This publication is part of a series titled Onsite Sewage Treatment and Disposal Systems, commonly referred to as septic systems. This series is intended to give state and local government officials, soil scientists, consulting engineers, Extension agents, and citizens a basic understanding of onsite wastewater treatment and the behavior of different wastewater-borne contaminants coming from septic systems.
Introduction and Purpose
Viruses are microscopic infectious agents that replicate only inside the living cells of other organisms, although some may remain infectious for significant periods of time outside of the host. More than 100 types of viruses can cause disease in humans, and they can be transmitted in numerous ways. One way viruses enter the human body is through consumption of, or contact with, contaminated food or water. Estimates suggest that 60%–70% of groundwater sources in the United States have been contaminated at some time with viruses and/or bacteria, including those that come from sewage wastes (Macler 1996). If contaminated groundwater is used for drinking purposes without treatment, it then becomes one means of viral transmission in humans. To learn about the behavior of bacteria and protozoa in septic systems, see an accompanying article in this series, "Onsite Sewage Treatment and Disposal Systems: Bacteria and Protozoa" (Lusk et al. 2011) available at https://edis.ifas.ufl.edu/ss552.
Onsite sewage treatment and disposal systems (hereafter referred to as septic systems) can be one source of viral contamination of groundwater, especially if the systems are poorly sited or maintained (Yates 2006). Preventing this means of viral contamination is especially important in Florida because 90% percent of the population obtains their drinking water from groundwater and nearly 3 million septic systems are in use. The objectives of this publication are to (1) characterize the behavior of viruses in septic systems and the soil drain field (also called the soil treatment unit or leach field) and (2) summarize major findings about the extent and character of groundwater contamination with viruses emanating from septic systems.
Viruses in Raw Domestic Wastewater
Studies of viruses in wastewater often evaluate "human enteric viruses," which are causative agents of many human infections, including (1) gastrointestinal infections, (2) hepatitis, (3) respiratory infections, (4) encephalitis, (5) conjunctivitis, and (6) meningitis. Figure 1 shows a rotavirus, which is a human enteric virus that causes severe gastrointestinal infections, especially in young children.
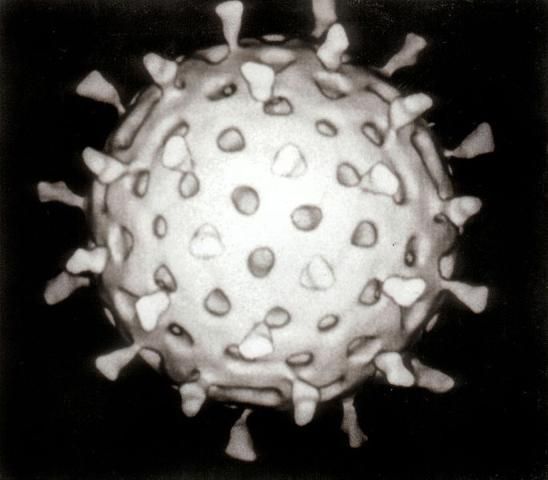
Credit: Wikipedia, http://en.wikipedia.org/wiki/File:Rotavirus_Reconstruction.jpg
Table 1 lists several enteric viruses and the infections they can cause. Enteric viruses usually enter septic systems from human excreta. However, it is important to note that infectious viruses are not part of the normal fecal material; they are present only in the body wastes of an infected individual. Hence, household wastewater will contain varying numbers of viruses and will reflect the combined infection and carrier status of the residents utilizing a septic system. As such, counts of viruses in wastewater can be quite variable. Data are limited on average concentrations of enteric viruses in septic systems wastewater. Canter and Knox (1985) and Charles et al. (2003) estimate that virus concentrations in septic tanks may range from 107 to 1010 virus particles per liter of wastewater during times of infection in a household. Though these high concentrations will be attenuated to a degree by dilution and by soil processes in the drain field, enteric viruses have very low infectious doses in the order of just tens to hundreds of virus particles (Borchardt et al. 2003).
Over 100 types of viruses have been detected in human fecal matter (Gerba and Bitton 1984). Assuming that most individuals have at least one viral infection in a year, it can be estimated that septic systems will receive wastewater containing viruses once a year (Gerba 1984). Anderson et al. (1991) collected about 200 stool samples from children in Florida homes served by septic systems. They identified poliovirus, Echovirus, Coxsackievirus, Enterovirus, and Adenovirus in stool samples from infected children and on several occasions, infected residents were found to be shedding the same virus over a period in excess of 30 days. Human immunodeficiency virus (HIV) has been found in raw household wastewater, but there is no evidence of HIV transmission from septic systems, most likely because HIV has low survivability in the environment. For example, Casson et al. (1992) observed a 2- to 3-log reduction in HIV infectivity just 48 hours after it entered the waste stream. Hepatitis A has been found in wastewater and is of greatest concern because, unlike HIV, it can remain infectious for long periods of time in soil and can cause disease severity (Bitton 1980).
Behavior of Viruses in the Drain Field of Septic Systems
Viruses are notoriously persistent in wastewater and may remain a viable means of infection for months after their entry into the wastewater (Santamaria and Toranzos 2003). In the work by Anderson et al. (1991) in Florida, septic tank effluent sampling from homes where stool samples were positive for viruses demonstrated that the human enteroviruses shed in feces were subsequently discharged from the septic tank for as long as 60 days after the last detectable virus in the stool samples.
The main way septic systems can remove viruses is in the soil drain field. Unlike bacteria, virus particles are not effectively filtered from soil because virus particles are much smaller than bacteria. Virus particles range in size from 20 to 300 nanometer (1 nanometer is one billionth (10-9) of a meter), so they can only be filtered (physically strained) from soils with fine pore sizes (most of which are present in clay soils). Therefore, most viruses are removed in the drain field only by adsorption on soil particles or by inactivation in the soil. While the two processes of adsorption and inactivation of viruses are discussed separately below, it is important to note that they often occur interactively in the soil. Factors that influence virus adsorption and inactivation are summarized in Table 2 and illustrated in Figure 2. Azadpour-Keeley et al. (2003) also provide a comprehensive review of the fate of viruses in the soil.
Adsorption of Viruses in Soils
All viruses have an outer protein coating that encapsulates their DNA or RNA. These protein coats have various electrical charges that promote adsorption to soil particles. As such, several researchers have correlated adsorption of viruses to soil properties such as pH, organic matter content, mineralogy, and texture—all properties that may affect the amount of charge associated with the soil surfaces (Schijven and Hassanizadeh 2002; Jin and Flury 2002). In general, high clay contents, the presence of iron oxide clay minerals, and low pH values have been most positively correlated with virus adsorption (Zhao et al. 2008). See Table 2 for a summary of factors that control virus adsorption in the soil.
Field and laboratory studies indicate that soil particles can adsorb and retain as much as 95% or more of the viruses that percolate through the soil. For example, in a column study by Dowd and Pillai (1997), 79%–100% of viruses were removed from solution. However, these values should be taken with caution, as virus adsorption is variable and may be much lower if environmental conditions are not favorable (see Table 2). For example, Goyal and Gerba (1979) observed virus adsorption rates ranging from 0.01% to 99.9%, pointing out that many investigations of virus adsorption usually focus on only one or a few controlling factors and ignore others that can be of significance in controlling the transport of viruses. These authors also point out that viruses have outer protein coatings that vary by type in their adsorptive characteristics, so adsorption values will depend on the type of virus studied.
Virus Inactivation
Virus inactivation occurs when virus particles are degraded to the point that they are no longer infectious. Factors that affect viral inactivation are summarized in Table 2. Virus inactivation is often most affected by soil water content, with fastest inactivation occurring with decreased water content (Zhao et al. 2008; Blanc and Nasser 1996). Yeager and O'Brien (1979) reported that viruses could persist for at least 180 days in saturated sandy loam or sand soil, whereas no infectivity was recovered from dried soil regardless of soil type. Hurst et al. (1980) found that inactivation rate of poliovirus-1 increased when soil water content of a sandy soil increased from 5% to 15% and then decreased when the water content further increased to 25%.
Note that virus adsorption to soil does not necessarily result in virus inactivation, and adsorption has been shown to be reversible after a change in soil conditions. Hurst et al. (1980) suggested that soils with a high virus adsorption capacity might in fact favor virus survival. If the viruses later desorb from soil surfaces, they may still be infectious. In a study at an in situ septic systems experiment facility in Tampa, Florida, Nicosia et al. (2001) observed virus desorption within the surface 0.6 meters of fine sandy soil under a septic tank effluent infiltration cell during the rainy season, implying that during high rainfall events, viruses once held by soil surfaces may be released and may still be viable agents of infection.
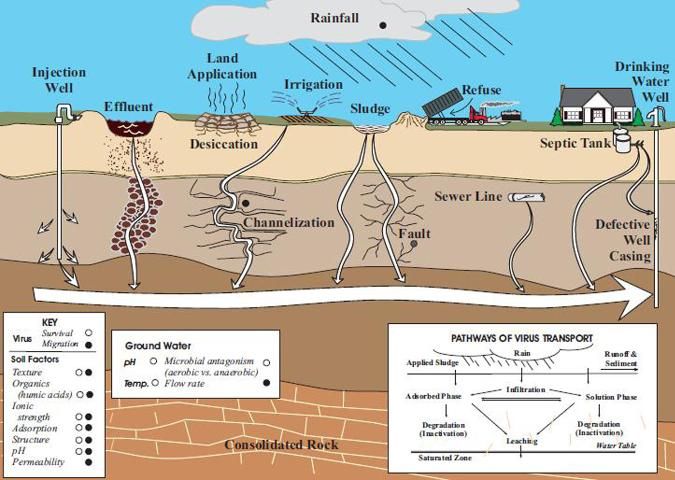
Credit: Azadpour-Keeley et al. (2003).
Viruses and Water Quality
As discussed above and noted in Table 2, many environmental factors affect the migration and survival of viruses in the soil. Thus, viral contamination from septic systems will depend largely on site conditions. A study in Florida where significant numbers of viral agents were identified in stool samples from residents and subsequently in septic tank effluent from the homes found no evidence of active viruses in soil samples taken below the drain field in the soil treatment unit of these same homes (Anderson et al. 1991). Katz et al. (2010) assessed the travel of enteroviruses in parts of northern Florida and found very good attenuation of viruses in the top 5–7 cm of the unsaturated soil zone, except in cases where the depth to fractured limestone bedrock was shallow or where average daily water use was highest. In fact, septic system drain fields most likely do a good job of preventing viral contamination of groundwater, except in some of the most problematic environments. Berger (2008) gives a thorough review of viruses in groundwater and associated disease outbreaks for many regions of the United States. He attributes the most common cause of virus movement into groundwater to sensitive aquifers, which are those that allow rapid transport of contaminants because of certain geologic features. For example, Berger (2008) states that viruses from septic systems are likely to enter groundwater any time one of the following aquifer types is present:
-
alluvial or coastal plain sand aquifers,
-
shallow unconfined aquifers,
-
aquifers with thin or absent soil cover,
-
aquifers in areas with high human population density, and
-
aquifers with restricted geographic extent, such as barrier island sand aquifers.
The U.S. EPA (2006) suggests that the following aquifer types be listed as sensitive to contamination with microscopic pathogens, such as viruses:
-
Limestone aquifers: They are likely to be karst, with numerous sinkholes and springs that facilitate rapid movement of contaminants including viruses.
-
Gravel aquifers: They have large pores that promote rapid water flow and little opportunities for virus removal.
-
Any aquifer known to have fractured rocks: Fractures provide open spaces for rapid flow of contaminants including viruses.
Therefore, conditions that especially encourage virus transport to groundwater include the following:
-
porous soils,
-
fractured bedrock,
-
high septic tank density,
-
karst topography (sinkholes, springs, and caves),
-
shallow water tables, and
-
times of seasonal high rainfall.
There is a research need to establish construction and operating guidelines that will help prevent virus transport from septic systems in the sensitive environments and conditions mentioned above. For example, Katz et al. (2010) suggest that for karst environments or where septic tank density is high we may need to revise current Florida regulations that require only 23 meters of separation between drain fields and potable wells. As an example of how vulnerable environments can be problematic in terms of virus transport from septic systems, Paul et al. (2000) observed that enteric viruses from septic systems could travel up to 3,922 meters in just 27 hours in a Florida Keys coastal environment where soils are very thin over porous limestone at the ground surface and the water table is shallow. Personne et al. (1998) and Futch et al. (2010) add that the frequent appearance of enteric viruses in groundwater off the Florida coast may also be explained by heterogeneity of the limestone bedrock and numerous cracks and fissures. These facilitate rapid flow of groundwater through large pores, leaving little time for virus removal in the drain field.
Futch et al. (2010) investigated the transport of viruses into groundwater and coastal waters in the Florida Keys where limestone exists predominately at ground surface with almost no overlying soil. The study found adenoviruses and enteroviruses present in 8% and 32% of groundwater samples, respectively. They reported septic systems as the most likely cause of these viruses in the groundwater. These same authors found that the viruses remained active in the groundwater and could be transported to coastal waters where they potentially harm sensitive coral reefs in the area. In the Futch et al. (2010) study, virus transport was doubled in the summer, which was attributed to two factors — increased rainfall and higher human population during the tourist season. However, it is important to point out that the area studied by Futch and colleagues likely contained older septic systems that had little or no separation between the drain field and the water table. Current Florida regulations require a 2-foot separation between the drain field and the water table. Most of the septic systems included in the aforementioned study would not meet current rules. Systems constructed since 1984, when the new groundwater separation rule was implemented, are less likely to cause groundwater contamination.
In a study of Florida onsite wastewater systems in sandy soils and water table approximately 1 meter below the drain field, Anderson et. al. (1991) tracked Coxsackievirus A9 from an infected householder's feces, through the septic tank, into the drain field, and subsequently in groundwater samples taken directly below the drain field. At this point, viruses were at very low numbers (0.003 infective units per liter groundwater). However, no virus was detected in groundwater samples 3 meters down gradient of the drain field.
Treatment Alternatives
Several alternative systems (also called performance-based systems) have been developed to potentially reduce virus contamination from septic systems. These systems may include additional sand filters, peat filters, or constructed wetlands that septic effluent passes through, and these filters can serve in addition to or as a replacement for a conventional drain field. These alternative systems can help to overcome remediation deficiencies in the natural soil properties. Olson et al. (2005) compared virus removal for all three of these treatment alternatives. Their study passed septic tank effluent that had been seeded with bacteriophage viruses (a type of virus often used as a proxy in virus studies that infects bacteria and has similar enough properties to human enteric viruses) through a 1.2 meter deep sand filter, a 1.4 meter deep peat filter, or constructed wetlands. These authors found that all systems provided 95% removal of seeded or indigenous viruses in summer and typically 90% removal in winter. They argue that an additional 0.6 m of loamy sand soil from a conventional trench dispersal system would improve these values to 99.5% and 98%, respectively. The peat filter demonstrated the greatest removal by far of viruses in summer and winter, followed by the sand filter, and then the constructed wetland.
Septic systems may also be fitted with chlorine or ultra-violet (UV) radiation disinfection units. These can be built into the septic system during construction, or older systems may be retrofitted. These units typically are implemented so they are one of the last parts of treatment before septic tank effluent passes into the drain field. Chlorine and UV light treatment is especially useful for disinfection in sensitive environments, where the normal soil environment may not be adequate for suitable virus removal (WERF 2009). Both require additional costs for materials and installation ($600–$2,000 per unit), and UV lamps will also require an energy source. WERF (2009) additionally estimates that chlorine systems for individual homes require $70–$200 per year to operate; UV lamps require $190–$280 per year to operate. Also, both systems will require owner maintenance on a regular basis. For example, UV lamps must be replaced once a year.
Summary
Presence of human enteric viruses even in small concentrations in drinking water can cause disease outbreaks. Even low levels of viruses in groundwater have the potential to threaten public health. Viruses have been shown to enter groundwater from septic systems. While some researchers show that viruses are largely attenuated in the soil drain field, others have shown that virus attenuation is variable. Contamination rates can be high if soils are porous or if drain fields overlie fractured bedrock because these conditions provide little opportunities for viruses to adsorb to soil particles. Highly porous soils and rocks also enable rapid transport of virus-laden effluent to groundwater supplies, which reduces the chance that the virus particles will be inactivated before reaching drinking water wells.
Consult the following EDIS articles in this series for more information on these topics:
SS549/SL347 - Onsite Sewage Treatment and Disposal Systems: An Overview
SS550/SL348 - Onsite Sewage Treatment and Disposal Systems: Nitrogen
SS551/SL349 - Onsite Sewage Treatment and Disposal Systems: Phosphorus
SS552/SL351 - Onsite Sewage Treatment and Disposal Systems: Bacteria and Protozoa
SS554/SL353 - Onsite Sewage Treatment and Disposal Systems: Trace Organic Chemicals
References
Anderson, D.L., A.L. Lewis, and K.M. Sherman. 1991. "Human Enterovirus Monitoring at Onsite Sewage Disposal Systems in Florida." In On-site Wastewater Treatment, Vol. 6. ASAE Publ. 10-91, edited by J. Converse, 94-104. St. Joseph, MI: American Society of Agricultural Engineers.
Azadpour-Keeley, A., B.R. Faulkner, and J. Chen. 2003. Movement and Longevity of Viruses in the Subsurface. United States EPA Report EPA/540/S-03/500. Accessed January 2011. http://www.epa.gov/nrmrl/pubs/540S03500/540S03500.pdf.
Berger, P. 2008. "Viruses in Groundwater." In Dangerous Pollutants (Xenobiotics) in Urban Water Cycle, edited by P. Hlavinek, O. Bonacci, J. Marsalek, and I. Mahrikova. Dordrecht, the Netherlands: Springer.
Bitton, G. 1980. Introduction to Environmental Virology. New York: John Wiley & Sons.
Blanc, R., and A. Nasser. 1996. "Effect of Effluent Quality and Temperature on the Persistence of Viruses in Soil." Water Science and Technology 33:237-42.
Borchardt, M.A., P.D. Bertz, S.K. Spencer, and D.A. Battigelli. 2003. "Incidence of Enteric Viruses in Groundwater from Household Wells in Wisconsin." Applied Environmental Microbiology 69:1172-80.
Canter, L.W., and R.C. Knox. 1985. Septic Tank System Effects on Groundwater Quality. Chelsea, MI: Lewis Publishers.
Casson, L.W., C.A. Sorber, R.H. Palmer, A. Enrico, and P. Gupta. 1992. "HIV Survivability in Wastewater." Water Environment Research 64(3):213-15.
Charles, K.J., D.J. Roser, N.J. Ashbolt, D.A. Deere, and R. McGuinness. 2003. "Buffer Distances for On-site Sewage Systems in Sydney's Drinking Water Catchments." Water Science and Technology 47:183-89.
Dowd, S.E., and S.D. Pillai. 1997. "Survival and Transport of Selected Bacterial Pathogens and Indicator Viruses under Sandy Aquifer Conditions." Journal of Environmental Science and Health 32(8):2245-58.
Dowd, S.E., S.D. Pillai, S. Wang, and M.Y. Corapcioglu. 1998. "Delineating the Specific Influence of Virus Isoelectric Point and Size on Virus Adsorption and Transport through Sandy Soils." Applied Environmental Microbiology 64:405-10.
Futch, J.C., D.W. Griffin, and E.K. Lipp. 2010. "Human Enteric Viruses in Groundwater Indicate Offshore Transport of Human Sewage to Coral Reefs of the Upper Florida Keys." Environmental Microbiology 12: 964-74.
Gerba, C.P. 1984. "Applied and Theoretical Aspects of Virus Adsorption to Surfaces." Advances in Applied Microbiology 30:133-68.
Gerba, C.P., and G. Bitton. 1984. "Microbial Pollutants: Their Survival and Transport Patterns to Groundwater." In Groundwater Pollution Microbiology, edited by G. Bitton and C. Gerba. New York: John Wiley & Sons.
Goyal, S.M., and C.P. Gerba. 1979. "Comparative Adsorption of Human Enteroviruses, Simian Rotavirus, and Selected Bacteriophages to Soils." Applied Environmental Microbiology 32(2):241-47.
Hurst, C.J., C.P. Gerba, and I. Cech. 1980. "Effects of Environmental Variables and Soil Characteristics on Virus Survival in Soil." Applied and Environmental Microbiology 40:1067-79.
Jin, Y., and M. Flury. 2002. "Fate and Transport of Viruses in Porous Media." Advances in Agronomy 77:39-102.
Katz, B.G., D.W. Griffin, P.B. McMahon, H.S. Harden, E. Wade, R.W. Hicks, and J.P. Chanton. 2010. "Fate of Effluent-borne Contaminants beneath Septic Tank Drainfields Overlying a Karst Aquifer." Journal of Environmental Quality 39(4):1181-95.
Macler, B. 1996. "Developing the Ground Water Disinfection Rule." Journal of the American Water Works Association 88:47-55.
Nicosia, L.A., J.B. Rose, L. Stark, and M.T. Stewart. 2001. "A Field Study of Virus Removal in Septic Tank Drainfields." Journal of Environmental Quality 30(6):1933-39.
Olson, M.R., R.P. Axler, R.E. Hicks, J.R. Henneck, and B.J. McCarthy. 2005. "Seasonal Virus Removal by Alternative Onsite Wastewater Treatment Systems." Journal of Water and Health 3:139-55.
Paul, J., M. McLaughlin, D. Griffin, E. Lipp, R. Stokes, and J. Rose. 2000. "Rapid Movement of Wastewater from On-site Disposal Systems into Surface Waters in the Lower Florida Keys." Estuaries 23: 662-68.
Personné, J.C., F. Poty, L. Vaute, and C. Drogue. 1998. "Survival, Transport and Dissemination of Escherichia coli and Enterococci in a Fissured Environment. Study of a Flood in a Karstic Aquifer." Journal of Applied Microbiology 84: 431-38.
Redman, J.A., S.B. Grant, T.M. Olson, J.M. Adkins, J.L. Jackson, M.S. Castillo, and W.A. Yanko. 1999. "Physicochemical Mechanisms Responsible for the Filtration and Mobilization of a Filamentous Bacteriophage in Quartz Sand." Water Resources 33:43-52.
Santamaria, J., and G.A. Toranzos. 2003. "Enteric Pathogens and Soil: A Short Review." International Microbiology 6(1):5-9.
Schijven, J.F., and S.M. Hassanizadeh. 2002. "Removal of Viruses by Soil Passage: Overview of Modeling, Processes, and Parameters." Critical Reviews in Environmental Science and Technology 30:49-127.
US EPA (United States Environmental Protection Agency). 2006. National Primary Drinking Water Regulations: Ground Water Rule. Federal Register 71(224):67427-65660.
WERF (Water Environment Research Foundation). 2009. "Performance and Cost of Decentralized Unit Processes." Fact Sheet T7. Accessed October 6, 2011. http://www.werf.org/AM/Template.cfm?Section=Decentralized_Systems&Template=/CM/ContentDisplay.cfm&ContentID=15569.
Yates, M. V. 2006. "Septic Tank Density and Groundwater Contamination." Ground Water 23(5):586-91.
Yeager, J.G., and R.T. O'Brien. 1979. "Enterovirus Inactivation in Soil." Applied and Environmental Microbiology 38:694-701.
Zhao, B., H. Zhang, J. Zhang, and Y. Jin. 2008. "Virus Adsorption and Inactivation in Soil as Influenced by Autochthonous Microorganisms and Water Content." Soil Biology and Biochemistry 40:649-59.