi). This would bring the total pressure in the system to about 175 psi, exceeding the 160 psi pressure rating of the pipe.
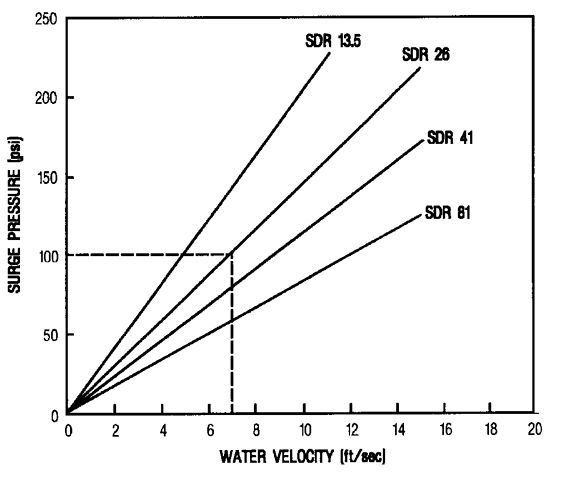
Velocity of Flow
Velocity (V) in a pipeline depends on the volume flow rate of water through the pipeline (gpm) and the cross-sectional area of flow. This basic relationship can be expressed as: Equations, 3a, 3b and 3c all use flow in gallons per minute (gpm), but equation 3a uses area in square feet, 3b uses area in square inches, and 3c uses inside pipe diameter in inches. The inside diameter of the pipe should always be used to determine the area of flow. Table 4 presents inside diameters and cross-sectional areas of PE pipe. Table 5 presents the outside diameters of PVC pipes and inside cross-sectional areas which correspond to pipes of different SDR values.
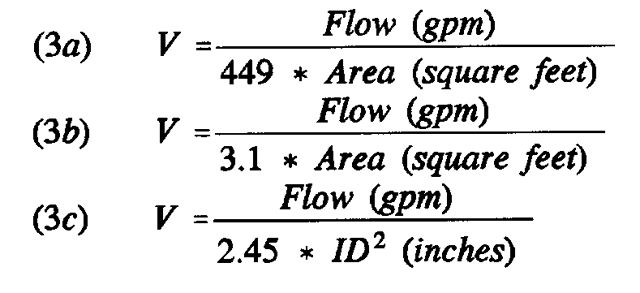
The cross-sectional areas from either Table 4 or Table 5 can be used with the pipe flow rate in gpm with equation 2b to determine the average flow velocity in the pipe. The flow velocity can then be used with Table 3 or Figure 1 to determine the surge pressure associated with a sudden valve closure or other flow restricting action.
Design and Management Considerations
This section discusses design and management criteria to be followed to minimize the effects of water hammer or surge pressures on the irrigation system.
In the design process, pipe should be selected with a pressure rating equal to or greater than the combination of operating plus surge pressures. Be certain to consider temperature effects on the pressure rating of the pipe. If the surge pressures are not known, Table 6 provides recommendations for maximum working pressures for nonthreaded PVC (PVC 1120, PVC 1220, and PVC 2120) and PE 3408 pipe. Table 6 should be used with caution, and only when other data are not available. It is always better to calculate surge pressures for the specific conditions of the site.
To help minimize surge pressures the maximum velocity of water in the pipeline should be limited. General recommendations are to limit maximum operating velocities to 5 per sec. In no case should the velocity exceed 10 per sec.
Entrapped air in a pipeline can cause problems. Air is very compressible and can compress and expand in the pipeline, resulting in varying velocity conditions, and, thus, significant pressure variations. Water-column separation can also produce significant surge pressures due to the high velocities encountered when the column rejoins.
Problems associated with air entrapment can be minimized by preventing air from accumulating in the system. This can be accomplished by using air-relief valves positioned at the high points of the piping system. In areas of relatively flat terrain these should also be used in the vicinity of the pump discharge, near the middle of the line, and at the downstream end of the line.
Additional design considerations include:
- surge arrestors (devices such as small pressure tanks which can absorb shock waves) or automatic pressure reducing valves at flow regulators and at the pump discharge;
- flow controllers used to minimize the rate of filling and to reduce start-up surge in filled lines;
- in cycling systems, design pipelines, if possible, to keep out all air, and then to restart with a filled system.
Water hammer cannot be completely eliminated in an economical design, but, by taking precautions during management and operation, the effects can be minimized. Start-up is critical, especially when pipe lines are empty. Empty lines should be filled as slowly as possible to allow entrapped air to escape. In addition the following cautions should be observed:
- Never completely close the gate valve on the discharge side of a deep-well turbine. This prevents excessive shut-off head from developing.
- Open all manual valves leading to the zones to be irrigated except at the pump discharge. The pump discharge valve should be opened slowly to allow slow filling of the pipe line. Caution should be observed when filling is interrupted and restarted because a quick surge may develop during the restart which could slam into a stationary or slow moving body of water. This situation could result in damaging water hammer pressures, especially if air becomes entrapped between the water fronts. Therefore, follow the same precautions on restart as during initial starting of the system.
- Make sure that all air has been discharged from the system before operating the system at full throttle.
- Close all manual valves slowly. No valve should ever be closed in less than 10 seconds; 30 seconds or more is preferable.
- Use the same precautions in stopping the irrigation system as used in start-up and general operation.
Summary
This publication discussed the causes and effects of water hammer in irrigation systems. Surge pressures experienced from velocity changes were discussed and presented for PVC and PE pipe materials. Guidelines for safe design include selection of the proper size and class of pipe to maintain safe velocities and to have a material which can withstand both operating and surge pressures expected from the system. Components such as air relief and surge arrestors can be positioned at strategic positions in the pipeline to minimize the resultant effects of water hammer in the irrigation system. Additional discussion focused on management considerations to help ensure safe operation.
References
ANSI/ASABE S376.3 FEB2016 (R2020) Standard. Design, Installation and Performance of Underground Thermoplastic Irrigation Pipelines. ASAE Standards 1985. 32nd edition. American Society of Agricultural Engineers, St. Joseph, MI. pp. 478–488.
Daily, J. W. and D. R. F. Harleman. 1966. Fluid Dynamics. Addison-Wesley Publishing Co., Inc. Mass. pp. 341–343.
Pair, C. H., W. W. Hinz, C. Reid and K. R. Frost. 1975. Sprinkler Irrigation, 4th ed. The Irrigation Association, Silver Spring, Maryland. pp. 247–248.
Seipt, W. R. 1974. Water Hammer Considerations for PVC Pipeline in Irrigation Systems. Transactions of the ASAE. pp. 417–423.
Appendix
Surge pressure in a conduit is related to the change in velocity and can be computed from the following formula:
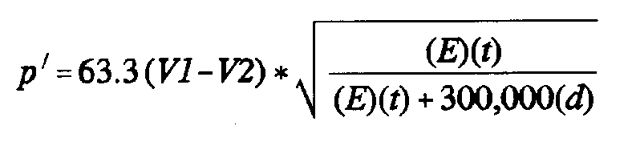
where p'=the resultant rise (surge) in pressure created by the decrease in velocity (psi), V1=the upstream (higher) velocity of water in the pipe (ft/sec), V2=the downstream (lower) velocity of water in the pipe (ft/sec), d=the inside diameter of the pipe (inches), t=the wall thickness of the pipe (inches), and E=the modulus of elasticity of the pipe material (psi), [approx. 400,000 psi for Type I PVC].
As can be seen, the most severe case occurs when V2 becomes zero due to a sudden valve closure or similar action.
This equation calculates the surge pressure that would theoretically occur if the velocity was instantaneously changed from V1 to V2. If a valve is closed slowly, the actual surge pressure will be less than this value. Thus, using this equation provides a safety factor.