Florida agriculture lost 25% of its land use in the last 30 years with a significant portion taken out of production by government buyouts enforcing environmental regulations for the purpose of reducing phosphorus and nitrogen discharges to lakes, rivers, springs, and wetlands. Producing crops aquaponically can reduce leaching, runoff, and water discharges to the environment by reusing nutrient effluent from aquaculture and hydroponic systems. Designing and managing agricultural production systems for minimal discharge of water and nutrients to the environment protects groundwater quality, makes agricultural water permitting easier to obtain, and will help maintain the long-term sustainability of agricultural enterprises. These designs also will reduce concerns about discharge of nutrients into coastal zones that could contribute to harmful algal blooms.
Aquaponics is an intensive sustainable agricultural production system that connects hydroponic and aquaculture systems to produce multiple cash crops with reduced water and fertilizer inputs. It is highly suited for small farm producers targeting local markets and agritourism opportunities (Figure 1).
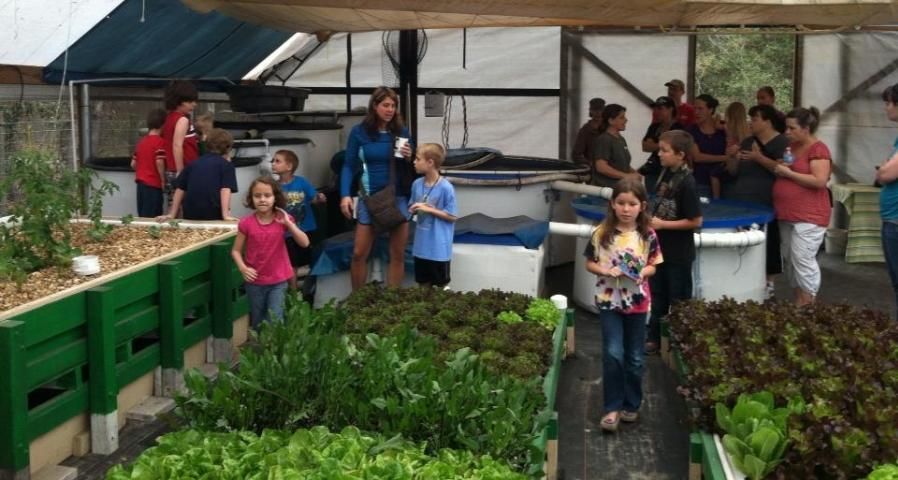
Credit: Green Acre Aquaponics
Choosing the Fish and Plants
Three organisms are involved in the optimum performance of aquaponic systems (Table 1). The plants and fish are the cash crops, while nitrifying bacteria play an important biofiltration role, converting toxic fish waste ammonia (Francis-Floyd et al. 2012) to nitrate nitrogen (Figure 2), one of the most important mineral nutrients required by plants.
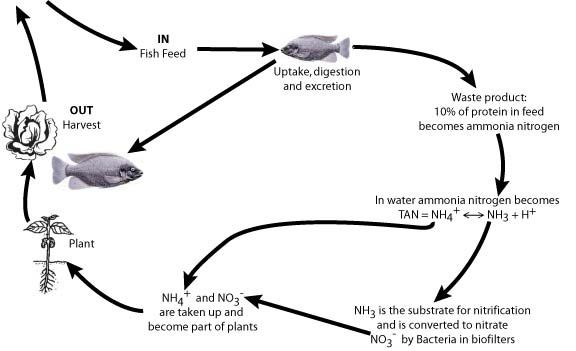
The choices of fish and aquatic organisms have an economic, environmental, and legal component (see the regulations section below). For example, tilapia is one of the most common aquacultured fish due to its tolerance of a wide range of water quality conditions (Lim and Webster 2006), its favorable feed conversion ratio (1.5–3 pounds of feed/pound of fish [Chapman 2012]) and its fast growth under warm conditions (market size in 6–12 months). It fits well when grown with warm-season vegetable crops like tomatoes, peppers, or cucumbers (Table 2). Tilapia has high-quality, textured white fillets, which present well at restaurants. Worldwide, tilapia farming is expanding at a rate of 12% to 15% annually. However, tilapia are sensitive to low temperatures and stop feeding at 60°F, with lethal temperatures beginning at 50°F and below. Also, inexpensive foreign imports depress wholesale prices, resulting in the need for farmers to seek marketing outlets that cater to the local production of food or live fish markets where higher prices could be expected. Other fish suitable to aquaponics but requiring more stringent water-quality conditions are channel catfish, koi, and other ornamental and bait fish. Malaysian prawn and red claw crayfish also have been used in aquaponic systems, but because they are non-native species their use in Florida is restricted (Knickerbocker 2013).
High-value vegetable crops that are commonly grown in hydroponic systems such as tomato, cucumber, pepper, lettuce, and mixed herbs are recommended for successful commercial aquaponic production (Tyson et al. 2013). However, matching the right plant to the right hydroponic system is an important decision. All plants can be grown hydroponically, but to maximize yields, plants that grow best in drier, well drained soils do best in media hydroponic systems while plants that prefer well-watered, continuously moist soil (most leafy salad crops and herbs) adapt well to either water culture or media systems (Table 3).
Production Methods
The most common aquaculture system used in aquaponics is the recirculating aquaculture tank system (RAS). This tank becomes the base nutrient and water reservoir that flows to the hydroponic subsystem and is usually recirculated back to the tank. This is an intensive, usually high-fish-density production system (Timmons et al. 2002) that allows for buildup of waste nutrients from fish feed to levels that can benefit plant growth. Pond water may contain harmful microorganisms and algae, so the use of well water or municipal water sources when using RAS aquaponics is recommended.
The most common hydroponic systems (Tyson et al. 2013) currently being used in aquaponics are the floating raft, nutrient film technique (NFT), and the bench bed hydroponic systems (Table 3). Floating raft systems are ideally suited for quick-turnaround lettuce crops. Many commercially available NFT systems can handle only small-rooted crops like lettuce because of limited trough volume (4 in. wide x 2 in. deep). Large-rooted vegetables such as tomato, cucumber, pepper, and mint can be grown with NFT provided the trough's root-zone space is large enough (18 in. wide x 4 in. deep) to accommodate the plant roots and allow water to continue flowing down the trough. Properly designed and operated media (perlite, vermiculite, peat, coconut coir, pine bark, pebbles, and combinations) systems, such as the bench bed, have the broadest crop choices because they can accommodate water-loving plants or plants that need well-drained soils. Often, media-filled plastic pots are placed in the bench bed to facilitate crop cycles and make the beds easier to clean and maintain.
Water Source and Quality Considerations
Theoretically, almost any type of vegetable production system could be linked to an aquaculture system. However, linking pond aquaculture to hydroponics unnecessarily introduces potentially harmful microorganisms and algae to the aquaponic system, which could adversely affect the fish and plants. Thus it is recommended to use well water or municipal water sources when using RAS aquaponics. Municipal water sources contain chlorine and/or chloramines, which are very toxic to fish and can kill them when present in very low concentrations. To minimize the risk of chlorine exposure, municipal water should be aerated in a separate tank for a few days before placing in the aquaculture tank. Ideally, water should be tested for the presence of chlorine before it is allowed to come in contact with the fish.
Determining the best production methods to use can be complicated by the various optimum temperatures and pH for maximum growth and yield of the three major organisms necessary for successful operations (Table 2). This often results in a compromise, depending on which plant and fish you choose to produce. A good starting point for warm-season vegetables, tropical fish, and nitrifying bacteria would be to keep the water pH between 6.5 and 7.5. Plant uptake of certain nutrients is better at pH 6.5. Nitrification rate increases at pH 7.5, speeding the reaction which turns toxic ammonia (NH3) into nitrate nitrogen (NO3-), the preferred nitrogen source for plants. At pH 7.5, micronutrient deficiency in plants (primarily iron and manganese) may occur but can be overcome by foliar nutritional sprays of the deficient nutrients. Many factors can influence system water pH, including:
-
Nitrification produces hydrogen ions and consumes carbonate ions, thus reducing water pH.
-
Uptake of nitrate ions by plant roots results in the secretion of hydroxide ions, thus increasing pH.
-
The natural pH of replacement source water can raise or lower the pH of the aquaponic system.
To increase pH to recommended levels, add calcium hydroxide or potassium hydroxide. To reduce pH to recommended levels, add sulfuric, phosphoric, citric, or hydrochloric acid. Caution needs to be exercised here: Any chemicals, like these pH adjusters, which can quickly change tank-water quality, should be added to a separate smaller tank and slowly added into the fish tank so the water quality changes occur gradually, which will reduce stress on the fish. Use of calcium carbonate (limestone) or magnesium-calcium carbonate (dolomite) can provide a slower rise in pH and a longer-lasting buffer.
Another important consideration for pH is its effect on the level of toxic ammonia (NH3). Tank-water ammonia exists in two forms, which together are called the total ammonia nitrogen (Francis-Floyd et al. 2012) or TAN (NH4+ ? NH3 + H+). Water temperature and pH will affect the percentage of each compound in the TAN equilibrium. For example, at 82ºF, the percentage of NH3 increases by nearly a factor of 10 for each 1.0 increase in pH and is 0.2%, 2%, and 18% of the TAN for pH 6.5, 7.5, and 8.5, respectively. The beneficial nitrification rate, which changes toxic ammonia to the plant-nutrient nitrate nitrogen, also increases as pH increases from 6.5 to 8.5 (Tyson et al. 2008), utilizing the NH3 as the beginning substrate in the reaction. Thus growers should consider the balance between pH and toxic ammonia verses the sustainability factor of driving the biological production of nitrate nitrogen. If the aquaponic system is balanced with ammonia levels between 0 to 1.0 ppm (1 ppm = 1 mg/L), pH could range between 6.5 and 7.5, with 6.5 more advantageous for the plant when TAN is 0 and nitrification more advantageous for the system from a sustainability point of view driving nitrate production when the TAN is 1.0 ppm. Tanks experiencing consistently higher ammonia levels >2.0 ppm may want to reduce pH to lower the toxic concentration of NH3, thereby reducing stress on the fish. In addition, stop feeding and begin water replacement to return TAN to safe levels before considering production at higher pH levels.
Besides regular measurements of pH and TAN, oxygen measurements also should be taken periodically. The most common causes of fish mortality are prolonged high ammonia (>2 ppm) and low oxygen (<3 ppm) levels. These conditions can be made worse if high fish densities and feed levels are maintained. A reasonable fish density for small farms is one pound of fish per cubic foot of aquaculture tank space (about 7.5 gallons). Higher densities (up to ½ lb/gal) can be maintained with 24-hour monitoring and backup pumps and generators ready in case of a power outage. For oxygen emergencies, stop feeding and increase aeration and water movement until oxygen returns to safe levels, >4 ppm. Refer to the Fish Health section below for further discussion of water quality.
System Sizing and Startup
System sizing recommendations depend on a number of variables. RAS recommendations suggest that 5%–10% of fish tank water volume be discharged daily and replaced with fresh water to help keep the tank water clean (Timmons et al. 2002). If the hydroponic subsystem is designed with enough plants to use up this discharge water through plant uptake and system evaporation (evapotranspiration), then the resulting aquaponic system can be maintained at near zero water discharges for both production systems. For this to occur—creating optimum system sustainability with minimal water discharges to the environment—the hydroponic subsystem should be much larger in surface area than the aquaculture subsystem. The lettuce/tilapia RAS floating raft aquaponic system (Rakocy et al. 1997) uses a ratio of 7.3:1, plant-to-fish surface area. Larger, fruiting greenhouse crops such as cucumber and tomato use more water than lettuce, requiring as much as 2 quarts of water per plant per day. Given that plants are often present at different stages of development from seedling to mature, in order to maintain consistent year-round aquaponic system water use, an average use of 1 quart per plant per day, depending on the average overall plant size, could be planned for hydroponic subsystems that use larger plants.
To help maintain good water-quality conditions, additional filtration of RAS tank-water solids usually is required and a separate biofilter for the nitrifying bacteria is recommended, especially with high densities of fish. Plants do have a biofilter role by removing ammonium (NH4+) from system water (Fig. 2), which reduces the concentration of the toxic ammonia (NH3) in the TAN equilibrium (NH4+ ? NH3 + H+). However, research suggests that nitrification is more important than plant uptake for biofiltration of the toxic ammonia (Tyson et al. 2008), and only nitrification can change ammonia to nitrate, the latter being the most common mineral nutrient required by plants.
Aquaponic system start-up procedures vary (Rakocy 2011; Timmons et al. 2002) depending on whether you rely solely on the fish to supply ammonia and on natural bacterial inoculation, or you introduce a nitrifying bacteria culture and provide a base of hydroponic nutrients (usually ¼ strength) to jump-start the system. The latter method provides a quicker start-up and allows introduction of plants into the system early on, without waiting on buildup of nutrients from fish waste. Establishing nitrifying bacteria in a biofilter is a 3–4 week process that should be completed before adding large amounts of fish to the tank. Once established, the bacteria will help prevent the buildup of ammonia and nitrite to levels that can be harmful to the fish. One successful start-up scenario could proceed as follows:
Day 1: Set up both the aquaculture and hydroponic subsystems without fish or plants.
Day 2: Fill tank water and adjust pH to 7.0–7.5, allowing a day to stabilize.
Day 3: Start pumps, allowing the system to run as planned.
Day 3: Add a broad spectrum hydroponic nutrient mix at about ¼ strength.
Day 3: Add household ammonia to the aquaculture tank for a final concentration of 3–5 ppm total ammonia nitrogen (TAN).
Day 4: Re-check pH and add a nitrifying bacteria culture to the biofilter and tank water.
Day 5: Plants may be added to the system.
Weeks 3–4: When ammonia and nitrite levels have resolved to near zero, add fish.
After start-up, never add ammonia nitrogen or urea to the tank water—the fish will produce enough ammonia to keep the nitrifying bacteria well fed and a properly balanced system will quickly change ammonia to nitrate nitrogen through the process of nitrification.
High feeding vigor is a good sign of healthy fish and reasonably good water quality. If fish are not vigorously feeding when food is first put into the tank, it could be a sign of poor fish health, poor water quality, and/or over feeding. Floating feed allows for better observation of this behavior. Maintaining good water quality is vital to keep fish healthy. The amount of fish feed to apply to the tanks depends on the size of the fish. Young fish (<25 g) usually are fed 6%–15% of their body weight per day, and older fish (>25 g) 1%–3% of their body weight (Chapman 2012). Spreading this daily feeding allowance over multiple feedings per day increases growth and reduces food waste.
Plant Environment and Nutrient Solutions
Controlling daytime air temperatures to accommodate maximum plant growth and yield (Table 2) is complicated by weather conditions and the degree to which you can control the growing environment. It is recommended to use some type of protected agriculture production system such as screen/shade, low or high tunnels, or greenhouses to reduce plant stress and control the environment to some degree. The pad and fan greenhouse is the most expensive of the latter options and provides the most control of factors that affect growth, disease, and insect-pest pressure. Indoor production, which has potential in urban areas, can be a costly alternative option because light also must be artificially supplied. A simple inexpensive outdoor shade structure providing 30% shade can reduce plant stress, especially during hot summer months. Fish tanks should be mostly covered to keep out sunlight, thereby reducing algae buildup.
Nighttime air temperatures during winter can be allowed to drop in greenhouses to 60°F–65°F to save energy. In Florida during the summer, night temperatures usually are above 72°F, and most tomato varieties will not set fruit under those conditions. For this reason, many southeastern US greenhouse tomato operations produce a fall/winter/spring cropping season with summer designated for cleanup and prepping for the next season. This may require a switch to different vegetable crops in the summer to supply the year-round plant biofiltration and water-use role important for aquaponics to operate sustainably with continuous plant production.
System plant-nutrient solutions should consider the daily addition of nutrients supplied by fish feed and the nutrient content of source water. In aquaculture, the generation of ammonia (NH3-N) in recirculating water through fish waste is based on the fish feeding rate:
P TAN = F x PC x 0.092
T
Abbreviations are F = feed weight, PC = protein content of the feed (%), T (time) = 1 day. Thus 1 kg of fish feed with 30% protein will produce 27.6 g of N in 1 day (Timmons et al. 2002). A rule of thumb to use for calculating this is that 10% of the protein in fish feed will become ammonia nitrogen in the recirculating water (Figure 2).
Other nutrients required by plants also are present in fish feed, but calcium, potassium, and iron are not found in adequate amounts and so must be added separately (Rakocy 2011). Addition of a quarter-strength broad-spectrum hydroponic nutrient formulation also can help jump-start plant growth when needed, especially during aquaponic start-up cycles. In general, fruiting crops require a higher concentration of nutrients in the solution, with tomato (Hochmuth and Hochmuth 2012) being one of the heaviest feeders compared to leafy salad crops and herbs. Soluble salt levels for optimum hydroponic vegetable production usually range between 1.5 and 2.5 millimhos/cm (dS/m), with lower levels for leafy salad crops and herbs and higher levels for fruiting crops. However, since feed is being added daily and changed into nutrients, and this nutrient-laden water is moving past the plant roots regularly in most aquaponic systems, soluble salt levels in aquaponic production usually can be maintained at about 1/3 of the concentration used in hydroponics and still provide adequate plant nutrition (Rakocy et al. 1997). More research in this area is needed to establish firm recommendations for using plant nutrients in aquaponics. When plant nutrition and health is in doubt, defer to the hydroponic recommendations for best results (Hochmuth and Hochmuth 2012; Resh 2013).
Fish Health
Maintenance of fish health in aquaponics systems is more challenging than in "fish only" aquaculture. Maintaining good water quality is vital to keep fish healthy, eating, and growing. Important water-quality parameters include ammonia, nitrite, pH, temperature, dissolved oxygen, alkalinity, and hardness. At the very least, TAN, pH, oxygen, and nitrite should be tested once a week, and more often during start-up or when major changes in fish or plant numbers occur.
One of the most common causes of fish stress and mortality is high ammonia levels in tank water. For every gram of toxic ammonia nitrogen that is reduced to relatively non-toxic nitrate nitrogen by nitrifying bacteria, 7.14 grams of alkalinity is consumed (Timmons et al. 2002), reducing pH. Nitrification slows considerably as pH becomes more acid. Therefore, in order to keep the biofiltration of toxic ammonia active, alkalinity should be tested once or twice a month or any time unexpected changes in pH are detected, and maintained around 50 ppm (mg/L) or higher depending on your target pH. Adequate levels of nitrate nitrogen needed for plant production in recirculating tank water should be safe for most common aquaponic fish, but some fish stress and reduced growth could occur if nitrate levels exceed those necessary for the plants (50–125 ppm N).
Fish behavior can provide information for later assessment. For example, fish gulping for air at the water's surface often indicates low oxygen in the tank water. The most common sign that fish may be getting sick is a change in behavior, usually first noticed as a sudden decrease in or complete cessation of feeding activity. In addition to changes in feeding activity, abnormal behavior also may include darkening or other color change, unusually slow movement, hanging or swimming in an unusual position (relative to the normal position of the species), swimming abnormally/spinning, lying on the bottom, or dead fish. Other signs of disease may include the presence of lesions (such as reddened areas), hemorrhages, torn or eroded fins, ulcers, white eyes, bumps or lumps, and increased mucus production. If a fish disease is suspected, water should be tested and appropriate management (e.g., water changes) carried out if indicated. Work with a fish veterinarian/health specialist who can help determine the cause of disease and suggest management options (Yanong 2012).
Regulations in Florida
Floridians who are raising aquatic species for commercial sale must annually acquire an Aquaculture Certificate of Registration from the Florida Department of Agriculture and Consumer Services (fdacs.gov/divisions-offices/aquaculture). This certificate is based on producers agreeing to abide by a set of best management practices that cover all aspects of commercial aquaculture. Transfer of live fish that require a certificate to possess can be made only from one permit/certificate holder to another. Recently killed fish may be sold to the general public "whole pondside" from the farm on agriculturally zoned land within Florida.
Many non-native animal species, such as tilapia, red claw crayfish, and Malaysian prawn, require certificates of registration from the Aquaculture Division of the Florida Department of Agriculture to produce commercially and are not allowed for personal possession by hobbyist. Blue tilapia may be possessed, cultured, and transported without a permit in central and south Florida south of a line drawn diagonally across the state from Crystal River to St. Augustine (Knickerbocker 2014). Non-native species comprise the bulk of aquacultured aquatic organisms in Florida and regulations are subject to change (Hill 2013), so before investing in an aquaponic facility do your homework to avoid costly financial mistakes and ensure compliance with existing regulations.
Fruits and vegetables may be sold from an agriculturally zoned property where they are grown without special permits, but once taken off the property they are subject to the rules and regulations at the point of sale. Small farms usually are not required to have vegetable produce inspected if they are not specifying the product is a particular size or grade (such as US No. 1 large). Regional marketing requirements are in effect for commercial tomato production in Florida, so check with the Florida Department of Agriculture and Consumer Services Marketing Division (freshfromflorida.com) for updates.
Marketing and Postharvest Handling
Growing is only half of a successful farming operation. Identifying someone in the family/organization with enthusiasm and good people skills to manage the marketing of the product is the other half. As with all agricultural commodities, make sure you have a firm buyer/price before beginning production. Greenhouse tomatoes have the potential for high returns provided yields are maximized and sales are close to retail price (Table 4). Competing with field-grown tomatoes (about $0.50/lb), selling to restaurants (about $1.50/lb), or getting the full potential retail price (about $2.00–$2.50/lb) significantly affects gross returns. Hydroponic lettuce also provides a quick return since each crop can be marketed in about 30 days from transplant. The United States Department of Agriculture (USDA) provides current wholesale price market commodity reports at http://www.ams.usda.gov/AMSv1.0/. Local retail prices can be checked at grocery stores.
How one handles fruits, vegetables, and aquaculture products after harvest has a profound effect on the length of time the product quality is maintained—the shelf life (USDA/ARS 2004). Fish in RAS tank culture often have off-flavor requiring purging before selling. Stop feed 24 hours before purging and do not feed again. Move fish to be purged to a fresh tank of water for 3–5 days and replace 25%–200% of the water daily to remove pollutants. Taste test unseasoned fillets by cooking in a microwave to be sure off-flavor is gone (Timmons et al. 2002). Price, quality, postharvest handling, and good marketing skills equal success.
Food Safety Considerations
Both fish and edible plants are regulated by the US Food and Drug Administration, with the latest food safety rules being administered through the Food Safety Modernization Act (FSMA) https://www.fda.gov/food/guidance-regulation-food-and-dietary-supplements/food-safety-modernization-act-fsma. Fish are regulated under the seafood HACCP rule and plants under the Produce Safety rule.
Processing fish in any way by cutting or scaling them necessitates the use of a regulated food safety compliant processing facility which can be expensive to build or rent. Some growers sell edible fish as "whole pondside," which complies with current state and federal regulations and simplifies marketing. However, the buyer must have an Aquaculture Certificate to possess restricted non-native live fish. If the fish are deceased, no permit is required. Another option not common in aquaponics, but that could be considered, is to grow ornamental fish like koi or bait fish, which eliminates the food safety considerations and simplifies marketing regulations, provided the fish are not shipped out of state. Fruits and vegetables harvested from the plant (considered first cut) do not require special food-processing facilities unless they are cut again before packing, in which case they are considered processed food subject to FSMA's Preventive Controls for Human Foods rule.
Food safety requirements for fruits and vegetables also are driven by the retailer/restaurant owners making the purchase. The large box stores and chain restaurants almost always require third-party food safety audits of farms. Very few aquaponic farms have passed food-safety audits because many auditors equate fish effluent with raw manure from warm-blooded mammals, which is not allowed in fruit or vegetable production. The body temperature of cold-blooded animals like fish is considered too low for optimal proliferation of most bacteria likely to infect humans (Fox et al. 2012). However, the potential for transient contamination by animals like mice or birds (feces containing enterotoxigenic E. coli) or amphibians and reptiles like frogs and lizards (Salmonella spp.) requires that animals should be excluded from the production area and any plants soiled with fecal droppings be removed and culled. As an additional precaution, the edible portions of harvested aquaponic fruits and vegetables should not come in contact with the recirculating fish effluent.
Elevated recirculating aquaponic systems using potable water, especially in conjunction with some type of protected agriculture structure, are much less likely to come in contact with pathogens that cause food-borne illnesses compared to open-field soil production of fruits and vegetables. A variance to the "auto-failure" third-party audit rule for raw manure should be established for aquaponic-system fish effluent so that aquaponic farmers can pass food safety audits and access the same markets enjoyed by other farmers (Fox et al. 2012). Currently, aquaponic farms can sell at local farmers' markets, mom & pop grocery stores and restaurants, or on-farm sales, which do not require the audits. Since vegetables usually account for the majority of income on aquaponic farms, this food safety marketing limitation slows the adoption of this sustainable agricultural production system. It still is advisable for all farms to follow recommended GAPs (Good Agricultural Practices), procedures that can be noted and used for marketing purposes (Schneider et al. 2011).
Benefits of Decoupling Aquaponic Systems
Some aquaponic producers have been able to pass food safety audits by using UV sterilization of the fish effluent prior to it entering the hydroponic system. Another design option which is gaining popularity is to decouple the return flow, disconnecting the continuous recirculating system, so that each fish and plant production unit can be operated independently. The amount of a one-way flow from the aquaculture tank to a hydroponic storage tank is based on the quantity of water taken up by the plants and evaporation from the systems (evapotranspiration). The storage tank can then be treated to optimize water quality, nutrient balance and sanitized with chlorine to remove micro-organisms before being pumped to the root zone of the plants. Under these conditions, two storage tanks may be necessary, one to receive the effluent, while the other already treated tank is injecting into the hydroponic system. Other advantages include:
-
The water quality and temperature for the fish can be optimized for that species without regard to its effect on plant growth.
-
Optimizing the water quality for each species will result in higher fish and plant yields.
-
Treating the holding tank to eliminate micro-organisms should allow passage of food safety audits and expands hydroponic design options to include micro-irrigation systems.
-
One production unit (fish or plants) can be shut down for repairs, maintenance, or growing season restrictions, while the other can continue to operate independently.
For optimum plant yields, decoupling will most likely require the use of a hydroponic fertilizer at the following rates, ¼ strength (lettuce) to ½ strength (tomato) since the lack of recirculation will reduce overall nutrient buildup from fish effluent. Other expenses of decoupling could be the addition of two hydroponic holding tanks and a solids/nitrifying bacteria bio-filter for the aquaculture system. The increased plant and fish yields and the convenience of operating the systems independently could offset the additional costs.
Decoupling opens up many advantages that would help overcome barriers to adoption of aquaponics, making it more attractive to existing large scale hydroponic farmers. If this occurs, it will help to mainstream adoption of this sustainable food production system.
Summary
Aquaponics is an intensive production system producing multiple crops with reduced water and fertilizer inputs and is highly suited for small farm producers targeting local markets and agritourism opportunities. Aquaponic systems are more complex than plant- or fish-only systems because the optimal conditions for each group of organisms—plants, fish, and the nitrifying bacteria—are not exactly the same. Like many new technologies, it will be some time before firm recommendations for every aquaponic situation (type of hydroponic subsystem x type of plant x type of fish x conventional vs. organic production, etc.) are tested (Table 5) and put into readily available production guides. It is recommended to start small, produce only what you can sell, and grow with the system and markets, learning as you go.
Resources
Pentair Aquatic Eco-systems, Apopka, FL
Crop King, Inc., Lodi, OH
Nelson and Pade, Inc. aquaponics.com
University of Florida link: sfyl.ifas.ufl.edu
Small farm link: smallfarm.ifas.ufl.edu
Literature Cited
Adler, P. R., J. K. Harper, F. Takeda, E. D. Wade, and S. T. Summerfelt. 2000. "Economic evaluation of hydroponics and other treatment options for phosphorus removal in aquaculture effluent." HortScience 35: 993–999.
Chapman, R. 2012. Culture of hybrid tilapia: a reference profile. CIR1015. Gainesville: University of Florida Institute of Food and Agricultural Sciences. edis.ifas.ufl.edu/fa012
Fox, B. K., C. S. Tamaru, J. Hollyer, L. F. Castro, J. M. Fonseca, M. Jay-Russell and T. Low. 2012. "A preliminary study of microbial water quality related to food safety in recirculating aquaponic fish and vegetable production systems." College of Tropical Agriculture and Human Resources Document. FST-51. www.ctahr.hawaii.edu/oc/freepubs/pdf/FST-51.pdf
Francis-Floyd, R., C. Watson, D. Petty and D. Pouder. 2012. Ammonia in aquatic systems. TA16. Gainesville: University of Florida Institute of Food and Agricultural Sciences. edis.ifas.ufl.edu/fa031
Goodman, E. R. 2011. "Aquaponics: Community economic development." Dept. of Urban Studies and Planning Thesis, Massachusetts Institute of Technology, Cambridge, MA. dspace.mit.edu/handle/1721.1/67227
Hill, J. 2013. Regulations pertaining to non-native fish in Florida aquaculture. FA-121. Gainesville: University of Florida Institute of Food and Agricultural Sciences. https://edis.ifas.ufl.edu/publication/fa121
Hochmuth, G. and R. Hochmuth. 2012. Nutrient solution formulation for hydroponic (perlite, rockwool, NFT) tomatoes in Florida. HS-796. Gainesville: University of Florida Institute of Food and Agricultural Sciences. edis.ifas.ufl.edu/cv216
Knickerbocker, K. 2013. "Species used in aquaponics: Rules and regs." Florida Department of Agriculture Aquaculture Division. fdacs.gov/content/download/64591/file/FDACS-P-01770-speciesusedincommercialaquaculture
Lennard, W. A. and B. V. Leonard. 2006. "A comparison of three different hydroponic sub-systems (gravel bed, floating and nutrient film technique) in an aquaponic test system." Aquaculture Intl. 14: 539–550.
Lim, C. and C. D. Webster. 2006. Tilapia: Biology, culture, and nutrition. Binghamton, NY: The Food Products Press.
McMurtry, M. R., P. V. Nelson, D. C. Sanders, and L. Hodges. 1990. "Sand culture of vegetables using recirculated aquacultural effluents." Appl. Agr. Res. 5: 280–284.
Rakocy, J. E., D. S. Bailey, K. A. Shultz, and W. M. Cole. 1997. "Evaluation of a commercial-scale aquaponic unit for the production of tilapia and lettuce." 4th Intl. Symp. on Tilapia in Aquaculture 1: 357–372.
Rakocy, J. E., D. S. Bailey, R. C. Schultz, and E. S. Thoman. 2004. "Update on tilapia and vegetable production in the UVI aquaponic system." 676–690. In New Dimensions on Farmed Tilapia: Proc. 6th Intern. Symp. on Tilapia in Aquaculture. Manila, Philippines. ag.arizona.edu/azaqua/ista/ista6/ista6web/pdf/676.pdf
Rackocy, J. 2011. Aquaponics Q and A. Montello, WI: Nelson and Pade, Inc.
Resh, H. M. 2013. Hydroponic Food Production. Boca Raton, FL: CRC Press.
Schneider, R., K. Schneider, and D. Archer. 2011. Food safety on the farm—An overview of good agricultural practices. FSHN06-01. Gainesville: University of Florida Institute of Food and Agricultural Sciences. edis.ifas.ufl.edu/fs135
Timmons, M., J. Ebeling, F. Wheaton, S. Summerfelt, and B.Vinci. 2002. Recirculating aquaculture systems, 2nd edition. North East Reg. Aquaculture Ctr. Pub. No. 01–002.
Tyson, R. V., E. H. Simonne, D. D. Treadwell, J. M. White, and A. Simonne. 2008. "Reconciling pH for ammonia biofiltration and cucumber yield in a recirculating aquaponic system with perlite biofilters." HortScience 43: 719–724. journals.ashs.org/hortsci/view/journals/hortsci/43/3/article-p719.xml
Tyson, R., R. Hochmuth, and D. Cantliffe. 2013. Hydroponic vegetable production in Florida. HS405. Gainesville: University of Florida Institute of Food and Agricultural Sciences. edis.ifas.ufl.edu/pdffiles/HS/HS40500.pdf
USDA/ARS. 2004. The commercial storage of fruits, vegetables, and florist and nursery stocks. United States Department of Agriculture, Agricultural Research Service Handbook No. 66. ars.usda.gov/ARSuserfiles/oc/np/commercialstorage/commercialstorage.pdf
Yanong, R. 2012. Fish health management considerations in recirculating aquaculture systems, Part 1: Introduction and general principles. CIR120. Gainesville: University of Florida Institute of Food and Agricultural Sciences. edis.ifas.ufl.edu/fa099
Tables
Overview of the roles and factors that affect the growth of the major organisms present in aquaponic systems.