Introduction
The terms alkalinity and hardness are often used interchangeably when discussing water quality. These aggregate properties of water share some similarities but are distinctly different. The objectives of this document are to provide readers with 1) an overview of alkalinity and hardness, 2) an understanding of the importance of alkalinity and hardness from management and ecological perspectives, 3) a summary of methods for measuring each, and 4) the current state regulations for each in public waters of Florida.
Descriptions
Alkalinity
Alkalinity is a measure of the acid-neutralizing capacity of water. It is an aggregate measure of the sum of all titratable bases in the sample. Alkalinity in most natural waters is due to the presence of carbonate (CO3=), bicarbonate (HCO3-), and hydroxyl (OH-) anions. However, borates, phosphates, silicates, and other bases also contribute to alkalinity if present. This property is important when determining the suitability of water for irrigation and/or mixing some pesticides and when interpreting and controlling wastewater treatment processes. Alkalinity is usually reported as equivalents of calcium carbonate (CaCO3).
Hardness
Hardness is most commonly associated with the ability of water to precipitate soap. As hardness increases, more soap is needed to achieve the same level of cleaning due to the interactions of the hardness ions with the soap. Chemically, hardness is often defined as the sum of polyvalent cation concentrations dissolved in the water. The most common polyvalent cations in fresh water are calcium (Ca++) and magnesium (Mg++).
Hardness is usually divided into two categories: carbonate hardness and noncarbonate hardness. Carbonate hardness is usually due to the presence of bicarbonate [Ca(HCO3)2 and Mg(HCO3)2] and carbonate (CaCO3 and MgCO3) salts. Noncarbonate hardness is contributed by salts such as calcium chloride (CaCl2), magnesium sulfate (MgSO4), and magnesium chloride (MgCl2). Total hardness equals the sum of carbonate and noncarbonate hardness. In addition to Ca++ and Mg++, iron (Fe++), strontium (Sr++), and manganese (Mn++) may also contribute to hardness (APHA et al. 1998). However, the contribution of these ions is usually negligible.
Hardness is usually reported as equivalents of calcium carbonate (CaCO3) and is generally classified as soft, moderately hard, hard, and very hard. It is best to report results as the actual equivalents of CaCO3 because the inclusive classification limits for each category may differ between users of the information. The classification scheme used by the US Environmental Protection Agency (EPA) is shown in Table 1.
Sources of Alkalinity and Hardness
Water alkalinity and hardness are primarily a function of 1) the geology of the area where the surface or groundwater is located and 2) the dissolution of carbon dioxide (CO2) from the atmosphere. The ions responsible for alkalinity and hardness originate from the dissolution of geological minerals into rain and groundwater. Rainwater is naturally acidic, which tends to solubilize some minerals more easily. Surface and groundwater sources in areas with limestone formations are especially likely to have high hardness and alkalinity due to the dissolution of bicarbonates and carbonates.
The interaction of CO2 with the dissolved minerals is described by the carbonate system. The carbonate system describes a series of chemical equilibria with CO2(g) in the atmosphere and various bicarbonates and carbonates dissolved from surrounding mineral deposits. CO2(g) readily dissolves in water (Equation 1). The dissolved CO2(aq) reacts with water molecules to form carbonic acid (H2CO3*), which is very unstable and quickly dissociates, yielding H+ and a bicarbonate ion (HCO3-) (Equations 2 and 3). At pH = 6.3, the amount of CO2 dissolved in water equals the amount of bicarbonate ion (HCO3-). Dissolved CO2 is dominant when pH < 6.3. At higher pH, the HCO3- dissociates to yield H+ and a carbonate ion (CO3=) (Equation 4).
CO2(g)?CO2(aq) Eq. 1
CO2(aq) + H2O?H2CO3* Eq. 2
H2CO3*?H+ + HCO3- pKa= 6.3 Eq. 3
HCO3-?H++ CO3= pKa= 10.3 Eq. 4
At pH 10.3, the bicarbonate ion concentration equals the carbonate ion concentration. CO3= is dominant at pH > 10.3, and HCO3- dominates between pH 6.3 and 10.3. The pH of most natural waters falls in the 6 to 9 range because of the bicarbonate buffering.
Alkalinity and Hardness Relationship
Alkalinity and hardness are related through common ions formed in aquatic systems. Specifically, the counter-ions associated with the bicarbonate and carbonate fraction of alkalinity are the principal cations responsible for hardness (usually Ca++ and Mg++) (Equations 3 and 4). As a result, the carbonate fraction of hardness (expressed as CaCO3 equivalents) is chemically equivalent to the bicarbonates of alkalinity present in water (Burton Jr. and Pitt 2002) in areas where the water interacts with limestone (Timmons et al. 2002). Any hardness greater than the alkalinity represents noncarbonate hardness.
Ecological/Management Significance
Alkalinity
Alkalinity is important because it buffers the pH of water within the system. Without this buffering capacity, small additions of acids or bases would result in significant changes of pH, which could be deleterious for aquatic life. Alkalinity also influences the distribution of some organisms within aquatic systems. For example, most freshwater amphipod species are usually limited to low-medium carbonate waters, whereas some species such as Gammarus lacustris may be found in high carbonate waters (Pennak 1989).
The associated carbonates and bicarbonates comprising the majority of alkalinity may also impact the bioavailability and toxicity of several metallic environmental contaminants and pesticides to nontarget and target organisms. For example, the bioavailability of copper-based, non-chelated pesticides generally decreases as alkalinity increases due to copper's reactions with the oppositely charged bicarbonate/carbonate ions and competition of the counter cations for active sites. The efficacy of these copper-based pesticides decreases as bioavailability decreases. For this reason, always consult the pesticide label for specific instructions regarding alkalinity of makeup water for pesticide mixing. Failure to follow the recommendations may result in significant waste of material and personnel resources, with no resulting pest control benefits.
Hardness
Calcium and magnesium are needed to support calcification of larval skeletal structures and to support newly fertilized freshwater fish eggs (Timmons et al. 2002). Additionally, hardness has been associated with mitigation of the toxicity of some metals to gill-breathing organisms. In fact, the Florida Department of Environmental Protection (FDEP) state water quality criteria for copper in Class I and III freshwater* is based on water hardness using the following relationship (Equation 5):
Cu = e(0.8545[ln(Hardness)]-1.702) Eq. 5
The mitigating effects are likely due to the individual polyvalent cations (e.g., Mg++, Ca++) causing hardness as opposed to hardness itself (Burton Jr. and Pitt 2002). Some of the mitigating effects may be due to the formation of less bioavailable metallic hydroxides and carbonates by associated increases in alkalinity or due to competition of the polyvalent hardness ions for active sites on/in the organism (antagonistic effects).
Hardness can also affect the utility of water for industrial purposes. Hard water is often the source of scale formed in hot water heaters and industrial systems where water is heated. This scale results from the precipitation of calcium carbonate, which becomes less water soluble as the temperature increases (Snoeyink and Jenkins 1980). In these situations, water is usually softened by precipitating the CaCO3 or by using ion exchange softening methods.
*(Florida recognizes five designated uses for public water resources: Class I is water used for drinking, Class II is water used to produce shellfish, Class III is water used for recreation (e.g., swimming) and aquatic life support, Class IV is water used for agriculture, and Class V is water used for navigation, utility, and industrial purposes. Each type of water use has specific quality standards that determine if the designated use is being maintained.)
Measurement
Following is a brief description of measurement methods for alkalinity and hardness. Refer to the original source-methods for more details regarding actual procedures (APHA et al. 1998; USEPA 1978ab).
Alkalinity
Alkalinity measurements are based on titration of a water sample to a designated pH using diluted sulfuric acid (0.1N or 0.02N H2SO4) as a titrant and a pH meter for measuring the pH (Figure 1). One mL of 0.1N H2SO4 is equivalent to 5 mg of CaCO3, and 1 mL of 0.02N H2SO4 is equivalent to 1.00 mg CaCO3. The amount of alkalinity due to hydroxides, carbonates, and bicarbonates can be determined by measuring the phenolphthalein alkalinity and total alkalinity. Phenolphthalein alkalinity is measured by titrating to pH 8.3. The endpoint for total alkalinity varies depending on the suspected alkalinity range and the suspected presence of silicates and phosphates. For alkalinity values up to 30 mg/L as CaCO3, an endpoint pH of 4.9 is recommended (APHA et al. 1998). Endpoint pH values of 4.6 and 4.3 are recommended for alkalinities from 30 to 150 and 150 to 500 mg/L as CaCO3, respectively (APHA et al. 1998). An endpoint pH of 4.5 is recommended if silicate and/or phosphate presence is suspected or known (APHA et al. 1998).
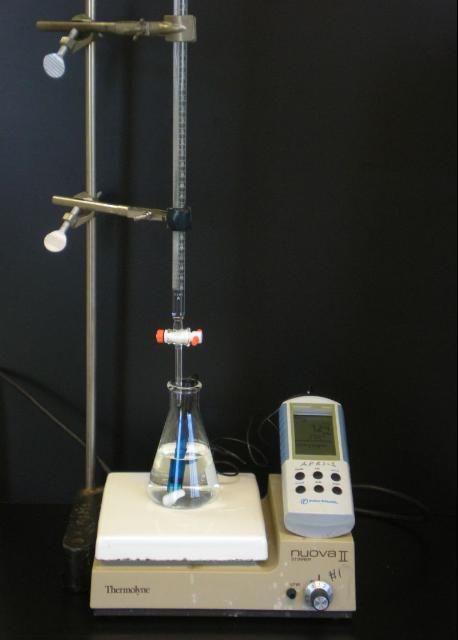
If a pH meter is not available, color indicators can be used to indicate when the desired pH is reached (Figure 2). In this case, phenolphthalein is used to indicate when the 8.3 pH is reached, changing from colored (pink-fuchsia) at pH > 8.3 to colorless below pH 8.3. For the total alkalinity endpoint, methyl orange or methyl purple dyes may be used. Methyl orange is orange at pH > 4.4 and changes to red at pH 3.1. Methyl purple is green above pH 5.4 and changes to purple at pH 4.8. Use of the dyes is not as accurate as use of a pH meter, as indicated by the color change ranges. This method also requires more experience in recognizing onset of subtle color changes.
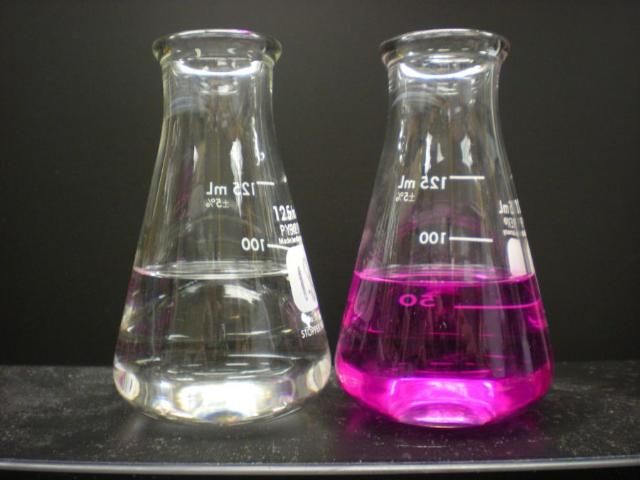
Calculation
Total alkalinity can be calculated using two different equations (Equations 6 and 7). These include:

where,
A = mL of standard acid used to titrate the sample
N = normality of the standard acid
Or

where,
A = mL of standard acid used to titrate the sample
t = titer of the standard acid (mg CaCO3/1.00 mL titrant)
Tips for Measurement
- Calibrate pH meter using manufacturer's instructions. Calibration standards should bracket the selected pH endpoint.
- Allow enough time between titrant additions for the pH electrode to stabilize.
- Reduce the volume of titrant added as the endpoint pH is approached.
- Measure at room temperature.
- Soaps, oily matter, suspended solids, or precipitates may affect the responsiveness of the pH electrode.
- Clean the pH electrode between samples.
- See EDIS publication Water Quality Notes: pH (https://edis.ifas.ufl.edu/ss538) for more information.
Interpretation
The contribution of bicarbonates, carbonates, and hydroxides to total alkalinity may be calculated using the relationships in Table 2 for phenolphthalein (P) and total (T) alkalinity (APHA et al. 1998).
Hardness
Hardness can be measured by titration or by quantification of individual ion concentrations (Ca++ and Mg++) contributing to hardness. Quantification of individual ions is the technique preferred by APHA et al. (1998). Brief descriptions of the two methods are included below.
Titration
The titration method is based on a colorimetric reaction that occurs when all hardness ions have been removed from the solution. Initially, the pH of the sample is adjusted to >10 using an ammonium hydroxide-ammonium chloride buffer. Next, an indicator solution (Eriochrome Black T or calmagite) is added. At pH 10 in the presence of Ca++ and Mg++, the indicator dye will turn the sample a red wine color. Next, 0.01M ethylenediaminetetraacetic acid (EDTA) is titrated into the sample until a color change occurs. The EDTA effectively removes the free Ca++ and Mg++ ions from solution. When all of the Ca++ and Mg++ have been removed from solution, the dye will change from wine-red to blue. The volume of EDTA titrant used to achieve the endpoint color change is used to calculate hardness using Equation 8.

where,
A = mL EDTA titrant used to reach the endpoint color change for the sample
B = mg CaCO3 equivalent to 1.00 mL EDTA titrant
Tips for Measurement
- The entire titration process should be completed no more than 5 minutes after initially adding the buffer.
- Perform titrations at room temperature (sample and titrant).
- Some metal ions can cause fading or indistinct endpoints. These can be reduced using various inhibitors. Consult APHA et al. (1998) for details.
- Organic matter (suspended or colloidal) may interfere with the endpoint. This problem can be overcome by drying the sample, combusting the organic materials, and redissolving the inorganic constituents before titration.
Hardness by Calculation
Using the calculation technique, separate determinations of calcium and magnesium are made using an appropriate analytical technique. Hardness is calculated using Equation 9. The major disadvantage of this method is the need for sophisticated equipment and the possibility of ignoring other hardness-contributing minerals in unusual conditions.
Hardness (as mg CaCO3/L) = 2.497•[Ca, mg/L] + 4.118•[Mg, mg/L] Eq. 9
Florida Surface Water Quality Criteria
FDEP is charged with protecting the quality of state-owned surface water bodies with respect to their designated uses. While no water hardness criteria are specified, metals criteria are based on water hardness. No alkalinity criteria are specified for Class II: shellfish propagation or harvesting and Class III-marine waters*. Alkalinity should not be depressed below 20 mg/L as CaCO3 for Class I and III-freshwaters and should be = 600 mg/L as CaCO3 for agricultural water supplies. Standards can be found at: https://www.flrules.org/gateway/RuleNo.asp?title=SURFACE%20WATER%20QUALITY%20STANDARDS&ID=62-302.530.
References and Suggested Readings
American Public Health Association (APHA), American Water Works Association (AWWA), and the Water Environmental Federation (WEF). 1998. Standard Methods for Examinations of Water and Wastewater, 20th ed. United Book Press, Inc. Baltimore, Maryland.
Burton, G. A., Jr., and R. E. Pitt. 2002. Storm Effects Handbook: A Toolbox for Watershed Managers, Scientists, and Engineers. Lewis Publishers, Boca Raton, FL.
Pennak, R.W. 1989. Fresh-Water Invertebrates of the United States, 3rd ed. John Wiley & Sons, New York.
Snoeyink, V. L. and D. Jenkins. 1980. Water Chemistry. John Wiley & Sons, New York. P. 220.
Timmons, M. B., J. M. Ebeling, F. W. Wheaton, S. T. Summerfelt, and B. J. Vinci. 2002. Recirculating Aquaculture Systems, 2nd ed. CAYUGA AQUA VENTURES, Ithaca, N.Y.
USEPA. 1978a. EPA Method #: 310.1: Alkalinity (Titrimetric, pH 4.5). Methods for the Chemical Analysis of Water and Wastes (MCAWW) (EPA/600/4-79/020).
USEPA. 1978b. EPA Method #: 130.2: Hardness, Total (mg/L as CaCO) (Titrimetric, EDTA). Methods for the Chemical Analysis of Water and Wastes (MCAWW) (EPA/600/4-79/020).
Water hardness classifications (reported as CaCO3 equivalents) used by the US EPA (EPA 1986).