Vegetable crops such as tomato (Lycopercicon esculentum Mill.), bell pepper (Capsicum annuum L.), watermelon (Citrullus lanatus (Thumbs.) Mat. & Nakai), summer squash (Cucurbita pepo L.), green bean (Phaseolus vulgaris L), potato (Solanum tuberosum L), and eggplant (Solanum melongena L.) are widely grown in Florida in the winter and spring seasons. The main irrigation methods used for vegetable production are drip irrigation (also called micro-irrigation), sprinkler irrigation (also called overhead irrigation) including center or linear pivot irrigation, and seepage irrigation (also called subirrigation or water table control) (Figure 1). Drip irrigation consists of delivering water to each plant through a network of pipes and drip tubing. Overhead irrigation is made up of pipes, sprinklers, and pivots. Irrigation scheduling of both drip and overhead irrigation can be precisely managed to meet crop demands and maximize crop yields and quality.
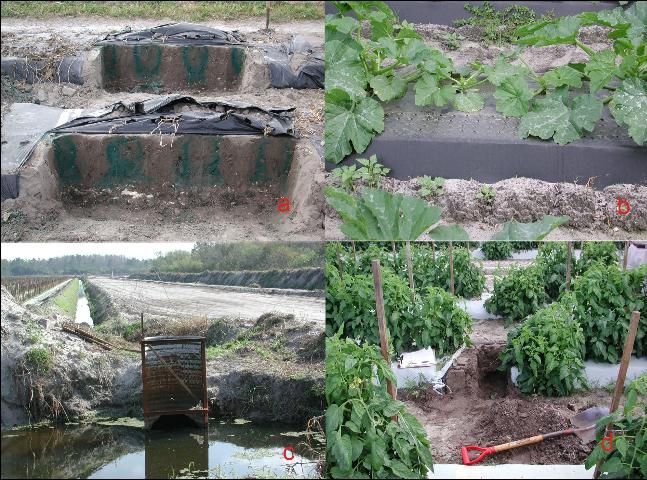
Credit: Eric Simonne, UF/IFAS
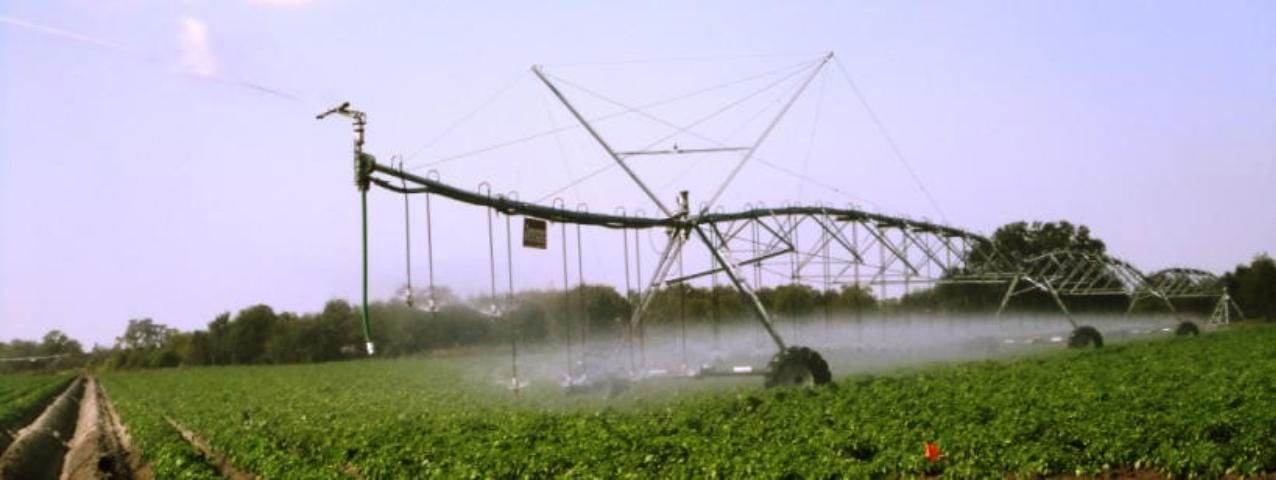
Credit: Guodong Liu, UF/IFAS
Seepage irrigation is the most common irrigation method in Florida on muck and sandy soils and consists of maintaining a water table perched on an impermeable layer. The top of the water table is typically maintained at between 18 and 24 inches (46 and 61 cm) deep. While drip irrigation and overhead irrigation have been gaining popularity in the last twenty years, seepage irrigation remains a very common production system in Florida. In the field, the distinction between seepage irrigation and drip irrigation or overhead irrigation is not always clear, as in most cases, a perched water table is maintained in drip- or overhead-irrigated fields. Because of the sandy soils low water-holding capacity, "true" drip or overhead irrigation (when all the water is provided by the drip tape) is rare in Florida. For example, growers provide two thirds of irrigation water through center pivot irrigation and the other one third through seepage irrigation for potato production in southwest Florida.
These vegetable crops are also grown with intensive fertilization with UF/IFAS N fertilizer recommended rates ranging from 150 to 200 lb/acre (168 to 224 kg/ha) N (Liu and Simonne et al. 2022). For crops grown with drip or overhead irrigation, current recommendations are to use the results of a soil test and to apply a third to a half of the N and K and all the P and micronutrients preplant. The remaining N and K are injected throughout the growing season. When drip or overhead irrigation is used, nutrients move with the water by gravity until the water encounters the impermeable layer. When irrigation water reaches the impermeable layer, lateral water movement occurs (Simonne et al., 2003). For crops grown with seepage irrigation, all nutrients recommended by the soil test results are applied preplant before the plastic is laid. Approximately a third of the N and K and all the P are applied broadcast in the bed (bottom mix), and the remaining N and K are applied in 2 bands located on the bed shoulders (top mix). In this system, water moves upward by capillarity and slowly solubilizes nutrients in the root zone. Heavy rains are common in Florida and may leach nutrients out of the root zone. A leaching rain occurs when it rains at least 3 inches (76 mm) in 3 days or 4 inches in 7 days (Simonne and Hochmuth 2004). After a leaching rain, drip-, overhead-, and seepage-irrigated fields may become flooded. Vegetable crop growth and yield are reduced when anaerobic conditions are maintained for more than 24 hours (Rao and Li 2003). To reduce the incidence of flooding, a network of ditches and canals conveys the water to large pumping stations that can rapidly move it out of the farmed land and into an enclosed retention area where denitrification may occur. Hence, the N fertilizer applied to vegetable fields may be taken up by the crop, denitrified (denitrification is the loss of NO3-N under warm and anaerobic conditions), volatilized (volatilization is the loss of NH4-N under warm and aerobic conditions at soil pH 6.3 or above), or moved off site by leaching rains where, because water is typically pumped into an enclosed retention area, denitrification may also occur (Cockx and Simonne 2003). Vegetable crop fertilizer application rates are often greater than the UF/IFAS recommended rates to ensure adequate fertilization and economical productivity despite these possible N losses.
In response to public awareness of environmental issues, section 303(d) of the Federal Clean Water Act of 1977 (US Congress 1977) requires that states identify impaired water bodies and establish total maximum daily loads (TMDLs) for pollutants entering these water bodies. Best Management Practices (BMPs) were defined as specific cultural practices aimed at reducing the negative environmental impact of agricultural production while maintaining or increasing yield and productivity. In 1987, the Florida legislature passed the Surface Water Improvement and Management (SWIM) Act requiring the development, by the five Florida water management districts of plans to clean up and preserve Florida lakes, bays, estuaries, and rivers. The modification made in 1994 to the Florida Fertilizer Law (Florida Statutes Chapter 576) known as the Nitrate Bill (FS 576.045) established a mechanism to fund projects aiming at protecting the state's water resources by improving fertilizer management practices (Kuhl et al., 1996). In 1999, the Florida Watershed Restoration Act defined a process for the development of TMDLs. The Florida Department of Agriculture and Consumer Services released the "Water Quality/Quantity BMPs for Indian River Area Citrus Groves" in 2000 (Parsons and Bowman, 2006) and the "Florida Vegetable and Agronomic Crop Water Quality and Quantity BMP Manual" in 2015 (Fla. Dept. Ag. Consum. Serv. 2015). Both manuals define the BMPs that will apply to these industries in Florida. Current nutrient BMPs focus on soil testing, plant analyzing, and irrigation scheduling.
The practical impact of fertilization practices on water quality in Florida is not fully understood. It is possible that all the N fertilizer used above the UF/IFAS recommended rate directly contributes to the degradation of water quality. It is also possible that N fertilization rates above current UF/IFAS recommendations are needed in seepage-irrigated soils (and drip- or overhead-irrigated flatwood soils with naturally high-water tables) to offset N loss by denitrification. However, the occurrence and rate of denitrification in vegetable fields is not known. Preservation of water quality through improved N management in vegetable production requires an understanding of the fate of N in vegetable fields, including denitrification. The objectives of this article are to
- describe denitrification and the factors known to affect its rate,
- present current methods available for the measurement of denitrification rate,
- summarize available estimates of denitrification rate, and
- attempt to provide guidelines on how to account for potential denitrification losses in fertilizer programs.
Factors Affecting Denitrification Rate
The N cycle is a set of transformations that affect N in the biosphere, by which N passes from air to soil, to soil organisms, and back to air. Denitrification is defined as the reduction of nitrate (NO3) to gaseous dinitrogen (N2) by a series of reactions in which N goes from NO3 to NO2 (nitrite) to NO (nitric oxide) to N2O (nitrous oxide), and finally to N2 (Payne, 1981). Denitrification can be described as: 4 H+ + 5 (CH2O) + 4 NO3- --> 2 N2 + 5 CO2 + 7 H2O, where NO3 is in the soil solution and N2 is a gas released into the atmosphere. The most prevalent denitrifying bacteria in soils are species of Pseudomonas (especially P. fluorescens) and Alkaligenes (Gamble et al. 1977), but approximately 30 genera have been confirmed to be capable of denitrification (Bryan 1981; Tsai 1989). In the absence of oxygen (anoxic conditions), these heterotrophic bacteria use nitrate as a terminal electron acceptor in their cellular respiration.
The main factors that affect the activity of the denitrifying bacteria are nitrate concentration (Fillery 1983), soil organic matter content (Brettar and Hofle 2002; Hahdel and Isermann 1992; Tsai 1989), moisture level (Tsai 1989; Weir et al. 1993), oxygen concentration (Fillery 1983), pH (Müller et al. 1980), and soil temperature (Mahli et al. 1990; Standford et al. 1975). In field conditions, these factors tend to act together, and it is often difficult to measure the specific effect of each of them. The simultaneous occurrence of favorable factors for the growth of the denitrifying bacteria will result in a bacterial population increase and a subsequent increase in denitrifying activity.
Nitrate concentration does not limit denitrification at concentrations greater than 1.4 grain/gallon (17.1 mg/L) (Paul and Clark 1989). Denitrification rate increases with increasing NO3 supply to a maximum and then declines with further increase in NO3. The decline may be resulted from high NO3 content inhibiting the enzymatic reduction of NO to N2O (Sigunga et al. 2002; 2007). Nitrate is the preferred N-form by most vegetables and the optimum NO3-N: NH4-N ratio is 3:1 (Barker and Mills 1980). Hence, the presence of NO3 in a field (from the application of NO3-containing fertilizer or from the conversion of NH4 to NO3 by nitrification) may stimulate denitrification in situations where N supply was the factor limiting denitrification.
In soils, denitrifying activity is highly correlated with water-extractable organic carbon and is frequently stimulated by the addition of exogenous carbon (Knowles 1982; Hahndel and Isermann 1993). Different organic compounds which support equal rates of denitrification may give different fractions of N2O in the products, suggesting that they may exert different effects on the enzymes involved (Knowles 1982; Reddy et al. 1982). Denitrification studies in columns on soils from Florida have found that when soil organic matter content is less than 0.91%, it becomes the limiting factor for denitrification, when NO3 supply is not limiting (Tsai 1989). In Florida, soil organic matter content may range from 1% to 2% in sandy soils to 40% to 60% in organic muck soils. Hence, soil organic matter content is not likely to limit carbon availability for the growth of denitrifying bacteria in sandy soils. (Moreover, the confining layer beneath south Florida Spodosols is highly organic). The incorporation of crop residues from vegetables or cover crops, or the application of manure or compost amendments increased soil organic matter content (Clark et al. 1995; Mahmood et al. 1997, 1998, 1999; Ozores-Hampton et al. 1994). However, increasing C:N ratio of the organic matter source tends to reduce the denitrification rate. In incubation studies, denitrification rate was highest with vetch (Vicia illosa Roth.) residues (C:N of 8) than with soybean (Glycine max [L.] Merr) (C:N of 43), corn (Zea mays L.) (C:N of 39), and wheat (Triticum aestivum L.) residues (C:N of 82) (Aulakh et al. 1991b). Information on the effect of crop residue from cover crops used in Florida such as sorghum Sudangrass (Sorghum bicolor (L.) Moench) or sunhemp (Crotalaria ochroleuca) on denitrification rates is currently limited.
Denitrification cannot occur in aerobic conditions. Concentrations of dissolved oxygen above 0.2 parts per million, ppm (0.2 mg/L) suppressed denitrification (Pesek et al. 1971). With constant soil water content, denitrification rate increases with decreasing oxygen concentration. With constant oxygen concentration, denitrification rate increases with water content because bacteria must use oxygen in NO3 instead of soluble O2 (Knowles 1982). In soils, the presence of oxygen and moisture content are linked because soil pores are either filled with water (anaerobic pore) or air (aerobic pores). Hence, soil texture and soil compaction affect denitrification by influencing the tortuosity of soil pore space, hence, the diffusion of substrates to, and products from, the microsites where denitrification occurs. An increase of the water-filled pore space causes a decrease of the oxygen concentration level in the soil, which favors anoxic conditions and hence, an increase in denitrification rate. Denitrification was reported to occur at low levels when soil moisture content was below 60% (Nishio et al. 1988) and was reduced when soil moisture content was below 90% (Craswell and Martin 1974). In the absence of rain, the seepage-irrigated soils of Florida can be characterized by decreasing water content from the impermeable spodic layer (the spodic layer is an organic/iron/alumina complex) to the surface of the soil. Hence, the soil just above the impermeable layer is constantly saturated and anaerobic, which favors denitrification. Alternating or contiguous aerobic and anaerobic conditions stimulate concurrent nitrification and denitrification, which may result in greater total N loss from the soil than would be found under continuous anaerobic conditions (Aulakh et al. 1991b).
pH affects denitrification rate and the type of N form released. Denitrification rate was very low at pH = 4.1, increased with increasing pH, and was very rapid in the pH range of 7.5 to 8.2 (Pesek et al. 1971; Müller et al. 1980). As pH decreases below 7, nitric (NO) and nitrous oxides (N2O) become the dominant by-product, while N2O and N2 are the dominant by-products at pH above 7 (Bryan 1981). Hence, liming of agricultural soils or using alkaline irrigation water favors the activity of denitrifying bacteria.
Temperature influences the activity of bacteria and the solubility of oxygen in water and therefore affects denitrification. Temperature also affects the product of denitrification (Aulakh and Rennie 1984). When temperature increases, the solubility of oxygen decreases, which favors denitrification. Denitrification rate is negligible at 38°F to 45°F (3°C to 7°C), increases with increasing temperature to a highest rate occurring between 145°F to 175°F (63°C to 79°C), and ceases at temperatures above 195°F (91°C) (Knowles 1982). Denitrification rates measured on columns of an EauGallie fine sand were 5.65, 28.68, and 51.44 ng/g /hour N2O-N at 25°F, 35°F, and 45°F, respectively (Espinoza 1997). In Florida, soil temperature at the 4-inch (10 cm) depth typically ranges between 60°F and 80°F (16°C and 27°C) during the cropping season (http://fawn.ifas.ufl.edu). Mulching with black plastic polyethylene film increases soil temperature in early spring and late fall to near the optimum range for microbial activity (Thiagalingam and Kanchiro 1973; Kowalenko and Cameroun 1976; Shinde et al. 2001). Except for potato and green bean, vegetable crops are typically grown with polyethylene mulch in south Florida (Olson 2004). Hence, denitrification rates in unmulched green bean or potato fields may be lower than those in adjacent mulched fields.
Soil fumigants are often used in vegetable production to reduce soilborne pathogens. Broad-spectrum fumigants such as Telone C-35 (1,3-dichloropropene) or chloropicrin also reduce all the levels of denitrifying bacteria. During the three weeks following fumigation, denitrification may occur at reduced rates, or may not occur at all. During most of the growing season, the conditions in Florida's irrigated soils are overall conducive to denitrification:
- Nitrogen and nitrate levels are high.
- Organic matter is incorporated in the tillage zone thereby supplying a carbon source.
- The water gradient above the impermeable layer creates aerobic and anaerobic conditions in proximity to one another.
- Soil pH is between 6 and 7.
- Soil temperature is between 60°F and 80°F (16°C and 27°C) during most of the year.
While all these factors contribute to denitrification, spatial and temporal variability of these factors affect the actual denitrification rate. Moreover, the actual denitrification rate cannot exceed the rate allowed by the most limiting factor. Therefore, actual denitrification rates and potential denitrification rates may be different in the field.
Methods for Measuring Denitrification Rate
The Association of Official Analytical Chemists (AOAC International) has yet to approve a method to measure denitrification in soils. Current methods used to quantify denitrification come from different scientific domains, such as ecology, agriculture, and industrial engineering, and reflect different interests in different aspects of denitrification. Studies comparing different methods to determine denitrification reported different denitrification rates based on methodology used (Aulakh et al. 1991a; Keeney 1986; Mahmoud et al. 1999). Hence, the appropriate method should be identified and selected before measurements begin. Methods used to measure denitrification may be grouped in two types: the indirect methods and the direct methods. The three most used indirect methods are based on nitrate disappearance, nitrate/chloride ratios, and N balances (Table 1). The direct methods used to determine denitrification activity in fields include isotopic methods (Sidle and Goodrich 2002), acetylene inhibition (Knowles 1990), gaseous diffusion (Hauck and Weaver 1986), prediction models that use micrometeorological data (Hauck and Weaver 1986) or simple field measurements (Rodriguez and Giambiagi 1995), and computer simulation (Lin et al. 2000; FAO 2001). Among these direct methods, the acetylene inhibition technique (AIT) is the most widely used with agricultural soils and can be used in the laboratory as well as in the field (Aulakh et al. 1991a).
The enzyme nitrous oxide reductase normally catalyzes the conversion of N2O into N2. When its activity is inhibited by acetylene (C2H2), N2O accumulates. As N2O concentration in the air is much lower than that of N2, it can then be quantified by gas chromatography with negligible background interferences (Knowles 1990). The protocol for the AIT includes:
- fabrication of a denitrification potential solution (containing sodium succinate and potassium nitrate),
- mixing the soil sample with the denitrification potential solution in an air-tight capped Erlenmeyer flask,
- adding ethylene into the flask,
- calculating the volume of the headspace in the flask,
- keeping the flask continuously agitated to prevent effects of diffusion,
- taking air space samples with a syringe, and
- injecting the head-space sample in a gas chromatograph (Knowles 1990).
The advantages of the AIT include an increase in sensitivity compared to other methods, the use of natural nitrate substrate pool, the possibility to automate and analyze large number of samples, and a relatively low cost compared to the other methods. While simple and versatile, the AIT has some limits:
- Acetylene is a poor nitrous-oxide reductase inhibitor at low nitrate concentrations.
- Acetylene may inhibit nitrification.
- Acetylene may be metabolized by soil microorganisms.
- Contaminants may be present in the acetylene (Keeney 1986; Knowles 1990).
Therefore, the AIT method is better suited for short-term measurements of denitrification.
Direct and indirect methods may be used to measure denitrification in the field or in the laboratory. However, it is accepted that three conditions should be met to make valid estimates of field denitrification rates from laboratory measurements. First, the internal environment of the experimental apparatus should be subjected for the duration of the experiment to the same episodic or seasonally cyclical changes that occur in the external environment of the field site. Also, the soil substrate being studied should have inherent heterogeneity and natural properties like those of the soil at the field site. Finally, the monitoring and measuring devices and sampling methods should not produce artifacts or create artificial conditions that may alter soil processes.
Progress in understanding and quantifying denitrification has been limited by the lack of uniformity in approaches and standardization in units used to report denitrification rate. Singunga (2003) proposed that soil samples for estimation of potential denitrification should be taken under saturated conditions (140% moisture level), in the presence of excess NO3 and C, and on undisturbed soil cores; soil temperature (values and fluctuations) and pH should also be cited. The reporting unit for denitrified N should be expressed in parts per million, ppm, (mg/kg) N per hour. Soil mass should be reported on a dry weight (oven-dry) basis.
Compilation of Available Estimates of Denitrification Rates
Denitrification estimates found in the literature from short-term studies (few days) from worldwide ecosystems are overall in good agreement (Table 2). Short-term studies reported denitrification rate in soils from unfertilized areas of 57.6 ppm per day (57.6 mg /kg /day) N in a hardwood forest (Brettar and Hofle 2002) and 0.03 to 0.07 ppm (mg/kg/ha/day) N for uncultivated land (Ryden 1985). Other short-term studies reported denitrification rates in highly fertilized agricultural fields (with fertilization rates of 179 to 536 lb/acre/year, or 200 to 600 kg/ha/year N) ranging from 0 to 1 ppm per day (0 to 3 kg/ha/day) N (Ryden and Rolston 1983). However, no consensus may be found in published denitrification estimates when all estimates are converted to the same unit and on a yearly basis. By compiling and transforming 94 denitrification rates found in the literature, the average yearly denitrifcation rate (followed by its standard error) was 171.4 ± 272.3 lb/acre/year (192 ± 305 kg /ha/year) N. These results show that reliable daily estimates of denitrification are available, but their occurrence on a year-round basis is poorly represented by the extrapolation of short-term estimates. There is no guarantee that a denitrification rate measured over a short period will be sustained over a long period of time.
Few denitrification estimates are available from vegetable fields in Florida. Due to the differences of the environment, N rate, soil organic matter content, and the crop tested, the estimates from different sources are usually not comparable. There are research data available in the literature. Approximately 23% of the N fertilizer was unaccounted for in an N balance made on bell pepper grown in lysimeters (Stanley and Clark 1993). The N not accounted for was assumed to be lost by denitrification. As the fertilizer rate used in this experiment was 300 lb N/acre (336 kg/ha), the potential denitrification estimate from this study was 25.7 ppm (77 kg/ha) per season. In another study, denitrification rate was measured every two weeks on undisturbed soil cores from an EauGallie fine sand with the acetylene inhibition technique (Espinoza 1997). Soil cores were collected from a field where two tomato crops (spring crop between February and June; fall crop between August and December) were grown with seepage irrigation and fertilized each with an N rate of 200 lbs/acre (224 kg/ha). Actual denitrification rates ranged between 1.012 to 0.016 ppm per day (33.6 to 45.6 g N/ha/day). Actual denitrification measurements were consistently greater than the ones predicted by the LEACHN model (Espinosa 1997). However, these two denitrification estimates are of limited practical use to help predict the importance of denitrification in designing fertilizer programs for vegetables. The denitrification estimate from lysimeter-grown bell pepper was obtained by difference (Stanley and Clark 1993) and is likely to over-represent denitrification rate in the field due to the accumulation of error in the fraction determined by difference. The depth of soil sampling in the tomato field was 0–8 inches (0–20 cm) (Espinoza 1997). A shallow sampling depth was used in this study to assess the effect of sludge amendment incorporated in the top 8 inches (20 cm) of soil on denitrification. Air content, and thereby oxygen availability in surface soil is much greater than that of the deeper soil layers. Hence, the denitrification estimate was largely underestimated from the tomato field (Espinoza 1997). These results have some implications on fertilizer recommendation and nutrient management. First, denitrification estimates currently available in the literature were made in studies in which the focus was not fertilization management. Hence, it is unlikely that any of them truly represent field-scale denitrification rates for a whole growing season. Therefore, there is no basis for systematically increasing fertilizer applications by amounts that poorly represent denitrification. The second consequence is that fertilizer recommendations need to be based on fertility trials conducted under conditions like those of production. Even if denitrification rate is not determined, it is at least factored into the recommendation. Hence, fertilizer recommendations may be higher in denitrification-prone areas (where seepage irrigation is used) than in other areas (deep sandy soils) for similar varieties, production seasons, and yield goals. In addition, improved N management may be achieved through regular monitoring of crop nutritional status by using whole-leaf analysis or fresh petiole-sap testing. With the difficulties associated with long-term field measurement of denitrification, denitrification may be indirectly determined through complete N balances that would include measurement of the different N fractions (crop removal, immobilization, and mineralization to and from organic matter, and leaching). Another implication of denitrification on fertilizer management is temporary flooding during the off season. Summer rains often result in the complete flooding of the vegetable fields. Although specific data are not available, it is likely that residual N may be denitrified at that time. As it reduces the potential for N loss to the ground and surface water, maintaining conditions favorable for denitrification during non-cropped periods could become a possible BMP on flatwood soils.
Literature Cited
Aulakh, M.S. and D.A. Rennie. 1984. "Transformation of Fall-Applied Nitrogen Labeled Fertilizers." Soil Sci. Soc. Amer. J. 48:1184–1189. https://acsess.onlinelibrary.wiley.com/doi/abs/10.2136/sssaj1984.03615995004800050047x. accessed on February 5, 2024.
Aulakh, M.S., J.W. Doran, and A.R Mosier. 1991a. "Field Evaluation of Four Methods for Measuring Denitrification." Soil. Sci. Soc. Amer. J. 55:1332–1338. https://acsess.onlinelibrary.wiley.com/doi/abs/10.2136/sssaj1991.03615995005500050023x. Accessed on February 5, 2024.
Aulakh, M.S., J.W. Doran, D.T. Walters, A.R. Mosier, and D.D. Francis. 1991b. "Crop Residue Type and Placement Effects on Denitrification and Mineralisation." Soil Sci. Soc. Amer. J. 55:1020–1025. https://acsess.onlinelibrary.wiley.com/doi/abs/10.2136/sssaj1991.03615995005500040022x. Accessed on February 5, 2024.
Barker, D.A. and H.A. Mills. 1980. Ammonium and Nitrate Nutrition of Horticultural Crops. pp. 395–423, In: J. Janick (ed.) Horticultural Reviews Vol. 2, Avi Pub., Westport, CT. https://agris.fao.org/search/en/records/64735e7ce17b74d22252c08c. Accessed on February 5, 2024.
Brettar, I. and M.G. Höfle. 2002. "Close Correlation Between the Nelimination Rate by Denitrification and the Organic Matter Content in Hardwood Forest Soils of the Upper Rhine Floodplain (France)." Wetlands 22(2):214–224. https://link.springer.com/article/10.1672/0277-5212(2002)022[0214:CCBTNE]2.0.CO;2. Accessed on February 5, 2024.
Bryan, B.A. 1981. Physiology and Biochemistry of Denitrification, pp. 127–165, In: C.C. Dlwiche (ed.) Denitrification, Nitrification, and Anitrous Oxide, John Willey & Sons, New York, NY.
Clark, G.A., C.D. Stanley, and D.N. Maynard. 1995. "Municipal Solid Waste Compost in Irrigated Vegetable Production." Soil Crop Sci. Soc. Fla. Proc. 54:49–53.
Cockx, E.M. and E.H. Simonne. 2017. Reduction of the Impact of Fertilization and Irrigation on Processes in the Nitrogen Cycle in Vegetable Fields with BMPs. HS948. Gainesville: University of Florida Institute of Food and Agricultural Sciences. https://edis.ifas.ufl.edu/HS201. Accessed on February 5, 2024.
Craswell, E.T. and A.E. Martin. 1974. "Effect of Moisture Content on Denitrification in a Clay Soil." Soil Biol. Biochem. 6:127–129. https://www.sciencedirect.com/science/article/pii/0038071774900728. Accessed on February 5, 2024.
De Datta, S.K, 1995. "Nitrogen Transformations in Wetland Rice Ecosystems." Fert. Res. 42:193–203. https://link.springer.com/article/10.1007/BF00750514. Accessed on February 5, 2024
Espinoza, L.A. 1997. Fate of Nitrogen and Metals Following Organic Waste Applications to Some Florida Soils. Ph.D. Dissertation, Univ. of Fla., Gainesville, FL, 152 pp.
FAO. 2001. Global Estimates of Gaseous Emissions of NH3, NO, and NO2 from Agricultural Land, Intl. Fert. Industr. Assoc., FAO, Rome, Italy. http://www.fao.org/3/Y2780E/y2780e00.htm#P-1_0. Accessed on February 5, 2024.
Fillery, I.R.P. 1983. Biological Denitrification, pp. 33–64, In: J.R. Freney and J.R. Simpson (eds.) Gaseous loss of Nitrogen from Plant-Soil Systems, Marinus Nijhoff, Boston, MA.
Fla. Dept. Ag. Consum. Serv. 2015. Florida Vegetable and Agronomic Crop Water Quality and Quantity BMP Manual. Fla. Dept. Ag. Consum. Serv., Tallahassee, FL. https://www.fdacs.gov/content/download/77230/file/vegAgCropBMP-loRes.pdf. Accessed on February 5, 2024.
Gamble, T.N., M.R. Betlach, and J.M. Tiedje. 1977. "Numerically Dominant Denitrifying Bacteria from World Soils." Appl. Environ. Microbiol. 33:926–939. https://www.ncbi.nlm.nih.gov/pmc/articles/PMC170793/. Accessed on February 5, 2024.
Hahndel, R. and K. Isermann. 1992. "Soluble Nitrogen and Carbon in the Subsoil in Relation to Vegetable Production Intensity." Acta Hort. 339:193–206. https://www.actahort.org/books/339/339_17.htm. Accessed on February 5, 2024.
Hauck, R.D and R.W Weaver. 1986. "Field Measurement of Dinitrogen Fixation and Eenitrification." Soil Sci. Soc Amer. Spec. Pub. 18, Madison, WI. https://acsess.onlinelibrary.wiley.com/doi/abs/10.2136/sssaspecpub18.c5. Accessed on February 5, 2024.
Jackson, L.E., L.J. Stivers, B.T. Warden, and K.K. Tanji. 1994. "Crop Nitrogen Utilization and Soil Nitrate Loss in a Lettuce Field." Fert. Res. 37:93–105. https://link.springer.com/article/10.1007/BF00748550. Accessed on February 5, 2024.
Keeney, D.R. 1986. Critique of the Acetylene Blockage Technique for Field Measurement of Denitrification. In: Hauck, R.D. and R.W. Weaver (eds.). "Field Measurements of Dinitrogen Fixation and Denitrification." Soil Sci. Soc. Amer. Spec. Pub. 18, Madison, WI.
Knowles, R. 1982. Denitrification in Soils, pp. 246-266, In: N.S. Subba Rao (ed.) Advances in Agricultural Microbiology, Butterworth Sci. Pub., London, UK.
Knowles, R. 1990. Acetylene Inhibition Technique: Development, Advantages, and Potential Problems, pp. 151–166, In: N.P. Revsbech and J. Sorensen (eds.) Denitrification in Soil and Sediment. New York, NY: Plenum Press.
Kowalenko, C.G. and D.R. Cameroun. 1976. "Nitrogen Transformation in an Incubated Soil as Affected by Combinations of Moisture, Content and Temperature and Adsorption Fixation of Ammonium." Can. J. Soil Sci. 56: 63–77. https://cdnsciencepub.com/doi/10.4141/cjss76-010. Accessed on February 5, 2024.
Kuhl, K.A., R.J. Budell, and B.L. McNeal. 1996. "Nitrogen BMP Program Implementation." Soil Crop Sci. Soc. Fla. Proc. 55:67–70.
Lin C., C.Chen, W.Hsiang, and T.Hu. 2000. "A Model and Its Implications for Denitrification in Soil Environment." Proc. Natl. Sci.Counc. 24(3):136–142. https://pubmed.ncbi.nlm.nih.gov/10943947/. Accessed on February 5, 2024.
Liu, G.D., Simonne, E.H. and G.J. Hochmuth. 2018. Soil and Fertilizer Management for Vegetable Production in Florida. HS711. Gainesville: University of Florida Institute of Food and Agricultural Sciences. https://edis.ifas.ufl.edu/cv101. Accessed on February 5, 2024.
Liu, G.D., E.H. Simonne, K.T. Morgan, G.J. Hochmuth, S. Agehara, R. Mylavarapu. Fertilizer management for Vegetable Production in Florida. Vegetable Production Handbook for Florida. 2022. Publication# CV296. Gainesville: University of Florida Institute of Food and Agricultural Sciences. https://edis.ifas.ufl.edu/cv296. Accessed on February 5, 2024.
Mahli, S.S., W.B. McGill, and M. Hyborg. 1990. "Nitrate Losses in Soil: Effect of Temperature, Moisture and Substrate Concentration." Soil Bio. Biochem. 22:773–787. https://www.sciencedirect.com/science/article/pii/003807179090150X. Accessed on February 5, 2024.
Mahmood, T., R. Ali, K.A. Malik, and S.R.A. Shamsi. 1997. "Denitrification with and without Maize Plants (Zea mays L.) Under Irrigated Field Conditions." Biol. Fertil. Soil. 24:323–328. https://link.springer.com/article/10.1007/s003740050251. Accessed on February 5, 2024.
Mahmood, T., G.R. Tahir, K.A. Malik, and S.R.A. Shamsi. 1997. "Denitrification Losses from an Irrigated Sandy Clay Loam Under Wheat Maize Cropping System Receiving Different Fertilizer Treatments." Biol. Fertil. Soil. 26:35–42. https://link.springer.com/article/10.1007/s003740050340. Accessed on February 5, 2024.
Mahmood T., Ali R., F. Azam, and K.A. Malik. 1999. "Comparison of Two Versions of the Acetylene Inhibition/soil Core Method for Measuring Denitrification Loss from an Irrigated Wheat Field." Biol. Fertil. Soil. 29:328–331. https://link.springer.com/article/10.1007/s003740050560. Accessed on February 5, 2024.
Müller, M.M, V. Sundman, and Skujins. 1980. "Denitrification in Low pH Spodosols and Peats Determined with the Acetylene Inhibition Method." Environ. Microbiol. 40(2):235–239. https://www.ncbi.nlm.nih.gov/pmc/articles/PMC291558/. Accessed on February 5, 2024.
Nishio, T., T. Kanamori, and T. Fujimoto. 1988. "Effects of Organic Matter, Moisture Content and Other Environmental Factors on Denitrification in Top Soils of an Upland Field." Soil Sci. Plant Nutr. 34:97–105. https://www.tandfonline.com/doi/abs/10.1080/00380768.1988.10415583. Accessed on February 5, 2024.
Ozores-Hampton, M., B. Schaffer, H.H. Bryan, and E.A. Hanlon. 1994. "Nutrient Concentrations, Growth, and Yield of Tomato and Squash in Municipal Solid-waste-amended Soil." HortScience 29(7):785–788. https://journals.ashs.org/hortsci/view/journals/hortsci/29/7/article-p785.xml. Accessed on February 5, 2024.
Parsons, L. and B. Bowman. 2006. Best Management Practices for Florida Citrus. HortTechnology 16(3): 389-393.
Paul, E.A. and F.E. Clark. 1989. Soil Microbiology and Biochemistry. San Diego, CA: Academic Press.
Payne, W.J. 1981. Denitrification. New York, NY: John Wiley & Sons.
Pesek, J., G. Stanford, and N.L. Case. 1971. Nitrogen Production and Use, pp. 217–269, In: R.A. Olson (ed.) Fertilizer Technology and Use, Soil Sci. Soc. Amer. Madison, WI.
Pratt, P.F., L.J. Lund, and J.M. Rible. 1978. An Approach to Measuring the Leaching of Nitrate from Freely Drained Irrigated Fields, p.223–256, In: D.R. Nielsen and J.G. MacDonald (eds.) Nitrogen in the Environment, Vol. 1, Academic Press, New York, NY.
Rao, R. and Y. Li. 2003. "Management of Flooding Effects on Growth of Vegetable and Selected Field Crops." HortTechnology 13(4):610–616. https://journals.ashs.org/horttech/view/journals/horttech/13/4/article-p610.xml. Accessed on February 5, 2024.
Reddy, K.R., P.S.C. Rao, and R.E. Jessup. 1982. "The Effect of Carbon Mineralization on Denitrification Kinetics in Mineral and Organic Soils." Soil Sci. Soc. Amer. J. 46:62–68. https://acsess.onlinelibrary.wiley.com/doi/abs/10.2136/sssaj1982.03615995004600010011x. Accessed on February 5, 2024.
Rodriguez, M.B. and N. Giambiagi. 1995. "Denitrification in Tillage and no Tillage Pampean Soils: Relationships among Soil Water, Available Carbon, and Nitrate and Nitrous Oxide Production. Commun." Soil Sci. Plant Anal. 26(19&20):3205–3220. https://www.tandfonline.com/doi/abs/10.1080/00103629509369521. Accessed on February 5, 2024.
Ryden, J.C. 1985. Denitrification Loss from Managed Grassland, pp. 121-134, In: H.L. Golterman (ed.) Denitrification in the Nitrogen Cycle. New York, NY: Plenum Press.
Ryden, J.C. and D.E. Rolston. 1983. The Measurement of Dentrification, pp. 91–132, in: J.R. Freney and J.R. Simpson (eds.) Gaseous Loss of Nitrogen from Plant-soil Systems, Martinus Nijoff, The Hague, The Netherlands.
Shinde, D., R.S. Mansell, A.G. Hornsby, and M. Savabi. 2001. "The Hydro-thermal Environment in Plastic-mulched Soil Beds: Modelling Analysis." Soil Crop Sci. Soc. Fla. Proc. 60:64–71. https://www.researchgate.net/publication/285818755_The_hydro-thermal_environment_in_plastic-mulched_soil_beds_Modeling_analysis. Accessed on February 5, 2024.
Sidle W.C and J.A Goodrich. 2002. Denitrification Efficiency in Groundwater Adjacent to Ditches within Constructed Riparian Wetlands: Kankakee Watershed, Illinois-Indiana, U.S.A. https://link.springer.com/article/10.1023/A:1022962127801. Accessed on February 5, 2024.
Sigunga, D.O., B.H. Janssen, and O. Oenema. 2002. "Denitrification Risks in Relation to Fertilizer Nitrogen Losses from Vertisols and Phaoezems." Commun. Soil. Sci. Plant Anal. 33(3&4):561–578. https://www.tandfonline.com/doi/full/10.1081/CSS-120002764. Accessed on February 5, 2024.
Sigunga, D.O. 2007. "Potential denitrification: Concept and Conditions of Its Measurement." Commun. Soil Sci. Plant Anal. 34(17&18):2405–2418. https://www.tandfonline.com/doi/full/10.1081/CSS-120024776. Accessed on February 5, 2024.
Simonne, E.H., D.W. Studstill, R.C. Hochmuth, G. McAvoy, M.D. Dukes, and S.M. Olson. 2003. "Visualization of Water Movement in Mulched Beds with Injections of Dye with Drip Irrigation." Proc. Fla. State Hort. Soc. 116:88–91.
Standford, G., S. Dzienia, and R.A. Vander Pol. 1975. "Effects of Temperature on Denitrification Rate in Soils." Soil Sci. Soc. Am. Proc. 39:860–870. https://acsess.onlinelibrary.wiley.com/doi/abs/10.2136/sssaj1975.03615995003900050024x. Accessed on February 5, 2024.
Stanley, C.D. and G.A. Clark. 1993. "Water Use and Nitrogen Balance for Subirrigated Fresh-market Bell Pepper Production." Proc. Fla. State Hort. Soc. 106:202–204.
Thiagalingam, K. and Y. Kanchiro. 1973. "Effects on Temperature on Nitrogen Transformation in Hawaiian Soils." Plant & Soil 38:177–189. https://link.springer.com/article/10.1007/BF00011225. Accessed on February 5, 2024.
Tsai, Y.H. 1989. Factors Affecting Denitrification Kinetics in Selected Florida Soils, Univ. of Fla. Masters Thesis, Gainesville, FL.
U.S. Congress. 1977. Federal Clean Water Act, Title 33, Chapt.26. https://en.wikisource.org/wiki/United_States_Code/Title_33/Chapter_26. Accessed on February 5, 2024.
Weier, K.L., J.W. Doran, J.F. Power, and D.T. Walters. 1993. "Denitrification and the Dinitrogen/nitrous Oxide Ratio as Affected by Soil Water, Available Carbon, and Nitrate." Soil Sci. Soc. Amer. J. 57:66–72. https://acsess.onlinelibrary.wiley.com/doi/abs/10.2136/sssaj1993.03615995005700010013x. Accessed on February 5, 2024.