Purpose and Target Audience
Microorganisms that reside very close to the root system (known as the rhizosphere) play a pivotal role in regulating plant and soil health. By harnessing the activities of these beneficial microbes, it becomes possible to facilitate plant growth, streamline plant nutrient uptake, and ultimately enhance crop yields and quality. Within the rhizosphere, these microbes also contribute to diminishing the reliance on synthetic fertilizers and pesticides by promoting natural plant-microbe-soil interactions, such as nutrient cycling and disease resistance. Furthermore, these rhizosphere microbes actively contribute to the preservation of soil structure and nutrients, thereby mitigating soil degradation, erosion, and nutrient leaching. The newly acquired insights pertaining to rhizosphere microbes stand as an initial guiding concept, enabling growers to fine-tune their agriculture practices toward the goal of sustainable farming. These insights are tailored for a diverse audience including the growers, producers, and the wider public interest in fostering environmental awareness.
Introduction: The soil is alive!
A gram of soil can harbor 100 million to a billion microorganisms, meaning every inch of our soil is alive (Raynaud and Nunan 2014) (Figure 1). Most efforts of microbial management to improve crop production focus on alleviating negative interactions between crops and pathogens. However, most soil microbes are good, and many of them play important roles in improving soil health by storing and releasing nutrients.
In addition, many microbes that live near plant roots can help plants access nutrients and fight pathogens. Together, these efforts can assist crop production. While it is recognized that many soil microbes perform key roles in crop productivity, the importance of these underground activities is easily overlooked because of their small size. By focusing on a specific group of microbes living on or near plant roots, this publication provides understanding for these questions: Who are these microbes and how do they improve plant and soil health?
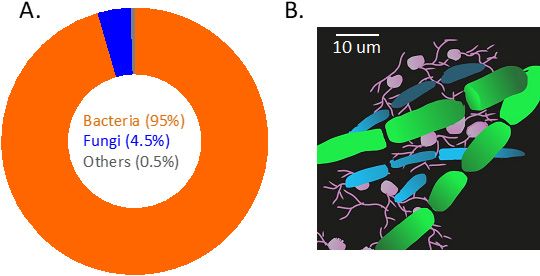
Credit: Hui-Ling Liao, UF/IFAS
Activity of Soil Microbes in the Rhizosphere
The “rhizosphere” is defined as the area of soil surrounding the root system. It is a specific region directly influenced by the communication between the microbes and their plants. Except plant pathogens, many microbes in the rhizosphere are considered beneficial to the plants and soil, including endophytes, mycorrhizal fungi, nitrogen-fixers, and saprophytes (Figure 2). We will discuss each group of these microbes in the proceeding sections. All of these microbes together with plant feeders (plant pathogens) are considered plant-associated microbes. To establish and defend their territory, the good rhizosphere microbes form a protective barrier called “rhizosphere microbiome,” covering the root surfaces. In this zone, the beneficial microbes can prevent root infections by reducing access and providing nutrients (e.g., nitrogen, phosphorous, and micronutrients) to the plants.
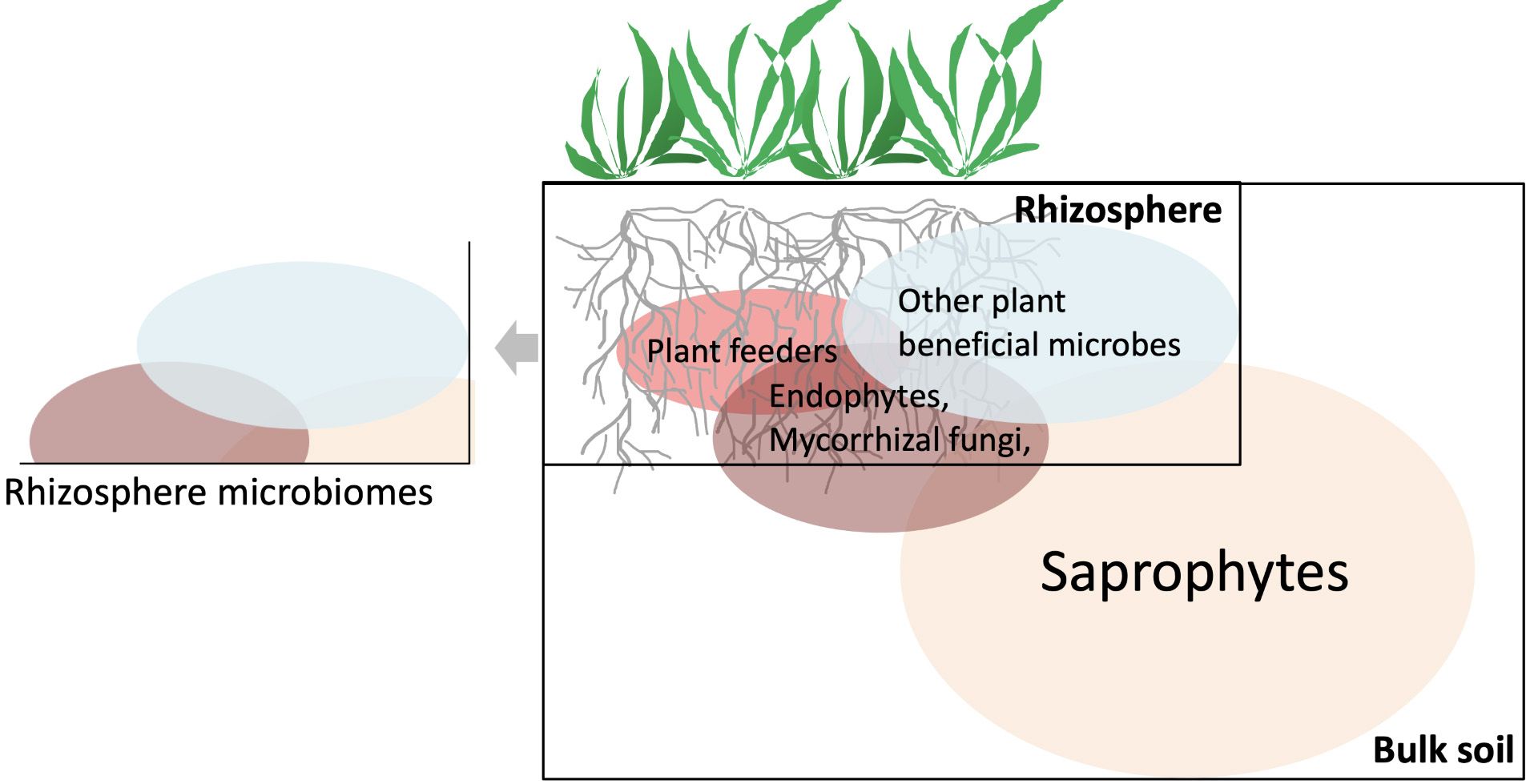
Credit: Hui-Ling Liao, UF/IFAS
The rhizosphere harbors relatively lower diversity (i.e., fewer different types of microbes) but a greater total number of soil microbial organisms (1010-1011 cells per gram rhizosphere soil) compared to the rest of the soil (i.e., the bulk soil). This lower diversity but greater abundance in the rhizosphere likely occurs because plants recruit only a specific subset of soil microbes to the rhizosphere (Yin et al. 2021). Plant roots can influence which soil microbes live in the rhizosphere by secreting specific root exudates and volatiles, a complex mixture of low-molecular weight compounds. These root exudates include sugars, amino acids, organic acids, and other small molecules that can feed the microbes in the rhizosphere. It is estimated that nearly 10-35% of the carbon from plants is released as exudates to the rhizosphere and used by microbes (Kuzyakov 2002).
Rhizosphere microbes are critical for nutrient availability and plant pathogen defense.
Rhizosphere microbes govern many soil ecosystem processes, including soil formation (e.g., nutrient cycling, aggregation), crop nutrient acquisition, and plant disease resistance. Rhizosphere microbes are essential for soil formation.
Soil microbes, including the rhizosphere microbes, are critical to soil formation because soil microbes are responsible for processing organic matter (Figure 3). For example, plant symbionts and other rhizosphere microbes (e.g., saprophytes living in the rhizosphere) can obtain plant-synthesized carbon from plant roots or through the carbon-associated compounds released from plant roots or plant litter (rhizodeposition) (Zhang, Maltais-Landry, and Liao 2021). These microbes also process the nutrients from plant litter through decomposition. Some of these processes may reduce or raise the production of nitrogen and carbon gas through respiration. Overall, these microbial-mediated processes are critical to balance the storage and use of soil carbon. Of the nearly 2,500-gigatonne (GT) carbon in Earth’s soil, it is estimated that more than 2000 GT are regulated by soil biota and 80% of soil biota-mediated carbon are regulated by soil microbes (Gougoulias, Clark, and Shaw 2014).
Soil aggregation is a good example showing that rhizosphere microbes enhance soil formation. A soil aggregate is the basic unit of soil structure and comprises of microbes, other biota, organic matter, and pores. Aggregates hold soil water, store soil organic matter, regulate aeration, and host microbes. Aggregate formation and stability control seed germination and root growth. The plant roots and soil biota (including rhizosphere microbes) contribute to aggregate formation and stability. Some rhizosphere microbes can produce exopolysaccharides, which are polysaccharides secreted by microbes into the soil, to facilitate aggregate formation (Lynch and Bragg 1985; Alami et al. 2000).
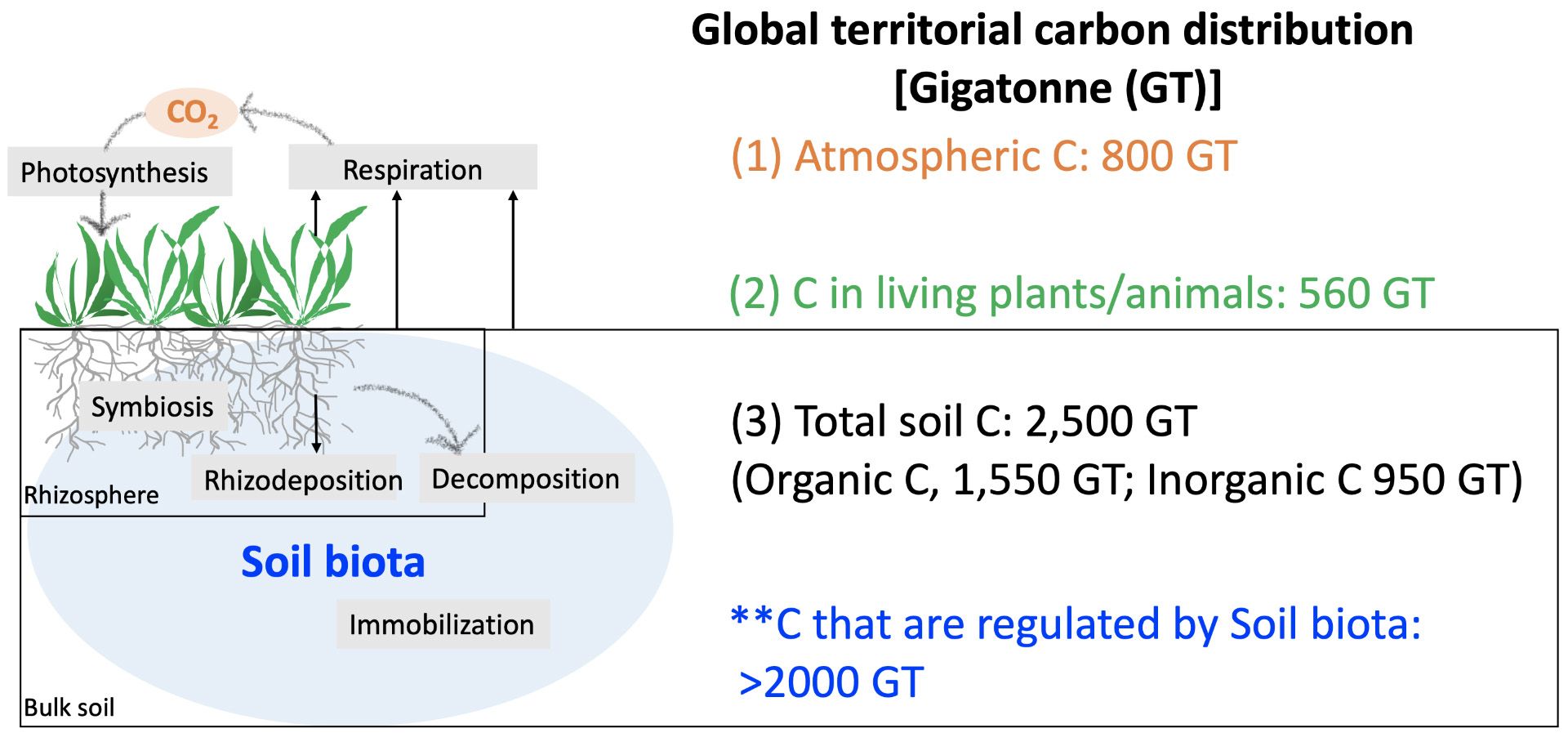
Credit: Hui-Ling Liao, UF/IFAS
Crop Nutrient Acquisition Mediated by Rhizosphere Microbes, Including Free-living Saprophytes and Plant Symbionts
The strategies that the rhizosphere microbes utilize to facilitate plant nutrient acquisitions largely depend on their association with soil and plants. Two major types of good rhizosphere microbes are (free-living) saprophytes and symbionts (Figure 2). Some microbes can perform dual lifestyle, meaning that the same taxon can live in rhizosphere as saprophytes and plant symbionts. Microbial saprophytes include a group of microorganisms that feed on organic matter from dead organisms. They are the major microbial group that contributes to soil decomposition and soil nutrient cycling. These saprophytes mainly convert large organic compounds into smaller, simple molecules. The nutrients processed by these saprophytes, especially nitrogen, phosphorus, and micronutrients, can become accessible to plant symbionts and plants. In general, the dominant saprophytes presenting in tropical and sub-tropical agriculture soil include the genera of Bacillus, Fusarium, Penicillium, Trichoderma, Mortierella, etc.
Symbiosis occurs when two or more unlike organisms live and interact with each other (de Bary 1879). Many rhizosphere microbes can build symbiotic relationships with plants (“microbial symbionts”) that are fundamental to sustainable plant growth and crop productivity. These microbes provide plant hosts with essential nutrients— including nitrogen, phosphorous, and water— in exchange for plant-fixed carbon. Rhizosphere microbial symbionts include arbuscular mycorrhizal fungi (AMF), ectomycorrhizal fungi (EMF), nitrogen-fixing bacteria, and endophytic fungi/bacteria (Figure 2).
Mycorrhizal fungi are well-known plant symbionts that establish partnerships with plants by sharing essential and often limiting nutrients between roots and fungi. For example, AMF are ubiquitous, obligate symbiotic fungi from the phylum Glomeromycota. AMF live in the roots of more than 80% of land plants, including wild grasses, forest trees, and many agricultural crops. Nearly 2% of terrestrial plant species host EMF, and most are tree species. AMF penetrate the cells within the roots and form fungal structures (called arbuscules) for nutrient exchange. The intracellular fungal hyphae (i.e., thread-like fungal structure) of AMF are enveloped by the perifungal membrane, which is an extension structure of the host cell plasma membrane (Figure 4). An apoplastic space in between the fungi and the plant cell serves as a plant-fungal interface to mediate nutrient exchange (Fungus-to-plant cell and Plant cell-to-fungus transfer interface). As shown in Figure 4, we can imagine that fungal hyphae can form a cauliflower-like structure (arbuscule) and develop this structure into part of the plant cell membrane (but does not destroy any plant membrane), creating a larger surface area interface between the fungi and plant for nutrient exchange.
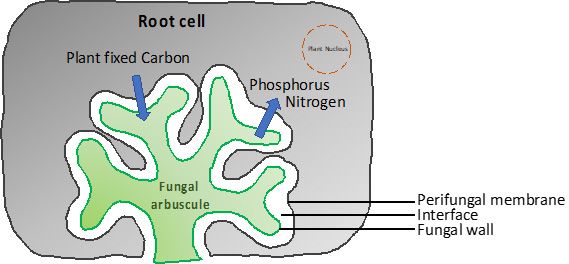
Credit: Hui-Ling Liao, UF/IFAS
Unlike AMF, EMF live within the root (intercellularly, i.e., between two plant cells) but do not penetrate the root cells; therefore, they can still perform the exchange of the nutrients between fungi and plants. Although AMF and EMF may prefer interacting with different types of plants, these mycorrhizal fungi can provide similar benefits to their host plant. Through interacting with plant roots and other microbes in the rhizosphere, mycorrhizal fungi facilitate plant nutrient access and stress tolerance. For example, AMF enhance plant tolerance against heat, drought, salinity, and toxic metals. In addition to living in the root tissues, mycorrhizal fungi form hyphae, expanding into the rhizosphere. These extensive hyphae can establish intensive networks (Figure 5). The hyphal networks serve as highways to transport nutrients from the soil-to-soil organisms, including neighboring plant species (Liao et al. 2021; Cahanovitc et al. 2022).
Another well-known plant symbiont are the nitrogen-fixing bacteria called rhizobia, which can form nodules on the roots of legumes (e.g., peas, peanuts, beans). These bacteria can turn nitrogen into a form plants can use. Endophytes are another group of microorganisms (including fungi and bacteria) that live in plant tissues without causing any apparent harm to plants. Many endophytes benefit plant health. For example, some endophytes can produce secondary metabolites (e.g., alkaloids, phenolic acids, terpenoids, quinones, steroids, etc.) to repel pathogens and insects. Some endophytes promote plant growth through regulating plant hormones and defense responses (e.g., Mortierella elongata) (Liao et al. 2019; Zhang et al. 2020). Some endophytes can transfer nutrients (e.g., nitrogen) to their plant hosts. (e.g., Metarhizium and Beauveria) (Behie and Bidochka 2014). Some (but not all) endophytes may have dual lifestyle since they can live in plants but can also survive independently without plants. These endophytes can be considered as rhizosphere microbes. For example, the endophyte (Mortierella sp.) can serve as a saprophyte in rhizosphere to decompose nutrients for the plant to use (Liao et al. 2019; Liao 2021). More studies on endophyte-plant interactions will help us to learn more about these hidden microbes.
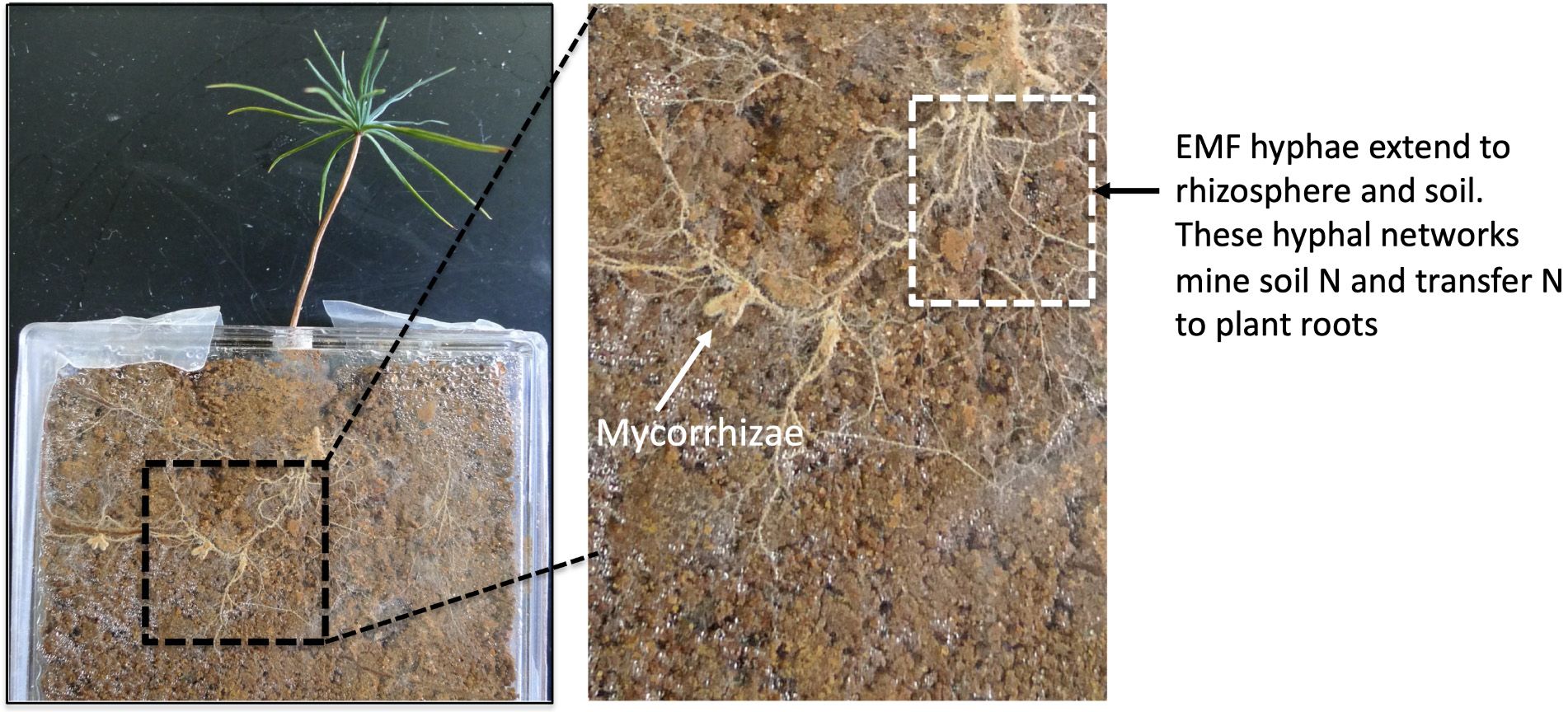
Credit: Hui-Ling Liao, UF/IFAS
Rhizosphere microbes help plant resistance to diseases.
Rhizosphere microbes can repel or out compete emerging plant pathogens near roots (Li et al. 2021). There are several strategies that rhizosphere microbes use to directly protect plants against pathogens. These include: (1) suppressing the pathogenicity of the pathogens or forming a physical barrier against pathogens (antagonism), (2) enhancing plant nutrient access and tolerance to pathogens, and (3) occupying the habitat that supplies root-released nutrients and leaving less space or resources for pathogens (niches and resource competition). For example, many EMF form a sheath using their hyphae that covers the entire root tip (Fig. 2) to prevent pathogens from directly accessing and feeding on roots. Mycorrhizal fungi and nitrogen-fixing microbes benefit plants by providing plant nutrients and subsequently enhancing plant health against pathogens as well as other stresses. Some microbes in the rhizosphere can accelerate the immune system of plants by inducing plant systemic resistance pathways. The plant compounds produced by these pathways (e.g., induced systemic resistant pathways [ISR]) allow plants to block pathogen penetration or colonization (Berendsen, Pieterse, and Bakker 2012). Some rhizosphere microbiomes, including mycorrhizal fungi, can acidify soil to stress pathogens (Tedersoo, Bahram, and Zobel 2020). For example, beneficial bacteria such as those of the family Xanthomonadaceae and Comamonadaceae produce organic acids to suppress Fusarium wilt disease (Wen et al. 2020).
Rhizosphere microbes help alleviate plant stress.
In addition to pathogen attacks, plants can also encounter environmental stresses such as drought, heat, cold, salinity, and soil pollution (e.g., heavy metals). Rhizosphere microbes can play a critical role in helping plants alleviate these “abiotic” stresses. For example, while colonizing plant roots, part of AMF hyphae can grow into soil pores to transport water to the plant roots. Such efforts help increase productivity of a crop under drought conditions (Augé, Toler, and Saxton 2015). Various research findings have supported these benefits and more. Some endophytes, such as the heat-tolerant isolate of Bacillus cereus, mitigated the damage of soybean plants from heat stress by re-balancing plant hormones (e.g., abscisic acid, salicylic acid, and auxin) (Khan et al. 2020). Some other specific bacteria, such as Pseudomonas AK-1 and Bacillus SJ-4, helped soybeans tolerate salinity by manipulating the specific amino acid accumulation (proline) and lipoxygenase activation in plants (Kumari et al. 2015). While colonizing plant roots in soils with high levels of zinc, the zinc-tolerant EMF species (Suillus luteus) retained zinc in the fungal sheath and prevented high amounts of zinc from reaching the plant xylem tissues (Zhang et al. 2021).
Conclusion
Plant beneficial microbes are found within the rhizosphere, roots, and root surfaces. Many of these rhizosphere microbes can communicate with plants, and some form mutually beneficial relationships with plants. These rhizosphere microbes can improve plant health and soil nutrient cycling by regulating root exudation, alleviating plant stresses, and influencing other soil biota. The abundance and type of rhizosphere microbes can be influenced by any of these diverse soil conditions: temperature, moisture, and other organisms (e.g., plant species and other biota) as well as the presence of root and soil chemicals, atmospheric gases, crop species, and applied management practices. Learning how the rhizosphere can improve crop production and regulate soil quality, as well as the interactions between management practices and environmental variables, will help identify the next generation of management systems for sustainable agriculture.
References
Augé, R. M., H. D. Toler, and A. M. Saxton. 2015. “Arbuscular Mycorrhizal Symbiosis Alters Stomatal Conductance of Host Plants More under Drought Than under Amply Watered Conditions: A Meta-Analysis.” Mycorrhiza. 25: 13-24. https://doi.org/10.1007/s00572-014-0585-4
Alami, Y., W. Achouak, C. Marol, and T. Heulin. 2000. “Rhizosphere Soil Aggregation and Plant Growth Promotion of Sunflowers by an Exopolysaccharide-Producing Rhizobiumsp. Strain Isolated from Sunflower Roots.” Applied and Environmental Microbiology. 66(8): 3393-3398. https://doi.org/10.1128/AEM.66.8.3393-3398.2000
Behie, S. W., and M. J. Bidochka. 2014. “Nutrient Transfer in Plant-Fungal Symbioses.” Trends in Plant Science. 19(11): 734-740. https://doi.org/10.1016/j.tplants.2014.06.007
Berendsen, R. L., C. M. J. Pieterse, and P. A. H. M. Bakker. 2012. “The Rhizosphere and Plant Health.” Trends in Plant Science. 17(8): 478-486. https://doi.org/10.1016/j.tplants.2012.04.001
Cahanovitc, R., S. Livne-Luzon, R. Angel, and T. Klein. 2022. “Ectomycorrhizal Fungi Mediate Belowground Carbon transfer Between Pines and Oaks.” ISME Journal. 16: 1420-1429. https://doi.org/10.1038/s41396-022-01193-z
de Bary, A. 1879. Die Erscheinung der Symbiose. Strassburg, Germany: Verlag von Karl J.Trubner. https://doi.org/10.1515/9783111471839
Gougoulias, C., J. M. Clark, and L. J. Shaw. 2014. “The Role of Soil Microbes in the Global Carbon Cycle: Tracking the Below-ground Microbial Processing of Plant-derived Carbon for Manipulating Carbon Dynamics in Agricultural Systems.” Journal of Science and Food Agriculture. 94(12): 2362-2371. https://doi.org/10.1002/jsfa.6577
Khan, M. A., S. Asaf, A. L. Khan, R. Jan, S. -M. Kang, K. -M. Kim, and I. -J. Lee. 2020. “Thermotolerance Effect of Plant Growth-promoting Bacillus cereus SA1 on Soybean During Heat Stress.” BMC Microbiology. 20: 175. https://doi.org/10.1186/s12866-020-01822-7
Kumari, S., A. Vaishnav, S. Jain, A. Varma, and D. K. Choudhary. 2015. “Bacterial-mediated Induction of Systemic Tolerance to Salinity with Expression of Stress Alleviating Enzymes in Soybean (Glycine max L. Merrill).” Journal of Plant Growth Regulation. 34: 558-573. https://doi.org/10.1007/s00344-015-9490-0
Kuzyakov, Y. 2002. “Review: Factors Affecting Rhizosphere Priming Effects.” Journal of Plant Nutrition and Soil Science. 165(4): 382-396. https://doi.org/10.1002/1522-2624(200208)165:4%3C382::AID-JPLN382%3E3.0.CO;2-%23
Li, J., C. Wang, W. Liang, and S. Liu. 2021. “Rhizosphere Microbiome: The Emerging Barrier in Plant-Pathogen Interactions.” Frontiers in Microbiology. 12: 772420. https://doi.org/10.3389/fmicb.2021.772420
Liao H. -L. 2021. “The Plant-Growth Promoting Fungus, Mortierella elongata: Its Biology, Ecological Distribution and Growth-Promoting Activities: SS679/SL466, 3/2021.” EDIS 2021 (2). https://doi.org/10.32473/edis-ss679-2021
Liao, H. -L., G. Bonito, K. Hameed, S. H. Wu, K. -H. Chen, J. Labbé, C. W. Schadt, et al. 2021. “Heterospecific Neighbor Plants Impact Root Microbiome Diversity and Molecular Function of Root Fungi.” Frontiers in Microbiology. 12: 680267. https://doi.org/10.3389/fmicb.2021.680267
Liao, H. -L., G. Bonito, J. A. Rojas, K. Hameed, S. Wu, C. W. Schadt, J. Labbé, et al. 2019. “Fungal Endophytes of Populus trichocarpa Alter Host Phenotype, Gene Expression, and Rhizobiome Composition.” International Society for Molecular Plant-Microbe Interactions. 32(7): 853-864. https://doi.org/10.1094/MPMI-05-18-0133-R
Lynch, J. M., and E. Bragg. 1985. “Microorganisms and Soil Aggregate Stability.” In Advances in Soil Science, vol. 2, edited by B. A. Stewart. New York: Springer. 133-171. https://doi.org/10.1007/978-1-4612-5088-3_3
Raynaud, X., and N. Nunan. 2014. “Spatial Ecology of Bacteria at the Microscale in Soil.” PLoS One. 9(1): e87217. https://doi.org/10.1371/journal.pone.0087217
Tedersoo, L., M. Bahram, and M. Zobel. 2020. “How Mycorrhizal Associations Drive Population and Community Biology.” Science. 367(6480): eaba1223. https://doi.org/10.1126/science.aba1223
Wen, T., J. Yuan, X. He, Y. Lin, Q. Huang, and Q. Shen. 2020. “Enrichment of Beneficial Cucumber Rhizosphere Microbes Mediated by Organic Acid Secretion.” Horticulture Research. 7: 154. https://doi.org/10.1038/s41438-020-00380-3
Yin, C., J. M. Casa Vargas, D. C. Schlatter, C. H. Hagerty, S. H. Hulbert, and T. C. Paulitz. 2021. “Rhizosphere Community Selection Reveals Bacteria Associated with Reduced Root Disease.” Microbiome. 9: 86. https://doi.org/10.1186/s40168-020-00997-5
Zhang, K., G. Bonito, C. -M. Hsu, K. Hameed, R. Vilgalys, and H. -L. Liao. 2020. “Mortierella elongata Increases Plant Biomass among Non-Leguminous Crop Species.” Agronomy. 10(5): 754. https://doi.org/10.3390/agronomy10050754
Zhang, K., G. Maltais-Landry, and H. -L. Liao. 2021. “How Soil Biota Regulate C Cycling and Soil C Pools in Diversified Crop Rotations.” Soil Biology and Biochemistry. 156: 108219. https://doi.org/10.1016/j.soilbio.2021.108219
Zhang, K., R. Tappero, J. Ruytinx, S. Branco, and H. -L. Liao. 2021. “Disentangling the Role of Ectomycorrhizal Fungi in Plant Nutrient Acquisition along a Zn Gradient Using X-ray Imaging.” Science of the Total Environment. 801: 149481. https://doi.org/10.1016/j.scitotenv.2021.149481