The purpose of this publication is to provide information on managing Bemisia tabaci and tomato yellow leaf curl virus to growers, UF/IFAS Extension agents, and other crop protection professionals.
Nomenclature: A Whitefly by Any Other Name
Bemisia tabaci MEAM1 (Gennadius) (Hemiptera: Aleyrodidae), the sweetpotato whitefly (Figure 1), is commonly referred to as the silverleaf whitefly in Florida because of a disorder this pest produces in cucurbits. Prior to its present species designation of B. tabaci MEAM1 (an abbreviation for Middle East Asia Minor 1), it was referred to as the B biotype of B. tabaci after briefly being considered a separate species, Bemisia argentifolii. All these names can be found in the scientific literature. Globally, B. tabaci is thought to consist of dozens of cryptic species. Cryptic species appear essentially identical, but cannot interbreed and differ in aspects of biology, including the plants they can develop on (host range) and the tendency to develop resistance to insecticides. Bemisia tabaci MED (for Mediterranean, also referred to as the Q biotype whitefly) has been established in the Florida landscape since at least 2016 and is an example of a cryptic Bemisia tabaci species along with B. tabaci MEAM1 (McKenzie and Osborne 2017).
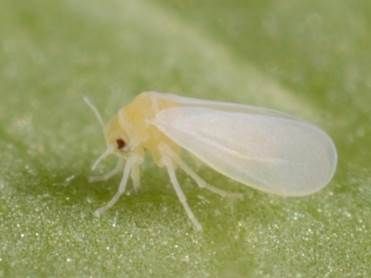
Credit: Lyle Buss UF/IFAS
Host Range
The sweetpotato whitefly became established in Florida in the late 1980s, and quickly displaced the strain of B. tabaci then present in the state, referred to as the A biotype. The A biotype had been an occasional pest of lettuce (Lactuca sativa) and other vegetables in Florida but was not considered a major constraint on vegetable production. The sweetpotato whitefly has a much broader host range than the endemic A biotype of B. tabaci and has become a serious pest of tomato (Solanum lycopersicon), eggplant (Solanum melongena), watermelon (Citrullus lanatus) and other cucurbits, bean (Phaseolus vulgaris) and many varieties of ornamental plants produced in Florida. It also infests field crops, including peanut (Arachis hypogaea) and cotton (Gossypium hirsutum), as well as many weeds. The sweetpotato whitefly can reproduce on important crops including cabbage (Brassica oleracea) and potato (Solanum tuberosum) without routinely reaching damaging levels. In these instances, growers may not attempt to manage the sweetpotato whitefly, with the result that the crops serve as a source of whitefly infestation for more vulnerable host crops like tomato, squash, cotton, and ornamental plants.
Life Cycle
The sweetpotato whitefly passes through an egg stage and four nymphal instars before becoming an adult. Eggs are football shaped and oviposited on the surface of the leaf, usually on the underside (Figure 2). They are initially pearly green and darken to a black coffee color before hatching. The whitefly nymph that emerges from the egg is called a crawler because it has functional legs and moves away from the egg in search of a suitable feeding site (Figure 2). Once the crawler settles down and molts to the second instar, it no longer has functioning legs and is sessile (immobile). In the later stage of the fourth nymphal instar, the red eyes of the developing adult are evident. This stage is sometimes called the red-eyed nymph stage and sometimes the pupal stage (Figure 3). When the adult emerges from the exuviae (caste skin) of the fourth instar, it leaves a characteristic T-shaped slit (Figure 3). Whitefly males are slightly smaller than females. Whiteflies can reproduce both sexually and asexually. When whitefly females reproduce without mating, referred to as parthenogenesis, the offspring are all male. Development from egg to adult usually requires about three weeks, depending on temperature and host plant. At 25°C and 65% relative humidity, the egg stage lasts about seven days on tomato, and the insects need about 15 days to pass through the four nymphal instars and reach adulthood (Salas and Mendoza 1995).
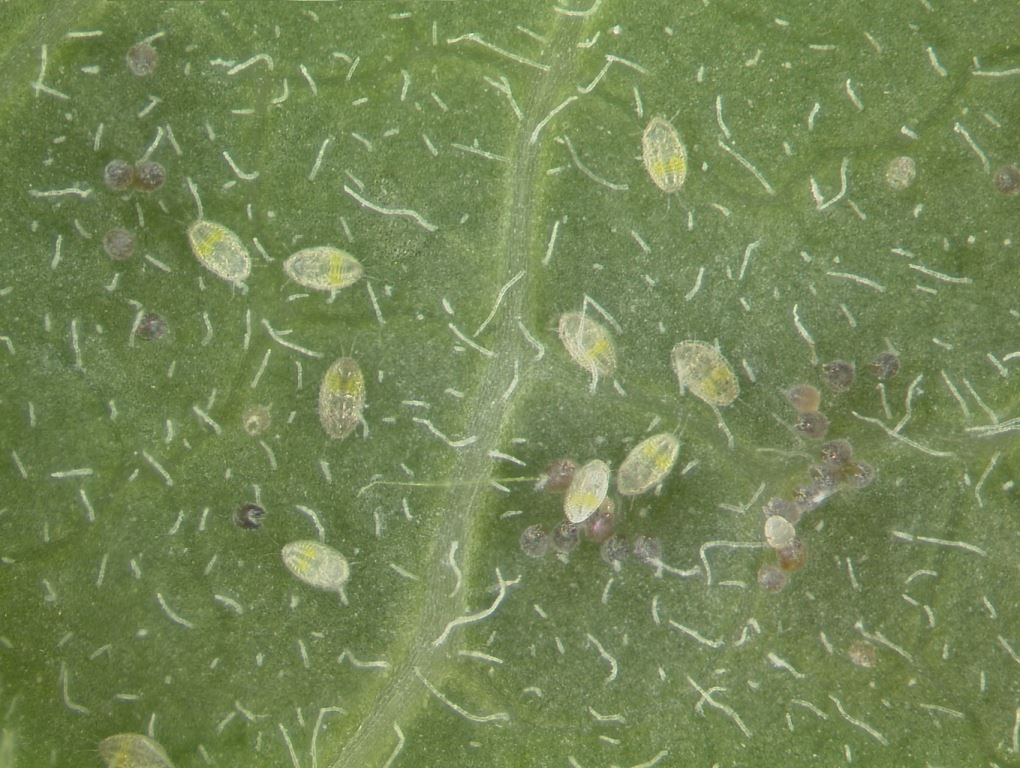
Credit: Lyle Buss UF/IFAS
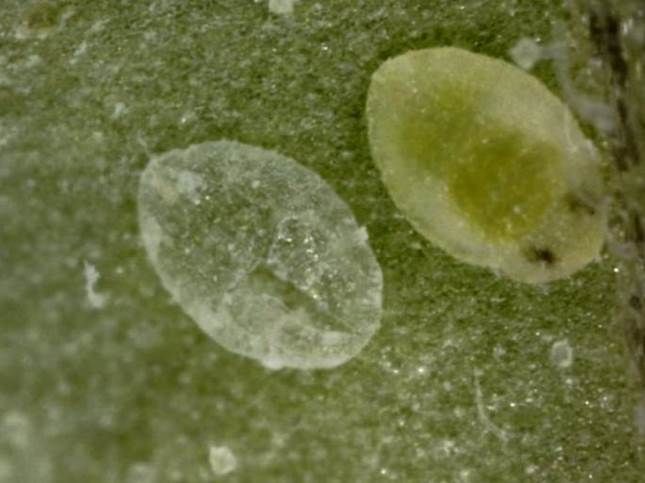
Credit: Lyle Buss UF/IFAS
Damage
Whiteflies have piercing-sucking mouthparts and feed by extracting plant sap from the phloem, which is part of the plant’s vascular system. High numbers of whiteflies (Figure 4) can debilitate a plant by extracting large amounts of sap from the plant. Whiteflies excrete copious honeydew, a sugary substance that accumulates on the leaves below where whiteflies are feeding and serves as a substrate for sooty mold, a type of Ascomycete fungus. Sooty mold can interfere with photosynthesis and make ornamental plants and cotton unmarketable. In addition, the sweetpotato whitefly is responsible for inducing two important plant disorders in Florida crops, silverleafing of squash (Cucurbita pepo) and irregular ripening in tomato, which is caused by nymphal feeding (Schuster 2001) (Figure 5). The greatest economic damage inflicted by sweetpotato whitefly in Florida is due to transmission of plant viruses in the family Geminiviridae: tomato yellow leaf curl virus (TYLCV) in tomato, and three viruses in cucurbits (Adkins et al. 2011).

Credit: Hugh Smith UF/IFAS
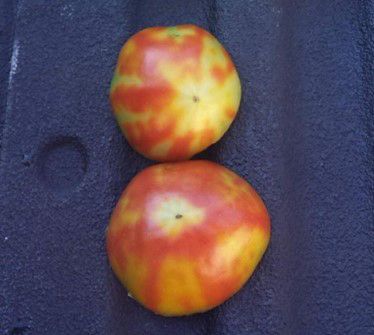
Credit: UF/IFAS
TYLCV
The sweetpotato whitefly transmits TYLCV in a persistent circulative manner, which means that once the whitefly has acquired TYLCV, it retains the virus and can transmit it to new plants for the remainder of its life, although there is evidence that whiteflies become less effective at transmitting TYLCV as they age (Rubenstein and Czosnek 1997). TYLCV produces characteristic symptoms in tomato plants: stunting, reduced leaf size, and bright yellow leaf margins (Polston et al. 1999) (Figure 6). The earlier the tomato crop is infected with TYLCV, the greater the reduction in yield (Levy and Lapidot 2008). Other hosts of TYLCV in Florida include snap beans, peppers (Capsicum annuum), and some weeds (Polston et al. 1999, 2006, Smith et al. 2015). There is no evidence that TYLCV reduces yield in crops other than tomato.

Credit: Hugh Smith UF/IFAS
Management
Clean Culture
Prompt destruction of harvested tomato fields is the most effective way to reduce whitefly numbers and viral inoculum. It is also important to use virus-free transplants.
Host Plant Resistance
There are several varieties of tomato with genetic tolerance to TYLCV. Please refer to “Tomato Production” (https://edis.ifas.ufl.edu/publication/cv137) in the Vegetable Production Handbook of Florida (https://edis.ifas.ufl.edu/publication/CV292) for current information on tomato varieties with tolerance to TYLCV. Varieties of tomato with tolerance to TYLCV can tolerate higher levels of the virus than susceptible varieties and still produce an acceptable yield (Figure 7). Note that these varieties are tolerant to but not resistant to the virus. They can acquire the virus, and if the amount or titer of virus transmitted is high enough, even TYLCV-tolerant tomato varieties will suffer reductions in yield.
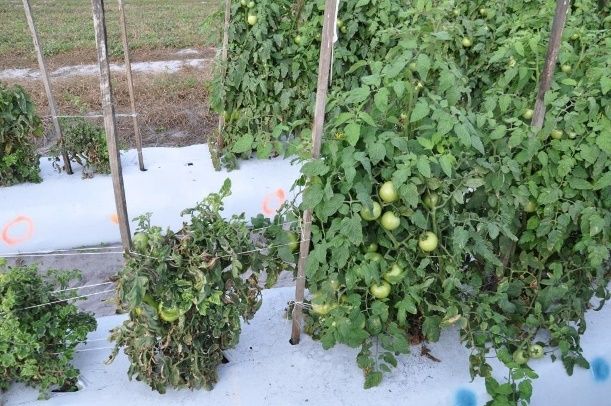
Credit: Hugh Smith UF/IFAS
Repellant Mulches
Metalized plastic mulch can be used to repel adult whiteflies (Figure 8). It is believed that metalized mulches confuse and repel whiteflies by reflecting UV light. As soil accumulates on the metalized mulch and the growing crop overshadows it, the repellant effect is reduced. Some Florida tomato growers have commented that when whitefly numbers are high, metalized mulches, which cost more than black or white plastic mulch, are not effective.
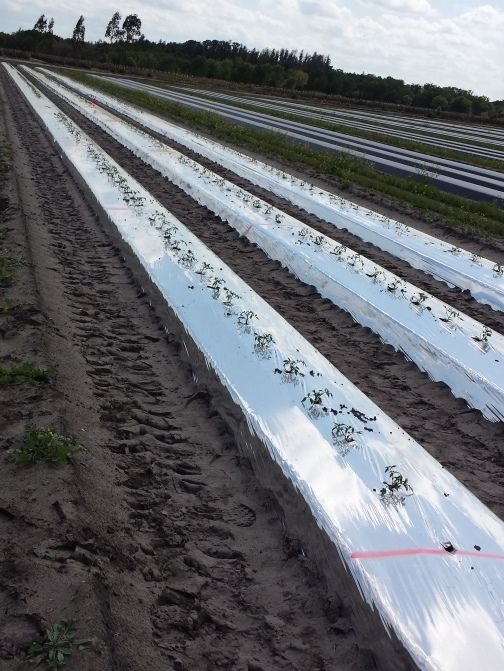
Credit: Hugh Smith UF/IFAS
Natural Enemies
Many types of predatory insects feed on whitefly nymphs, including ladybird beetles (Coleoptera: Coccinellidae), minute pirate bugs (Hemiptera: Anthocoridae) and lacewings (Neuroptera: Chrysopidae). Minute wasps also parasitize the nymphs. These natural enemies are rarely observed on large-scale conventional tomato farms in Florida, presumably because of intensive insecticide use. TYLCV is transmitted by whitefly adults, which have few natural enemies other than fungal diseases that only tend to appear when whitefly populations are dense. Viruliferous whitefly adults can transmit TYLCV to a plant in only a few minutes of feeding (Mehta et al. 1994). Natural enemies have not been found to reduce TYLCV transmission or whitefly numbers under field production conditions, but they may help regulate whitefly populations in unmanaged areas (Smith et al. 2014).
Insecticides
Table 1 lists important insecticide groups registered for use on Florida tomato for management of whiteflies and TYLCV. Consult product labels before use and follow all label directions and restrictions. Among the most important insecticides for whitefly management are those possessing systemic properties, meaning that the insecticide can be taken up through the roots or foliage and distributed throughout the plant via its vascular system. The neonicotinoid insecticides, which include dinotefuran (Venom), imidacloprid (Admire Pro) and thiamethoxam (Actara), are systemic, as is the butenolide insecticide flupyradifurone (Sivanto Prime). These are all categorized as mode of action (MOA) Group 4 insecticides according to the Insecticide Resistance Action Committee (IRAC) (https://irac-online.org/). The mode of action is the mechanism by which the insecticide kills. Group 4 insecticides disrupt nicotinic acetylcholine pathways in the insect. The diamide insecticide cyantraniliprole (Verimark/Exirel) (MOA 28) is also systemic. Diamide insecticides disrupt calcium metabolism in insects. Spirotetramat (Movento MPC) (MOA 23), a lipid biosynthesis inhibitor used to manage whitefly nymphs, has systemic properties.
Some insecticides are translaminar, meaning they will move from the side of the leaf where they are applied to the opposite side of the leaf. This is important for whitefly management as most whitefly life stages are found on the underside of the leaf. Insecticides in the chordotonal modulator group (MOA 9) are translaminar and include afidopyropen (Sefina), pymetrozine (Fulfill), and pyrifluquinazon (PQZ). These insecticides interfere with feeding and movement in insects. Pyriproxifen (Knack) (MOA 7) is an insect growth regulator used to manage whitefly nymphs and has translaminar properties. Many pyrethroid insecticides (MOA 3) work by contact, as does buprofezin (Courier) (MOA 16), used to manage whitefly nymphs. Insects must be directly exposed to contact insecticides, or ingest the insecticide, for the insecticide to work. Systemic insecticides tend to have a longer residual effect than translaminar insecticides, which are usually active for longer than contact insecticides.
Insecticide Resistance
Being effective for weeks rather than days can improve insecticide efficacy against whitefly populations, but the longer whitefly populations are exposed to insecticides in the field, the greater the risk that insecticide resistance will develop. Many factors contribute to the development of insecticide resistance. However, insecticide resistance is most likely to develop when successive generations of a pest population are exposed to the same mode of action (Georghiou 1983). Bemisia tabaci populations have demonstrated the ability to develop resistance to multiple modes of action. In Florida, resistance to imidacloprid and thiamethoxam has been documented in some whitefly populations (Schuster 2009; Smith et al. 2016), and there is evidence that resistance to spirotetramat is present in Florida (Rossitto De Marchi et al. 2021).
Insecticide Resistance Management (IRM)
Rotating modes of action, and only applying insecticides when necessary, are important components of insecticide resistance management. In addition, it can be useful to group modes of action according to the generation time of the target pest to reduce the likelihood that successive generations of the pest are exposed to the same modes of action. Five weeks has been suggested as an insecticide grouping interval for Bemisa tabaci, as this is a reasonable estimate of the time from the egg stage to the death of the adult (please see “Managing Resistance to Diamide Insecticides in Florida Tomato,” https://edis.ifas.ufl.edu/publication/IN978). Insecticides with the same mode of action can be applied more than once within the five-week interval, but in the following five-week interval, and ideally for the remainder of the crop cycle, that mode of action should not be applied again. This is referred to as the treatment interval approach to insecticide resistance management.
Maximum Dose Bioassay
Since 2019, whitefly populations have been collected from commercial vegetable fields in south Florida and maintained at the UF/IFAS Gulf Coast Research and Education Center (GCREC) for insecticide testing. The top labeled rate of key insecticides is tested against whitefly populations in a laboratory bioassay to describe patterns of insecticide susceptibility across south Florida. Figure 9 shows the results of testing twenty whitefly populations from south Florida vegetable fields for susceptibility to bifenthrin (Brigade), cyantraniliprole (Exirel), dinotefuran (Venom), flupyradifurone (Sivanto Prime), imidacloprid (Admire Pro), and thiamethoxam (Actara) (Rossitto De Marchi et al. 2021). Survival tended to be highest when whiteflies were treated with imidacloprid and lowest when treated with dinotefuran and flupyradifurone. Cyantraniliprole and thiamethoxam produced intermediary results. Results from bifenthrin, the one pyrethroid evaluated, were variable. Black horizontal lines below populations on the horizontal access indicate statistical relations among populations. Populations with the same line or overlapping lines are not statistically different in terms of overall survival.
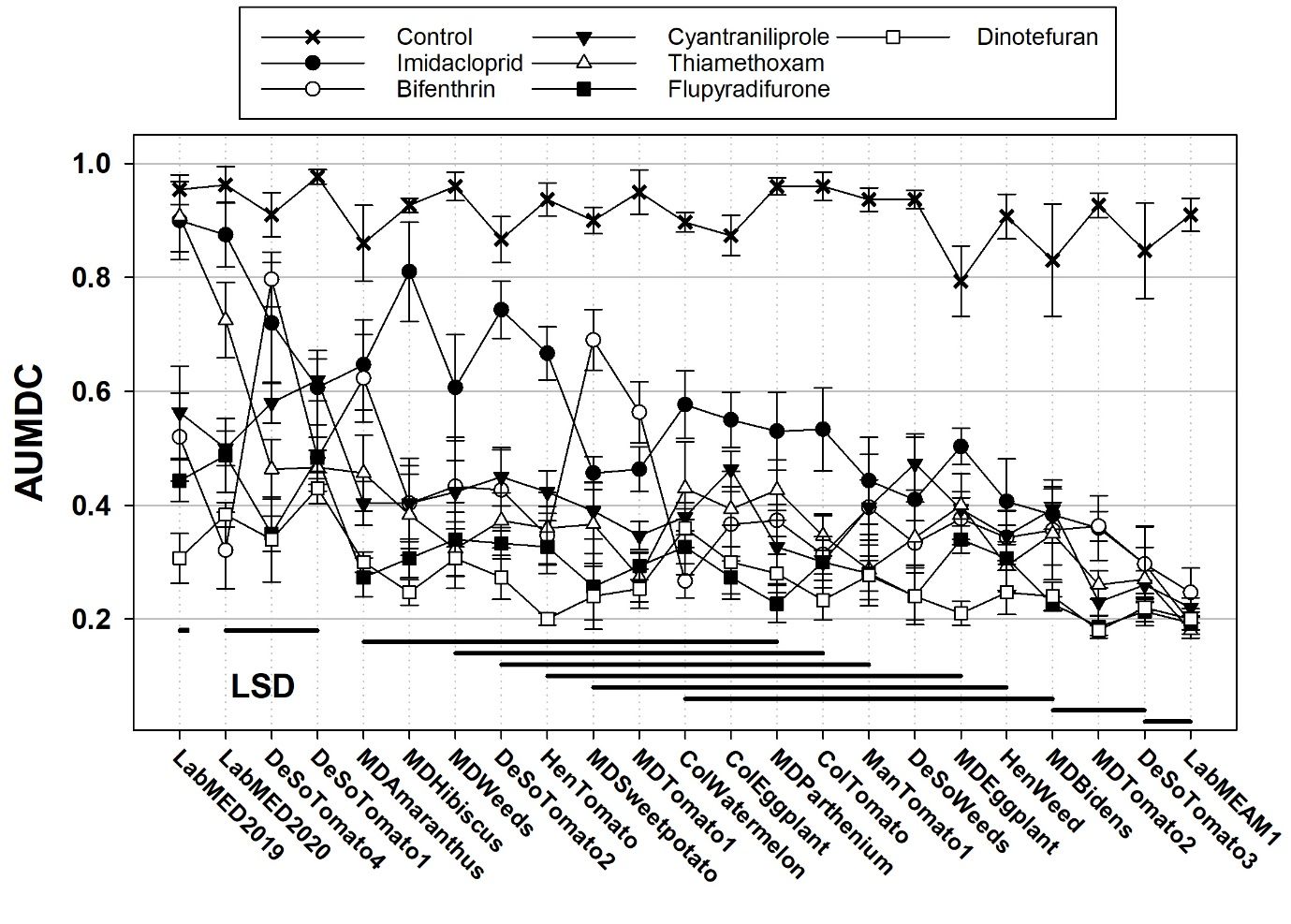
Credit: Adapted from Rossitto De Marchi et al. 2021
Figure 10 shows the results of screening nymphs in some of these populations for susceptibility to buprofezin (Courier), pyriproxifen (Knack) and spirotetramat (Movento) (Rossitto De Marchi and Smith 2021). Specifically, Figure 10 shows the percentage of whitefly nymphs from eleven field populations treated with buprofezin, spirotetramat, and pyriproxifen that completed development to adulthood. Populations were collected from Collier, DeSoto, Hendry, Hillsborough, Manatee and Miami-Dade counties and subjected to a maximum dose test when young nymphs. The maximum dose test uses the top labeled rate of the insecticide. Two weeks after treatment, the percentage of nymphs successfully completing development to the adult stage were recorded. The presence of cast skin (exuviae) is evidence that the nymph completed development and emerged as an adult whitefly. Buprofezin and pyriproxyfen successfully prevented development in all field-collected populations of whitefly, although a low percentage of B. tabaci MED nymphs treated with these insecticides reached adulthood. Spirotetramat (dark grey columns) failed to prevent adult emergence in a significant proportion of several populations, indicating lack of efficacy. The black columns indicate percentage of adult emergence in untreated portions of each population. Results of maximum dose bioassays such as those shown in Figures 9 and 10 are used to develop guidelines for growers regarding the optimal placement of insecticides in season-long rotations for whitefly management.

Credit:
Biopesticides
Biopesticides can be an important component of whitefly management. Biopesticides include insecticidal soaps and oils; botanical insecticides, including azadirachtin; and microbial insecticides. Biopesticides tend to kill in a way that does not select for resistance; therefore biopesticides are usually not assigned an IRAC MOA code. Research at GCREC indicates that insecticidal soap (M-Pede) can provide early-season protection against whiteflies and TYLCV comparable to some conventional insecticides (Figure 11) (Smith 2020). Applications of mineral oil (Suffoil-X) suppressed whitefly nymphs while applications of kaolin clay produced variable results. There is evidence that kaolin clay can increase numbers of whitefly nymphs and incidence of TYLCV (Figure 11) (Propowski and Puterka 2002; Smith 2020).
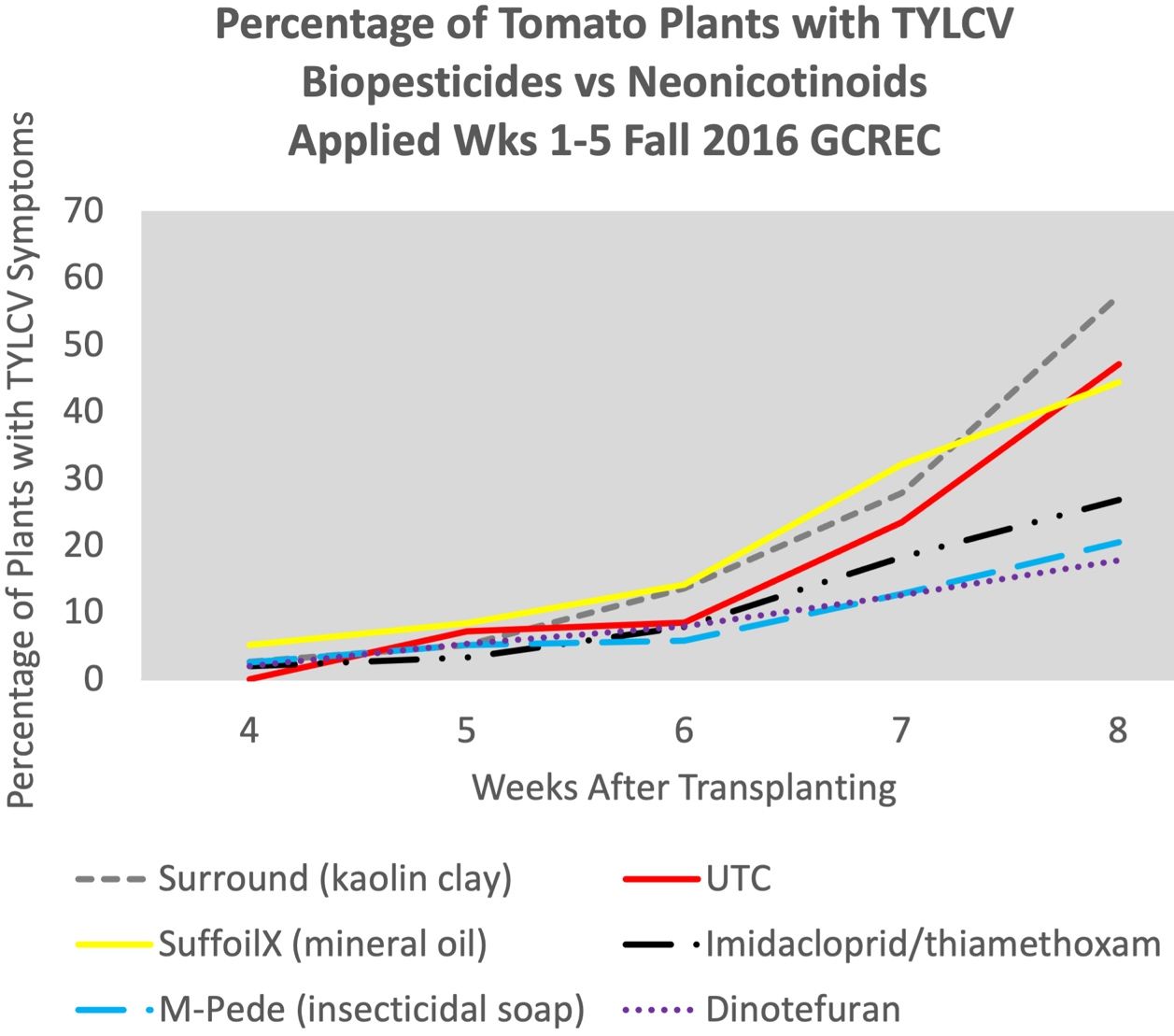
Credit: From Smith 2020, used with permission.
MEAM1 vs MED
Bemisia tabaci MED, also known as the Q biotype whitefly, has demonstrated the ability to develop much higher levels of resistance to some insecticides than B. tabaci MEAM1. In some countries, this has led MED to displace MEAM1 as a pest on both greenhouse and field crops. Bemisia tabaci MED was first detected in the United States in 2004 (Dennehy et al. 2010) and in Florida in 2005 (McKenzie et al. 2009). Bemisia tabaci MED has been established in the Florida landscape since 2016 (McKenzie and Osborne 2017).
Although B. tabaci MED has been detected in many states for several years, it has not yet become established in field agriculture in the United States or caused the level of problems observed in other countries. A Florida population of B. tabaci MED was tested for susceptibility to insecticides using the maximum dose test in 2019 and demonstrated much higher tolerance to imidacloprid and thiamethoxam than field-collected MEAM1 populations (Figure 9). The same population responded differently to whitefly nymph insecticides from MEAM1 populations. Spirotetramat was effective against the MED population, but survival for nymphs treated with buprofezin and pyriproxyfen was slightly higher than for MEAM1 populations Figure 10. In the absence of insecticide applications, MEAM1 tends to outcompete MED in mixed populations. This has been documented in other countries and in Florida (Smith et al. 2020; Rossitto De Marchi et al. 2023). Bemisia tabaci MED has probably not established in high numbers in Florida and other parts of the United States or displaced MEAM1 here, likely because most growers use reasonable approaches to insecticide resistance management and because natural refuges where insecticides are not applied are available.
Summary
Reduction of viral inoculum on a regional level through prompt destruction of harvested fields is essential for management of TYLCV. Metalized plastic mulches can reduce early-season virus transmission. Tomato varieties with genetic tolerance to TYLCV are available for Florida’s distinct production regions. The Group 4 insecticides dinotefuran (Venom) and flupyradifurone (Sivanto Prime) have resulted in high whitefly mortality in tests for several years without evidence of insecticide resistance. The diamide insecticide cyantraniliprole (Exirel, Verimark, MoA 28) is also an important whitefly management tool, although there is evidence of resistance to this material in some whitefly populations in Florida. The Group 9 insecticides afidopyropen and pyrifluquinazon (Sefina and PQZ respectively) have demonstrated efficacy against whitefly infesting Florida crops. The nymph materials buprofezin (Courier) and pyriproxyfen (Knack) prevent immature whiteflies from reaching adulthood. There is evidence in Florida whitely populations of resistance to spirotetramat (Movento MPC), which also targets whitefly nymphs. Biopesticides, including the insecticidal soap M-Pede, can help reduce whitefly numbers and virus transmission. Once an insecticide in a given mode of action has been applied to the tomato crop, its repeated application should be confined to the following five weeks in order to avoid treating successive generations of whiteflies with the same mode of action. This is referred to as the treatment interval approach to insecticide resistance management.
References
Adkins, S. A., C. G. Webster, C. S. Kousik, S. E. Webb, P. D. Roberts, P. A. Stansly, and W. W. Turechek. 2011. “Ecology and Management of Whitefly-Transmitted Viruses of Vegetable Crops in Florida.” Virus Research 159:110–114. https://doi.org/10.1016/j.virusres.2011.04.016
Dennehy, T. J., B. A. Degain, V. S. Harpold, M. Zabornac, S. Morin, J. A. Fabrick, R. I. Nichols, J. K. Brown, F. J. Byrne, and X. Li. 2010. “Extraordinary Resistance to Insecticides Reveals Exotic Q Biotype of Bemisia tabaci in the New World.” Journal of Economic Entomology 103:2174–2186. https://doi.org/10.1603/EC10239
Georghiou, G. P. 1983. “Management of Resistance in Arthropods.” Pest Resistance to Pesticides. Springer US, Boston, MA, pp. 769–792. https://doi.org/10.1007/978-1- 4684-4466-7_32
Levy, D., and M. Lapidot. 2008. “Effect of Plant Age at Inoculation on Expression of Genetic Resistance to Tomato yellow leaf curl virus.” Archives of Virology 153:171–179. https://doi.org/10.1007/s00705-007-1086-y
McKenzie, C. L., G. Hodges, L. S. Osborne, F. J. Byrne, R. G. Shatters, Jr. 2009. “Distribution of Bemisia tabaci Biotypes in Florida—Investigating the Q Invasion.” Journal of Economic Entomology 102:670–676. https://doi.org/10.1603/029.102.0227
McKenzie, C. L., and L. S. Osborne. 2017. “Bemisia tabaci MED (Q biotype) (Hemiptera: Aleyrodidae) in Florida Is on the Move to Residential Landscapes and May Impact Open-Field Agriculture.” Florida Entomologist 100:481–484. https://doi.org/10.1653/024.100.0213
Mehta, P., J. A. Wyman, M. K. Nahkla, and D. P. Maxwell. 1994. “Transmission of tomato yellow leaf curl virus by Bemisia tabaci.” Journal of Economic Entomology 87:1291–1297. https://doi.org/10.1093/jee/87.5.1291
Polston, J. E., R. J. McGovern, and L. G. Brown. 1999. “Introduction of tomato yellow leaf curl virus in Florida and Implications for the Spread of This and Other Geminiviruses of Tomato.” Plant Disease 83:984–988. https://doi.org/10.1094/PDIS.1999.83.11.984
Polston, J. E., L. Cohen, T. A. Sherwood, R. Ben-Joseph, and M. Lapidot. 2006. “Capsicum Species: Symptomless Hosts and Reservoirs of tomato yellow leaf curl virus.” Phytopathology 96:447–452. https://doi.org/10.1094/PHYTO-96-0447
Propawski, T. J., and Puterka G. J. 2002. “Particle Film Applications to Bell Pepper for Whitefly Control.” 1997. Arthropod Management Tests 27:E58. https://doi.org/10.1093/amt/27.1.E58
Rossitto De Marchi, B., and H. Smith. 2021. Efficacy of Buprofezin, Pyriproxyfen and Spitotetramat against Bemisia tabaci MEAM1 Nymphal Populations in Florida.” Crop Protection 149:2021 149. https://doi.org/10.1016/j.cropro.2021.105756
Rossitto De Marchi, B., H. Smith, W. Turechek, and D. Riley. 2021. “A Maximum Dose Bioassay to Assess Efficacy of Key Insecticides against Bemisia tabaci MEAM1 (Hemiptera: Aleyrodidae).” Journal of Economic Entomology 114 (2): 914–921. https://doi.org/10.1093/jee/toab016
Rossitto De Marchi, B., A. Bueno Gama, and H. A. Smith. 2023. “Evidence of the Association between the Q2 Mitochondrial Group of Bemisia tabaci MED Species (Hemiptera: Aleyrodidae) and Low Competitive Displacement Capability.” PLos ONE 18 (1): e0280002. https://doi.org/10.1371/journal.pone.0280002
Rubenstein, G., and H. Czosnek. 1997. “Long-Term Association of tomato yellow leaf curl virus with its Whitefly Vector Bemisia tabaci: Effect on the Insect Transmission Capacity, Longevity and Fecundity.” Journal of General Virology 78:2683–2689. https://doi.org/10.1099/0022-1317-78-10-2683
Salas, J., and O. Mendoza. 1995. “Biology of the Sweetpotato Whitefly (Homoptera: Aleyrodidae) on Tomato.” Florida Entomologist 78:154–160. https://doi.org/10.2307/3495680
Schuster, D. J. 2001. “Relationship of Silverleaf Whitefly Density to Severity of Irregular Ripening of Tomato.” HortScience 36:1089–1090. https://doi.org/10.21273/HORTSCI.36.6.1089
Schuster, D. J., R. S. Mann, M. Toapanta, R. Cordero, S. Thompson, S. Cyman, A. Shurtleff, and R. Morris II. 2009. “Monitoring Neonicotinoid Resistance in Biotype B Bemisia tabaci in Florida.” Pest Management Science. https://doi.org/10.1002/ps.1853
Smith, H. A. 2020. “Biopesticides for Management of Bemisia tabaci MEAM1 (Hemiptera: Aleyrodidae) and tomato yellow leaf curl virus.” Journal of Economic Entomology 113:2310–2318. https://doi.org/10.1093/jee/toaa131
Smith, H. A., D. Shrestha, E. van Santen, Q. Masroor, and A. Wong. 2020. “Development of Bemisia tabaci MEAM1 and MED on Tomato (Solanum lycopersicon) Alone and in a Mixed Population.” Florida Entomologist 103:72–79. https://doi.org/10.1653/024.103.0412
Smith, H. A., C. A. Nagle, and G. A. Evans. 2014. “Densities of Eggs and Nymphs and Percent Parasitism of Bemisia tabaci (Hemiptera: Aleyrodidae) on Common Weeds in West Central Florida.” Insects 5:860–876. https://doi.org/10.3390/insects5040860
Smith, H. A., T. E. Seijo, G. E. Vallad, N. A Peres, and K. L. Druffel. 2015. “Evaluating Weeds as Hosts of Tomato yellow leaf curl virus.” Environmental Entomology 44:1101–1107. https://doi.org/10.1093/ee/nvv095
Smith, H. A., C. A. Nagle, C. A. MacVean, and C. L. McKenzie. 2016. “Susceptibility of Bemisia tabaci MEAM1 (Hemiptera: Aleyrodidae) to Imidacloprid, Thiamethoxam, Dinotefuran and Flupyradifurone in South Florida.” Insects 7:57. https://doi.org/10.3390/insects7040057
Table 1. Key insecticides used for managing Bemisia tabaci and tomato yellow leaf curl virus in Florida tomato. Consult product labels before use and follow all label directions and restrictions. Please contact Hugh Smith for additional information (hughasmith@ufl.edu tel.: 813-419-6588).