Summary
Environmental education improves understanding of complex environmental problems, such as climate change, and benefits student learning. Ocean acidification, the lowering of seawater pH due to increased concentrations of carbon dioxide in the atmosphere, is an emerging environmental challenge associated with climate change. We designed a lesson plan for Florida grade eight educators to reinforce fundamental concepts in acid-base chemistry, including the pH scale, and to introduce students to waterbody acidification, its negative effects on shell-forming organisms, and the potential role of aquatic plant metabolism (i.e., photosynthesis and respiration) in mitigating these effects. In the three-part lesson plan, students will 1) identify household solutions based on pH measurements to become more familiar with the pH scale, 2) observe the effects of acidification (carbonation) on pH and on analogs for shell-forming organisms, and 3) test the effects of aquatic plant photosynthesis and respiration on pH. The goal of the lesson plan is to encourage students to link water chemistry and biological processes while learning about the challenges and potential solutions to acidification within a local context. This lesson plan will contribute to state learning standards while generating appreciation for the complexity of the natural environment.
Introduction
Humans impact the environment in many ways, especially through generating greenhouse gas emissions, which have led to climate change and associated changes in seawater chemistry, including ocean acidification. Ocean acidification refers to lower pH levels that result from higher amounts of carbon dioxide (CO2) in seawater. Water with a lower pH is more acidic and can cause negative impacts to marine life. This relates to fundamental concepts in chemistry, like the properties of acids and bases and the pH scale. Teaching about the environment helps people understand complex environmental problems and empowers them to take action to address local issues (Ardoin, Bowers, and Gaillard 2020). It can also improve students' environmental knowledge and attitudes (van de Wetering et al. 2022). We provide a lesson plan for teaching students about the links between water chemistry and biological processes, as well as the challenges and potential solutions to waterbody acidification within a local context.
The pH scale describes the acid-base properties of solutions. Specifically, pH is the inverse measure of hydrogen ion (H+) activity in solution. Low values on the pH scale indicate high H+ activity, meaning the solution is an acid. High values on the pH scale indicate low H+ activity, meaning the solution is a base. When CO2 dissolves in water, it reacts with water to release H+ ions, making the water more acidic. This process is called acidification. Acidification can negatively affect biological processes. For example, pH controls the behavior of heavy metals like aluminum in forest soils. Acid rain can lead to soil acidification, releasing soil-bound aluminum, which can damage plants and harm animals. The concept of pH is central to understanding how environmental issues such as acid rain and waterbody acidification impact biological processes.
Both marine and freshwater systems can become acidic, but through different mechanisms. For example, industrial pollution in the 1970’s led to high levels of sulfate deposition as precipitation (a form of acid rain) in the northeastern United States. This caused widespread acidification of lakes and rivers, which damaged these ecosystems and killed fish (Likens 1976). There is also some evidence that rising levels of CO2 in the atmosphere can indirectly cause freshwater acidification. For example, high atmospheric CO2 levels can enhance vegetation growth along lake shorelines and lead to higher decomposition rates when dead plant material accumulates in lakes. Decomposition produces CO2, which can make the water column more acidic (lower pH), potentially harming invertebrates (Weiss et al. 2018).
Ocean acidification, the most studied form of waterbody acidification, is the increase in seawater CO2 and reduction in pH. Increased CO2 levels in the atmosphere are a direct cause, effectively making seawater better at breaking down the shells of certain organisms (Figure 1; Doney et al. 2009). In simple terms, CO2 diffuses into the ocean where it interacts with a mixture of compounds, the carbonate system, that control seawater pH. If enough CO2 diffuses into the ocean, it can shift the balance of the carbonate system. The carbonate system protects seawater from changes in pH. Currently, and under various future climate change scenarios, the ocean has absorbed more CO2 than the carbonate system can handle and still maintain pH stability. The ability of seawater to soak up CO2 before changing pH is known as buffering capacity. Increased CO2 in seawater causes a shift toward producing more bicarbonate (HCO3-) ions, fewer carbonate (CO32-) ions needed to build shells, and too many hydrogen (H+) ions. All of these changes make the water more acidic with a lower pH.
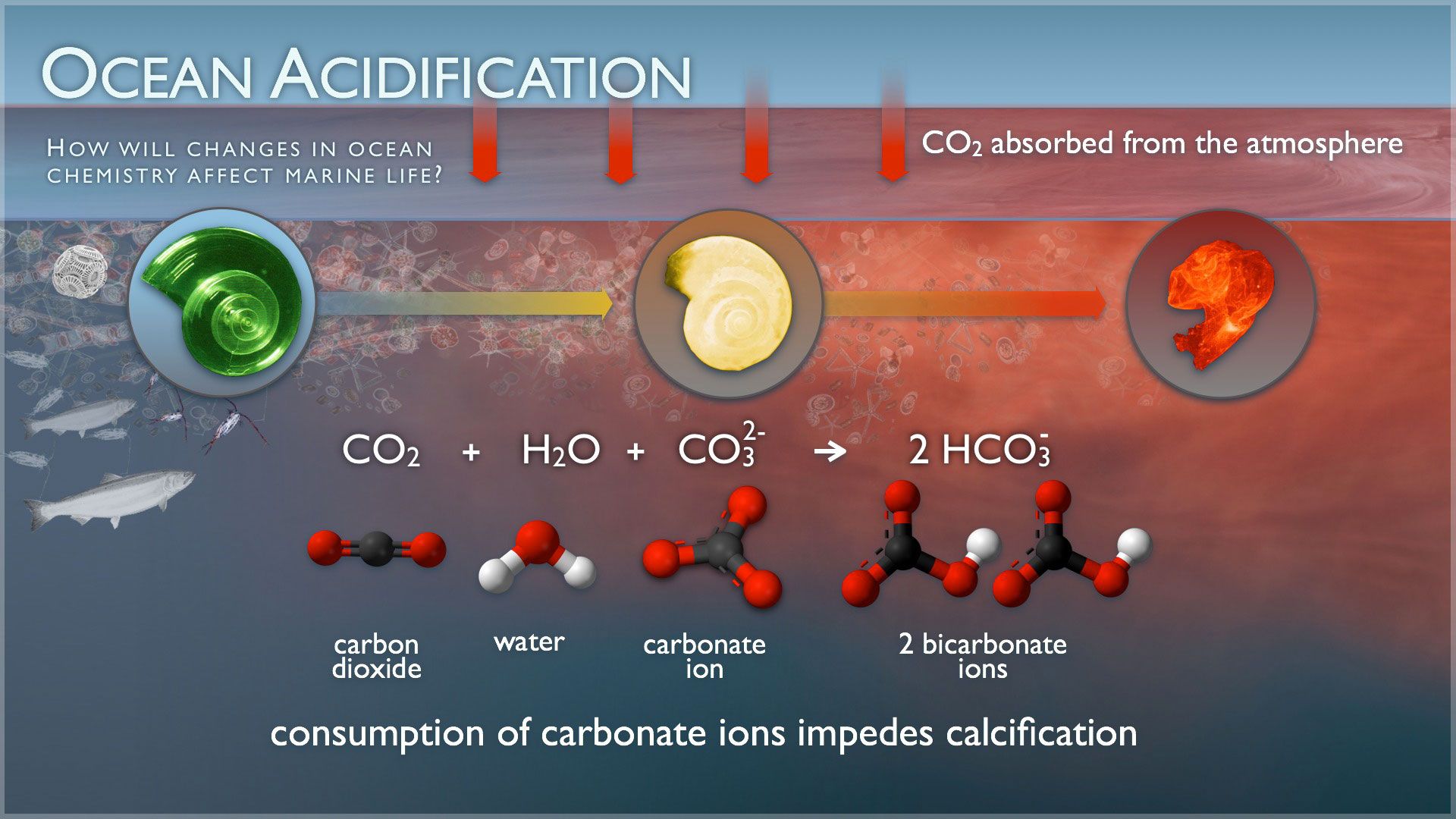
Credit: NOAA
The implications of ocean acidification for shell-forming organisms are profound. Organisms like diatoms, clams, and snails use calcium (Ca2+) and carbonate (CO32-) ions to build their calcium carbonate (CaCO3-) shells. In acidic oceans, CO32- becomes less available, so organisms must spend more energy to build their shells, which may even begin to dissolve (Doney et al. 2009). Ocean acidification can also impact the development of fish larvae and fish behavior (Cattano et al. 2018). For example, scientists have documented an increase of boldness and a disruption of anti-predator responses in fish exposed to higher CO2 levels (Heuer and Grosell 2014). Declines in wild and farmed fish and in shellfish populations due to acidification would likely disrupt the flow of energy through marine ecosystems, as well as negatively impact subsistence and commercial fisheries and shellfisheries (Doney et al. 2020). Solutions to dealing with ocean acidification on a global scale ultimately include strategies to reduce emissions to the atmosphere and remove CO2 from the atmosphere. On more local scales, the presence of submerged aquatic vegetation, like seagrass, can potentially mitigate elevated CO2 levels in coastal waters (Gattuso et al. 2018).
Seagrasses are marine plants found along coastlines globally (except for Antarctica; Short et al. 2007). They often form extensive meadows that harbor a diversity of sea creatures. Seagrasses directly affect water chemistry through plant metabolism: like all primary producers, they consume CO2 during photosynthesis and release CO2 during respiration. When seagrasses photosynthesize, they can remove some CO2 from seawater and make carbonate (CO32-) more available, at least temporarily, for shell-forming organisms. Where seagrasses coexist with shell-forming organisms, like clams and oysters, they can potentially prevent pH levels from dropping too low by drawing down CO2 from the water column, making conditions better for the growth of clams and oysters (Kapsenberg and Cryonak 2019). This effect on pH produced by seagrass has been observed in a few natural ecosystems (Ricart, Ward, et al. 2021) and in laboratory experiments (Ricart, Gaylord, et al. 2021). However, it is unclear how effectively seagrasses and other submerged aquatic vegetation can mitigate pH in different parts of the world.
Learning about seagrasses and their potential role in mitigating low pH in the coastal ocean will help students to understand how tightly linked biological and chemical components are in ecosystems and to think creatively about natural solutions to climate change. The hands-on activities presented here can be used to introduce or reinforce fundamental concepts in acid-base chemistry, plant metabolism, and carbon cycling. This lesson plan will contribute to state learning standards while generating appreciation for seagrasses among other important Florida natural resources and how they interact with the environment.
Overview
In this three-part activity, students will learn about the role of CO2 in waterbody acidity and how aquatic plants, such as seagrasses, can mitigate acidification by altering CO2 levels in water.
- Students will first gain familiarity with the pH scale by testing the pH of several household substances and discussing the qualities of acids, bases, and neutral solutions. This exercise will help students understand the term ‘acidification’ and prepare them to observe the subtle decrease of pH in carbonated water compared to unaltered water.
- Students will observe the effect of increased CO2 levels (via carbonation) on the pH of water and how acidified water reacts with chalk (made of calcium carbonate). This exercise will serve as an analogy to the impact of ocean acidification on shell-forming organisms: students will observe more effervescence when chalk is dissolved in carbonated water compared to chalk dissolved in tap water, demonstrating how shells made of a similar material are vulnerable to acidification and may dissolve in acidified waterbodies. Note that the chalk activity produces more dramatic results than shell dissolution because shells are composed of a crystalized form of calcium carbonate, which dissolves at a slower rate.
- Students will then hypothesize how aquatic plant metabolism (i.e., photosynthesis and respiration) may affect the pH of water. Several hours prior to the start of the lesson, the instructor will have prepared a simple incubation experiment to demonstrate the effects of photosynthesis (light conditions) and respiration (dark conditions) on pH. Seagrass may be difficult to obtain, so we suggest collecting marine macroalgae or purchasing aquaria plants instead. If the incubation experiment is successful, students will observe that CO2 consumption occurs under light conditions, increasing pH, while CO2 production occurs under dark conditions, lowering pH. This final exercise guides students to develop hypotheses based on prior observations and knowledge, test their hypotheses, and discuss the results.
Throughout the three-part activity, students will connect seemingly unrelated scientific principles and phenomena to better understand the impacts of ocean acidification and potential solutions. The following sections include the intended objectives related to performance standards, instructor and student protocols, a materials list and sourcing information, a worksheet template, assessment questions, and additional resources.
Objectives
Students will be able to:
- Define acids/bases and use pH measurements to classify household substances as acids/bases.
- Describe how CO2 concentration affects pH and the acidity of water.
- Apply basic knowledge of plant metabolism to predict how photosynthesis and respiration affect CO2 dynamics and pH/acidity in an aquatic environment.
Grade Level: 8
Subject Area: Physical Science, Life Science
Time
Preparation: preparing solutions—10–15 minutes; plant experiment set-up—5 minutes
Activity: part one—25 minutes; part two—15 minutes; part three—5 minutes
Clean-up: 10 minutes
Student Performance Standards (Sunshine State Standards)
- Identify basic examples of and compare and classify the properties of compounds, including acids, bases, and salts (SC.8.P.8.8).
- Describe and investigate the process of photosynthesis, such as the roles of light, carbon dioxide, water and chlorophyll; production of food; release of oxygen (SC.8.L.18.1).
- Describe and investigate how cellular respiration breaks down food to provide energy and releases carbon dioxide (SC.8.L.18.2).
- Construct a scientific model of the carbon cycle to show how matter and energy are continuously transferred within and between organisms and their physical environment (SC.8.L.18.3).
- Understand that scientific investigations involve the collection of relevant empirical evidence, the use of logical reasoning, and the application of imagination in devising hypotheses, predictions, explanations, and models to make sense of the collected evidence (SC.8.N.1.6).
Background
Ask students if they know why lemons are sour or why bleach is a good cleaning agent. Explain that all substances can be described as acids or bases, and acid-base chemistry can explain why some foods taste sour and why some liquids are good for cleaning. We can determine whether a solution is an acid or a base using a measurement scale called the pH scale. We need to know the pH of different substances to understand how they will affect our drinking water, human health, and the environment. In waterbodies, like streams, rivers, lakes and oceans, water can become more acidic due to higher levels of carbon dioxide (CO2)—this is called acidification. Waterbody acidification can harm aquatic organisms, but aquatic plants may be able to buffer the effects of acidification. The purpose of this activity is to familiarize students with the properties of acids and bases, measuring pH, and the concept of acidification, in which carbon dioxide shifts the balance of acids and bases in a solution.
Here are linked video clips for additional background on the topics covered in this activity:
- What Are Acids & Bases? https://www.youtube.com/watch?v=mnbS56HQbaU
- What is Ocean Acidification? https://oceanservice.noaa.gov/facts/acidification.html
- Carbon Dioxide, Shell Building, and Ocean Acidification https://www.whoi.edu/multimedia/carbon-dioxide-shell-building-and-ocean-acidification/
Instructor Materials
Quantities for the following items are based on a class size of 25 students (in groups of 5) testing 5 solutions (see Appendix I for sourcing information and estimated costs):
- 200 pH test strips with indicator keys
- ~10 oz of each household product of varying pH, examples include:
- Distilled white vinegar, lemon juice, tap water, baking soda, ammonia-based cleaner, etc.
- 210 2-oz clear plastic containers with lids
- Home soda maker (e.g., SodaStream) with bottle and CO2 cylinder
- Alternative: 1 12-oz can of club soda
- 5 ~½-in pieces of chalkboard chalk
- Aluminum foil
- Several sprigs of aquatic plants or macroalgae (Figure 2), examples include:
- Elodea, also known as American waterweed, a common aquaria plant
- Ulva, also known as sea lettuce, a common marine macroalgae
- Full spectrum LED grow light bulb installed in a clamp light fixture
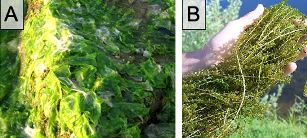
Credit: A) Caroline Donovan, Integration and Application Network (ian.umces.edu/media-library) and B) UF/IFAS Center for Aquatic and Invasive Plants
Instructor Guide
Preparation
- At least 8 hours before students begin the activity: Fill 2 plastic containers (for each group of students) with aquatic plants and water to the top of the container. Close the containers as tightly as possible. Cover one container with aluminum foil and leave the other uncovered. Place all plant-filled containers under the full spectrum light (Figure 3). If a full spectrum bulb is unavailable, the containers may be left outside in direct sunlight. For best results, the instructor should ensure the containers are exposed to the full spectrum light or bright sunlight up until the students are ready to measure the pH of the water within the containers.
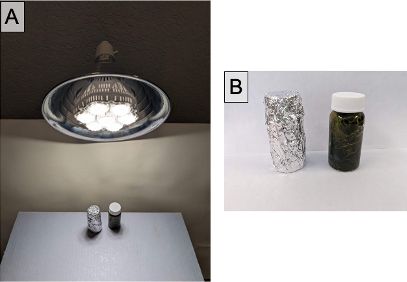
Credit: Alexandra L. Bijak, UF/IFAS
2. At least 10 minutes before students begin the activity: Gather materials, including household products of varying pH, preferably clear liquids. Baking soda can be dissolved into tap water to create a basic solution that is non-toxic. Ideally, the instructor will prepare solutions in at least one of each pH range: acidic (0-6), basic (8-14), and neutral (~7) solutions.
3. Label empty plastic containers numerically with the intent of using the numbered labels to code for each solution (2 per group of students).
4. Fill the labeled plastic containers halfway with solutions that match their numeric code (e.g., 1 = distilled white vinegar, 2 = dissolved baking soda, 3 = tap water, etc.).
Activity
Part One: Where do household substances fall on the pH scale?
- The instructor should guide students through each step of the activity, asking guiding questions and facilitating discussion along the way.
- Begin the activity with a safety warning that students should not touch or smell solutions unless given permission to do so. See Appendix II for a worksheet template.
- Tell students which substances are present in the solutions set. After they have guessed the identity of each solution based on their observations and pH measurements, reveal the identity of each solution.
Optional: At the end of Part One, allow students to mix weak acids and bases (e.g., vinegar and dissolved baking soda) to observe neutralization reactions. Do not allow students to mix strong acids and bases or cleaning products.
Guiding Questions:
- What properties of acids, bases, and neutral solutions did you observe?
- Which solutions were most difficult to identify and why?
- How can pH measurements be useful in everyday life?
Part Two: How does adding CO2 (carbonation) affect the pH of water?
- Using the home soda maker, create carbonated water. Explain that the soda maker pumps carbon dioxide (CO2) into the tap water. Distribute a plastic container filled halfway with the freshly carbonated water and 2 pieces of chalk to each student group.
- Explain that the chalk is made of calcium carbonate—the same material that makes up the shells of clams and many other organisms. (See Appendix III for examples.) Students living near Florida’s Gulf Coast may be familiar with commercially important eastern oysters (Crassostrea virginica), hard clams (Mercenaria mercenaria), and bay scallops (Argopecten irradians). Students living in South Florida may associate the queen conch (Strombus gigas) with the Florida Keys, where residents refer to themselves as “conchs” to reflect their laid-back lifestyle. Corals are also calcifying organisms and deposit calcium carbonate to create their exoskeleton, which is the rigid structure we see as a coral reef.
Guiding Questions:
- How did carbonating the tap water affect the pH (i.e., increased, decreased, etc.)?
- What do you think is happening when the chalk reacts with the solutions?
- How do you think organisms with shells might be impacted by acidification?
Part Three: How does aquatic plant metabolism affect the pH of water?
- Distribute the plant containers (1 with foil and 1 uncovered) per student group.
- Explain that photosynthesis and respiration consume and produce CO2, respectively. Ask students to formulate a hypothesis on how a submerged aquatic plant affects the pH of the surrounding water. Remind students that Florida hosts a variety of freshwater and marine plants, like eelgrass (e.g., Vallisneria americana) and seagrass (e.g., Thalassia testudinum).
Guiding Questions:
- Why do you think we kept the plant incubation containers closed for this experiment?
- If multiple plant species were incubated, which species had the greatest effect on pH?
Optional: See Appendix IV for assessment questions.
Clean-up
- Plastic containers can be rinsed and reused.
- Plant material should be left out to dry, then sealed in a plastic bag, and discarded in the trash. Do not dispose of plant material in waterbodies or natural systems. For more information on the proper disposal of classroom plant material, visit https://www.fws.gov/program/aquatic-nuisance-species-task-force/outreach.
Student Instructions
Activity
Part One: Where do household substances fall on the pH scale?
- Once assigned to a group, obtain the solutions set and pH test strips. Do not touch or smell solutions unless the instructor gives permission to do so.
- For each numbered solution, discuss its characteristics, such as color. Then dip a pH test strip into the solution and use the pH indicator key to measure the solution’s pH. Use a new pH strip for each solution.
- Use your observations and the pH measurements to guess the identity of each solution.
Part Two: How does adding CO2 (carbonation) affect the pH of water?
- Dip a pH test trip into the carbonated water as soon as you receive it from the instructor. Compare the pH of tap water to the pH of the carbonated water.
- Now drop a piece of chalk into the tap water and carbonated water. Compare how chalk reacts to tap water vs. carbonated water.
Part Three: How does aquatic plant metabolism affect the pH of water?
- When instructed to do so, retrieve 2 plant containers, 1 covered with foil and 1 uncovered. Quickly open the containers and dip a pH test strip into each container. Compare the pH from the plant containers that were left in dark vs. light conditions.
Anticipated Results
Part One: Where do household substances fall on the pH scale?
- Students will observe differences in pH across the household substances, but some substances will be easier to identify than others. For example, lemon juice and distilled white vinegar have strong scents.
- Students should recognize that acids, such as lemon juice, have a low pH (2.0-4.0), bases, such as baking soda, have a high pH (8.0-9.0), and neutral substances, like water, are close to a pH of 7.
Part Two: How does adding CO2 (carbonation) affect the pH of water?
- The carbonated water is more acidic and should have a lower pH (4.0-4.5) than the tap water.
- When placed in water, the chalk will ‘fizz’ and start to dissolve because the negatively charged calcium carbonate reacts with the more acidic water. There may be a stronger reaction for the chalk placed in the carbonated water.
Part Three: How does aquatic plant metabolism affect the pH of water?
- The plant containers were sealed up tightly and left under dark and light conditions for several hours. The plants left under dark conditions should have respired and produced CO2. The plants under light conditions should have photosynthesized and consumed CO2. Therefore, the containers left in the dark should have a slightly lower pH (more acidic) than the containers left in the light.
Explanation of Concepts
I. What are Acids and Bases?
Put simply, acids are chemicals that tend to give up protons (proton donors) while bases are chemicals that take protons (proton acceptors). Knowing whether a chemical pH is an acid or a base can provide information about its properties and allow us to predict how it will react with other chemicals. Acids are corrosive, meaning they react with some metals and cause them to dissolve, but bases do not react with metals. Citrus fruits, like lemons, taste sour because they contain citric acid. Soaps and detergents contain bases, so they taste bitter and feel slippery. We can also tell if a substance is an acid or a base by measuring pH, or the concentration of protons (hydrogen ions: H+) in solution (Figure 4). The pH range of acids is 0–6, and the range of bases is 8–14. Neutral substances, like pure water, have a pH of 7.
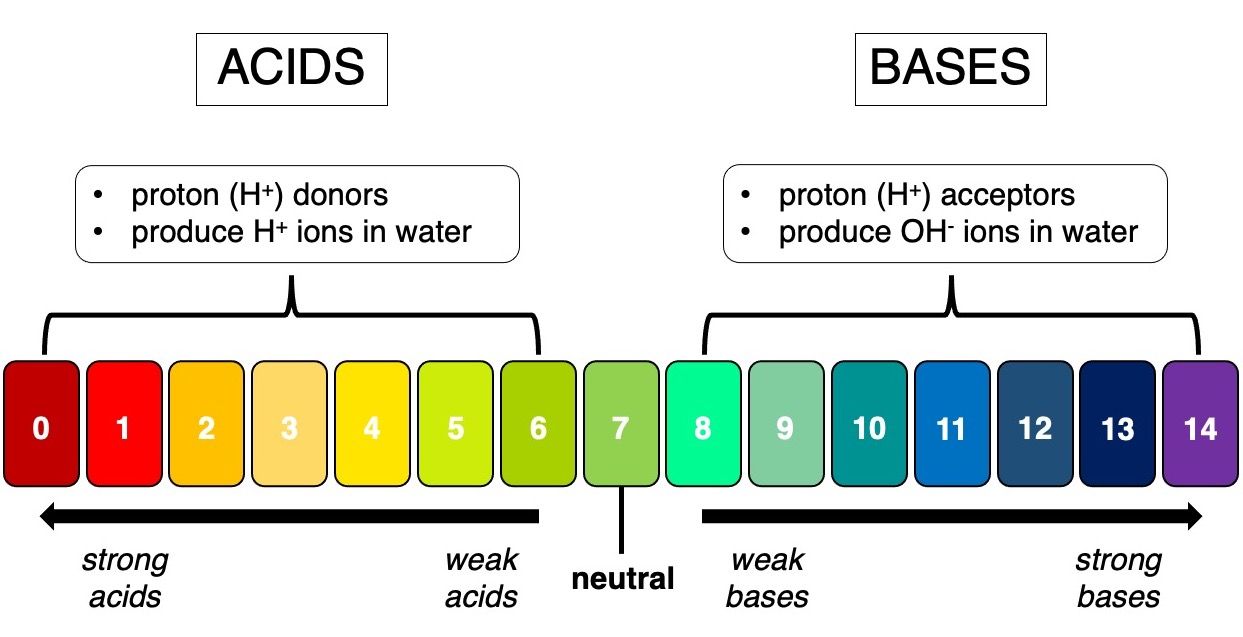
Credit: Alexandra L. Bijak, UF/IFAS
We need to know the pH of different substances to understand how they will affect our drinking water, human health, and the environment. Managers of drinking water systems must make sure the pH of water is near neutral so that it is safe to drink and not corrosive. Too low of a pH can be corrosive and leach metal ions from pipes as water enters the plumbing of a house. In our own bodies, we have several enzymes that perform all sorts of functions and require a specific pH to work properly: for example, there are digestive enzymes in our stomach that require an acidic environment (low pH). However, too much acidity from certain types of food such as orange juice, tomato sauce, or coffee can cause an upset stomach. Medicines like Pepto Bismol and Tums contain calcium carbonate, which is alkaline (basic) and helps to neutralize the acidity causing the upset stomach. Too low of a pH in natural systems, such as with acid rain in forests, can make the soil too acidic for many types of plants to grow.
II. Acid-Base Chemistry Affects the Environment
Even relatively weak acids can affect organisms and the environment because acids can dissolve materials made from carbonates. One example you can see on land is the effect of acid rain on manmade structures. Acid rain happens when rain droplets form in the presence of certain pollutants that make water more acidic. Over time, acid rain can erode buildings and statues made of limestone or marble, types of rock that contain carbonates.
Carbonation adds carbon dioxide (CO2) to water, which makes water slightly more acidic. CO2 levels have increased in the atmosphere since the industrial revolution in the 1860’s, mostly due to the burning of fossil fuels like coal and natural gas. CO2 from the atmosphere diffuses into the ocean and makes it more acidic—this process is called acidification. If the ocean becomes too acidic, organisms such as clams, oysters, and corals are at risk because their shells and skeletons are made from carbonates.
III. Aquatic Plants Can Affect Waterbody pH
Like all plants, aquatic plants and algae that grow underwater use sunlight, water, and CO2 during photosynthesis to produce sugars as their main energy source. When exposed to sunlight, plants photosynthesize, consuming CO2 and releasing oxygen (O2). When plants are kept under dark conditions, plants respire (or “breathe”), consuming O2 and releasing CO2. So, when aquatic plants photosynthesize and draw down dissolved CO2 in the water, they can make the water they live in slightly less acidic (Figure 5). Scientists hypothesize that aquatic plants can help organisms vulnerable to acidification, like clams and oysters, survive as oceans and other waterbodies become more acidic.
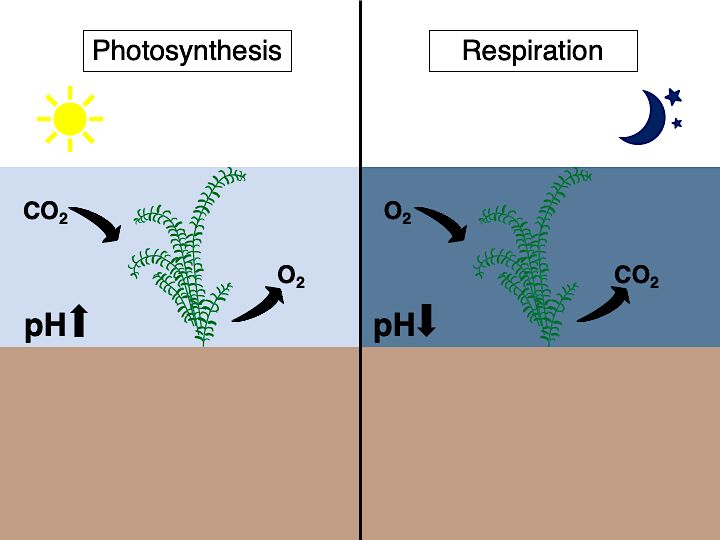
Credit: Alexandra L. Bijak, UF/IFAS; adapting plant and arrow symbols from Tracey Saxby and Alexandra Fries, Integration and Application Network (ian.umces.edu/media-library)
Additional Resources
United States Geographical Survey on pH and Water: https://www.usgs.gov/special-topics/water-science-school/science/ph-and-water
United States Environmental Protection Agency on Acid Rain: https://www.epa.gov/acidrain/what-acid-rain
National Association of Geoscience Teachers – Teach the Earth on Ocean Acid Demonstration Guide: https://serc.carleton.edu/teachearth/activities/181167.html
Minnesota Science Teachers Education Protect on Investigating Photosynthesis: https://serc.carleton.edu/sp/mnstep/activities/35653.html
References
Ardoin, N. M., A. W. Bowers, and E. Gaillard. 2020. “Environmental Education Outcomes for Conservation: A Systemic Review.” Biological Conservation 241: 108224. https://doi.org/10.1016/j.biocon.2019.108224
Cattano, C., J. Claudet, P. Domenici, and M. Milazzo. 2018. “Living in a High CO2 World: a Global Meta-Analysis Shows Multiple Trait-mediated Fish Response to Ocean Acidification.” Ecological Monographs 88 (3): 320–335. https://doi.org/10.1002/ecm.1297
Doney, S. C., V. J. Fabry, R. A. Feely, and J. A. Kleypas. 2009. “Ocean Acidification: The Other CO2 Problem.” Annual Reviews of Marine Science 1: 169–192. https://doi.org/10.1146/annurev.marine.010908.163834
Doney, S. C., D. Shallin Busch, S. R. Cooley, and K. J. Kroeker. 2020. “The Impacts of Ocean Acidification on Marine Ecosystems and Reliant Human Communities.” Annual Review of Environment and Resources. https://doi.org/10.1146/annurev-environ-012320-083019
Gattuso, J. P., A. K. Magnan, L. Bopp, W. W. L. Cheung, C. M. Duarte, J. Hinkel, E. Mcleod, et al. 2018. “Ocean Solutions to Address Climate Change and its Effects on Marine Ecosystems.” Frontiers in Marine Science 5: 337. https://doi.org/10.3389/fmars.2018.00337
Heuer, R. M., and M. Grosell. 2014. “Physiological Impacts of Elevated Carbon Dioxide and Ocean Acidification on Fish.” American Journal of Physiology-Regulatory, Integrative and Comparative Physiology 307 (9): R1061–R1084. https://doi.org/10.1152/ajpregu.00064.2014
Kapsenberg, L., and T. Cyronak. 2019. “Ocean Acidification Refugia in Variable Environments.” Global Change Biology 25 (10): 3201–3214. https://doi.org/10.1111/gcb.14730
Likens, G. E. 1976. “The acidity of rain and snow falling on parts of the U.S. and Europe has been rising—for reasons that are still not entirely clear and with consequences that have yet to be well evaluated.” Chemical & Engineering News 54 (48): 29–44. https://doi.org/10.1021/cen-v054n048.p029
Ricart, A. M., M. Ward, T. M. Hill, E. Sanford, K. J. Kroeker, Y. Takeshita, S. Merolla, et al. 2021. “Coast-wide Evidence of Low pH Amelioration by Seagrass Ecosystems.” Global Change Biology 27 (11): 2580–2591. https://doi.org/10.1111/gcb.15594
Ricart, A. M., B. Gaylord, T. M. Hill, J. D. Sigwart, P. Shukla, M. Ward, A. Ninokawa, and E. Sanford, et al. 2021. “Seagrass-driven Changes in Carbonate Chemistry Enhance Oyster Shell Growth.” Oecologia 196: 565–576. https://doi.org/10.1007/s00442-021-04949-0
Short, F. T., T. J. B. Carruthers, W. C. Dennison, and M. Waycott. 2007. “Global Seagrass Distribution and Diversity: A Bioregional Model.” Journal of Experimental Marine Biology and Ecology 350 (1–2): 3–20. https://doi.org/10.1016/j.jembe.2007.06.012
van de Wetering, J., P. Leijten, J. Spitzer, and S. Thomaes. 2022. “Does environmental education benefit environmental outcomes in children and adolescents? A meta-analysis.” Journal of Environmental Psychology 81: 101782. https://doi.org/10.1016/j.jenvp.2022.101782
Weiss, L. C., L. Potter, A. Steiger, S. Kruppert, U. Frost, and R. Tollrian. 2018. “Rising pCO2 in freshwater ecosystems has the potential to negatively affect predator-induced defenses in Daphnia.” Current Biology 28 (2): 327–332.e3. https://doi.org/10.1016/j.cub.2017.12.022
Appendix I: Sourcing Information and Cost Estimates for Instructor Materials
Table 1. Cost estimates for materials.
Appendix II: Worksheet
Part One—Measure pH to identify unknown solutions.
Part Two—Examine how carbonation (adding carbon dioxide) affects pH.
Part Three—Explore how plant photosynthesis and respiration affect pH.
Appendix III: Common Shell-forming Organisms of Florida.
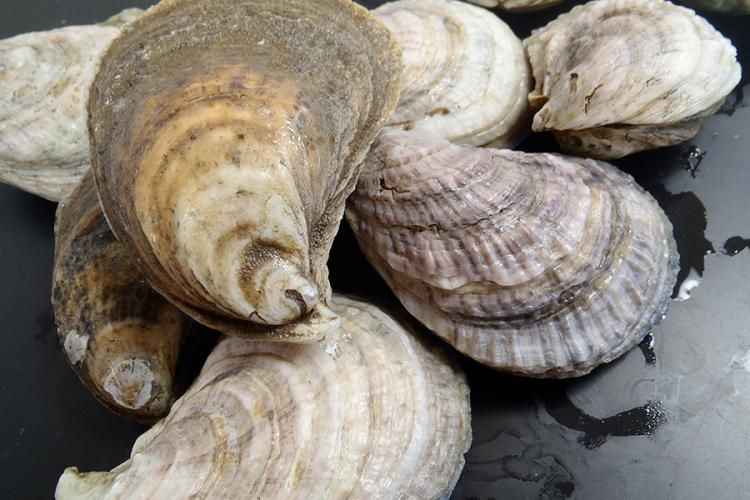
Credit: NOAA
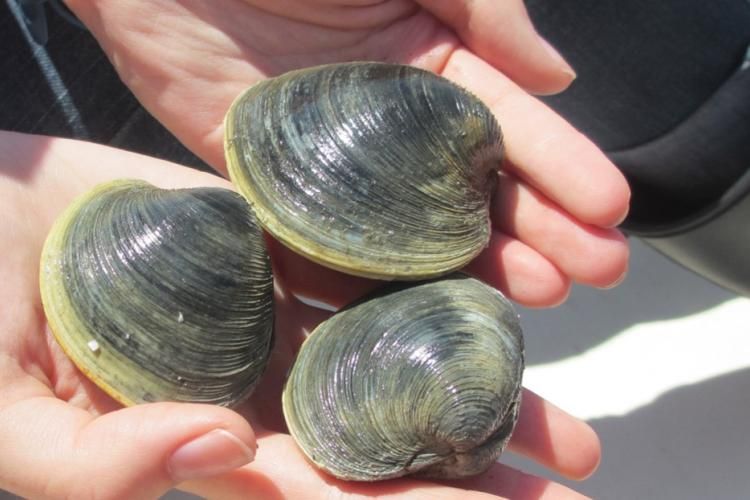
Credit: NOAA
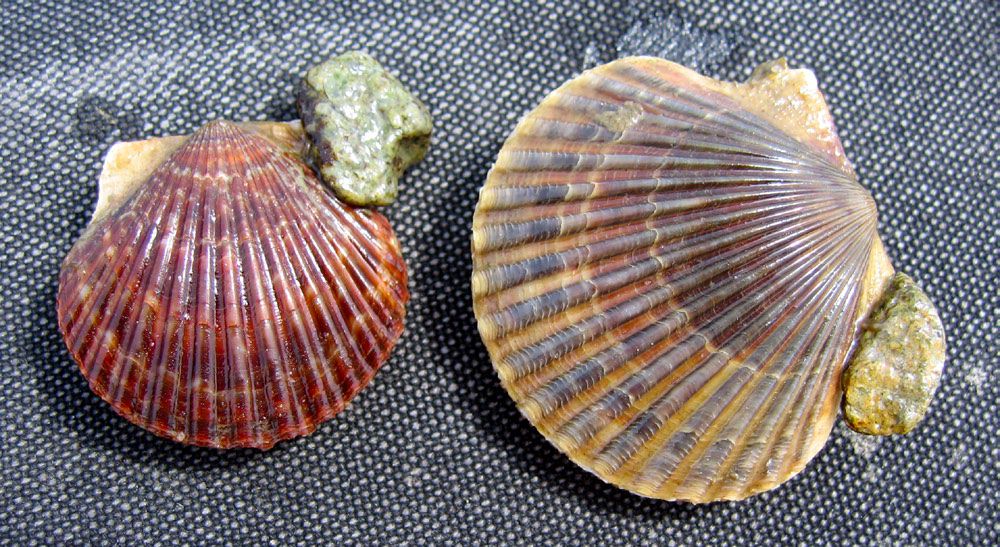
Credit: NOAA
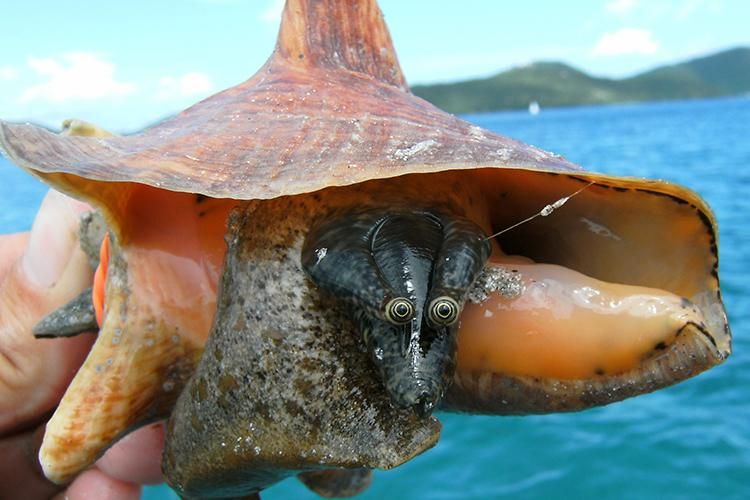
Credit: NOAA
Appendix IV: Assessment Questions
- Classify the following solutions as an acid or a base:
___ Coffee (pH = 5)
___ Soap (pH = 9)
___ Pure water (pH = 7)
___ Orange juice (pH = 3.9)
___ Bleach (pH = 11)
2. A) Describe how carbonation of water affects pH. B) If the enamel covering your teeth is made of a mineral similar to calcium carbonate (like chalk), then how might carbonated beverages affect your teeth?
3. Draw a diagram showing how aquatic plant metabolism (photosynthesis and respiration) affects the pH of water. Include the sun, a waterbody, a plant, CO2, and O2.
Appendix V: Glossary
Acid: a substance that produces hydrogen ions (H+) in solution, so it is termed a proton donor. Solutions that range in pH from 0–6 are referred to as acidic.
Base: a substance that accepts hydrogen ions (H+) in solution, so it is termed a proton acceptor. Solutions that range in pH from 8–14 are referred to as alkaline or basic.
Bicarbonate (HCO3-): one of three dissolved compounds in ocean water that primarily constitute the carbonate system.
Buffering capacity: the ability of an aquatic system to resist changes in pH.
Calcium carbonate (CaCO3-): the mineral that shell-forming organisms use to build their shells (or exoskeletons); for this reason, shell-forming organisms are referred to as calcifying organisms.
Carbonate (CO32-): one of three dissolved compounds in ocean water that primarily constitute the carbonate system; carbonate is used with calcium ions to form the mineral calcium carbonate.
Carbonate system: the dissolved compounds (carbon dioxide, carbonate, and bicarbonate) in the ocean that control the acidity of seawater and play a large role in the global carbon cycle.
Carbon dioxide (CO2): a gas released into the atmosphere from the burning of fossil fuels and natural processes and has a strong effect on earth’s climate and ocean pH.
Ecosystem: where all the organisms (e.g., plants, animals) and the non-living components (e.g., light, water) of the physical environment interact with each other.
Ocean acidification: the decrease in seawater pH over an extended period of time, primarily due to the uptake of atmospheric carbon dioxide.
pH: a measurement used to describe the acid-base properties of solutions; specifically, pH is the inverse measure of hydrogen ion (H+) activity (the negative log concentration of H+) so that low values on the pH scale indicate high H+ activity. The pH scale is a logarithmic scale because the H+ activity of solutions can span several orders of magnitudes (1 to 10-14). So, a solution with a pH of 4 is ten times more acidic than a solution with a pH of 5.
Photosynthesis: the process by which plants create their own food (sugars) from carbon dioxide and water.
Respiration: the process by which plants use oxygen and sugars to generate energy for growth.
Seagrass: a group of species of underwater marine plants that grow in shallow waters fringing the coastlines (not to be confused with algae or seaweed).