Farmers need soil-testing procedures to assess soils for potential plant-available nutrients. Soil testing is the foremost best management practice (BMP). It helps farmers achieve profitable crops while protecting the environment from excessive fertilization and nutrient losses. This publication describes the important steps required to test soil for potential plant-available nutrients. This information will be useful to county UF/IFAS Extension agents when training farmers and crop consultants about proper soil testing and nutrient management.
Scientists generally accept 17 elements as essential for plant growth (Barker and Pilbeam 2007). These elements are carbon (C), hydrogen (H), oxygen (O), phosphorous (P), potassium (K), nitrogen (N), sulfur (S), calcium (Ca), magnesium (Mg), iron (Fe), boron (B), manganese (Mn), copper (Cu), zinc (Zn), molybdenum (Mo), nickel (Ni), and chlorine (Cl). A certain amount of each of these nutrients—the crop nutrient requirement (CNR)—is critical for crops to complete their life cycles and to produce an optimal yield. Carbon and oxygen are supplied from air, and hydrogen from water. The remaining nutrients can be supplied from the soil; however, the soil may not always contain enough of these nutrients for optimal crop production. Farmers need to know the portion of the CNR that can be supplied from the soil, because these nutrients are essentially free to the farmer. If the CNR cannot be supplied entirely from the soil, then the soil-supplied nutrients can be augmented with fertilizers or other nutrient sources such as manures or composts. Nearly 150 years ago, scientists developed chemical tests to assess the concentrations of plant-available nutrients in a soil sample and then to use that assessment to make recommendations for supplemental fertilizer.
What is soil testing?
The Soil Science Society of America defines soil testing as "the application of soil science research to the rapid chemical analyses to assess the available nutrient status of a soil." Agronomic soil tests do not measure the total amount of a plant nutrient in the soil, or even the exact amount of plant-available nutrient for the season. Soil tests provide an index (i.e., indication, or assessment) of the nutrient-supplying capacity of the soil (see "Soil text index" section below). Soil testing is most applicable to nutrients of low mobility in soils—such as P, K, Mg, Ca, and micronutrients—because these nutrients will remain in the soil after the soil has been tested. This low mobility is in contrast to mobile soil nutrients—such as nitrogen—that may rapidly transform or leach from the soil in the time between soil testing and crop planting.
Why do we use soil testing?
We test soil to determine how to get the best crop yields and how to use fertilizer and other nutrient sources most efficiently. When soil testing was originally developed, the goal was to enhance crop yields by identifying productive soils. Today, crop productivity is still a goal, but another goal is to avoid excessive fertilizer applications and, thereby, protect the environment.
The soil test is a process that includes the following five activities: (1) collecting the soil sample, (2) processing the soil sample in the lab, (3) analyzing the sample for its extractable nutrient content, (4) interpreting the results of the analysis, and (5) using the information to make a fertilizer recommendation (Sikora and Moore 2014). Activities 1 through 4 are discussed in this publication, and activity 5 is discussed in the EDIS publication SS623, Fertilizer Recommendation Philosophies (Hochmuth et al. 2014).
Collecting the soil sample
The usefulness of the soil-testing process depends on the quality of the soil sample. A quality soil sample is representative of the soil for the field in question, and a quality sample is collected properly, in terms of depth and numbers of subsamples.
Depth
Soil samples for predicting fertilizer needs are collected from the top six inches of soil in the field, because the top six inches is the part of the soil typically tilled with plows and disks and the upper six-inch layer of soil also contains a large portion of the nutrient-absorbing roots.
Number of subsamples
Before sampling, the field should be divided into "management units," which are representative of areas that will receive different cultural practices, such as different crops or different planting dates (Figure 1). Management units may also represent soil types with different native mineral composition. (Current management units may be different from previous cropping-system-management units and may also have different nutrient content.) Your different management units should be sampled separately, because they may require different approaches to fertilization. A large field may have enough inherent variability to justify determining individual management units of 20 to 40 acres. To take a soil sample from a management unit, first collect 20 subsamples with a soil sampling probe, and then composite the subsamples in a plastic bucket and mix them. Take a sample volume of about a half-pint from the bucket of mixed soil and submit it to the lab in the paper bag provided for soil-testing submissions. Additional information on management units and soil sampling schemes can be found in the EDIS document SS402, UF/IFAS Nutrient Management Series: Soil Sampling Strategies for Precision Agriculture (Mylavarapu and Lee 2014).
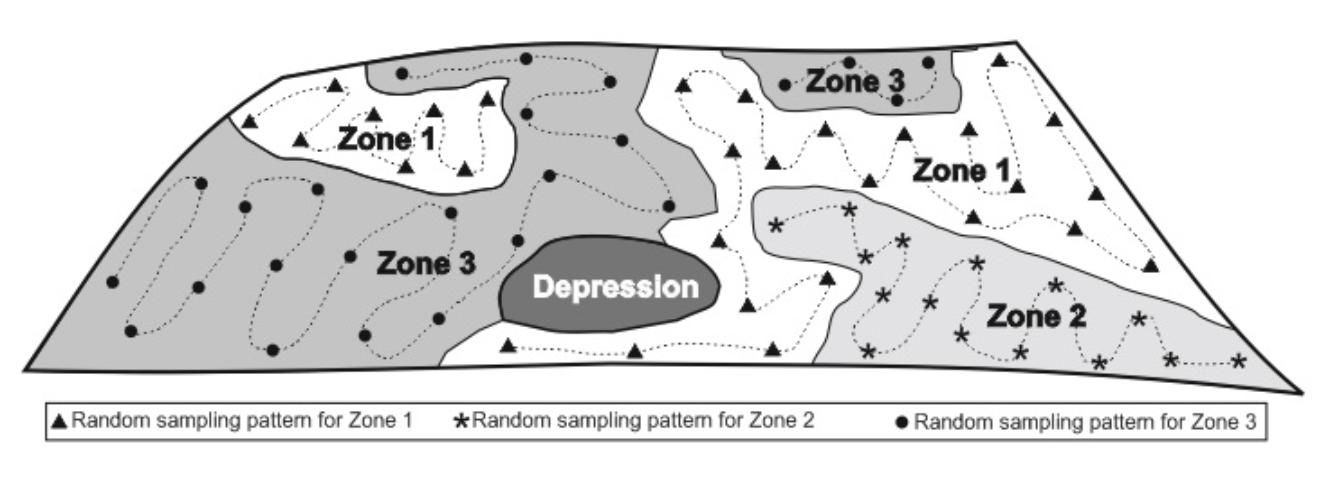
Credit: Greg Means, UF/IFAS
Additional information needed
In addition to the soil sample, the lab will require you to fill out some forms to provide information about the crop to be grown and the specific nutrient analyses being requested. This information will help the lab make the best fertilizer recommendation for the farmer.
Soil sampling and precision agriculture
Typically, soil testing and fertilizer recommendations are made for the entire management unit, even though there may be considerable variation across the management unit, which may be 20 acres in size. However, some growers are adopting precision agricultural techniques. For example, some farmers are applying fertilizers in precise techniques where the fertilizer rate is varied throughout a field according to the nutrient levels in the soil. Precision agriculture uses variable-rate application of fertilizers so that areas in the field needing more or less fertilizer can receive the appropriate rate. For variable-rate application to work, soil samples need to be taken on a more detailed basis. One way to take more detailed soil samples is to use a grid-sampling approach. Grids may be as small as two acres each. Other techniques for variable-rate application of fertilizers have been based on changes in soil type as described by Natural Resources Conservation Service (NRCS) soil maps, yield maps derived from previous crop yields, and various combinations of these and other techniques (Mylavarapu and Lee 2014).
Processing and analyzing the soil sample
When the sample arrives at the laboratory, most labs analyzing agricultural soils use the following steps:
-
The soil is dried at approximately 100oF to remove soil moisture.
-
The soil is sieved to remove old plant parts and stones. A clay-dominated soil may need pulverizing to break up clods.
-
A small portion of the sample is taken for processing in the lab.
-
The soil sample is mixed (usually by shaking) with a solution called an "extractant."
-
After mixing, the sample's liquid portion is filtered and analyzed for its nutrient content. Analytical equipment will vary, depending on the nutrients being determined and the individual lab design and setup.
-
The concentration of extracted nutrient from the liquid portion is converted to the dried-soil basis and is referred to as the soil-test index.
-
The index is then given an interpretation as to the ability of that soil to provide enough of a nutrient for optimal crop yield. For example, a low interpretation means that the soil cannot supply all of a particular nutrient for crop production. A high interpretation, however, means that the soil can supply all of a particular nutrient for crop production.
-
The final step is for the lab to make a fertilizer recommendation for those soil samples that received interpretations of less than high. The fertilizer recommendation provides the recommended rate, but the rate is not the only part of a recommendation. A complete recommendation also contains guidelines about placement and timing of the fertilizer application, which can help farmers use fertilizer efficiently while also protecting the environment.
The role of soil test extractants
The extractant, a solution that is mixed with the soil sample, is crucial to the soil test. Briefly, the extractant is developed for specific types of soils and growing conditions, such as soil reaction (pH) and the need for micronutrient results. The extractant is often a solution of various chemicals including water, acids, and certain organic chemicals. For example, the UF/IFAS Extension Soil Testing Lab now uses the Mehlich-3 soil test extractant—which is composed of acetic acid, ammonium nitrate, nitric acid, ammonium fluoride, and ethylene diamine tetra acetic acid (EDTA). There are at least a dozen soil test extractants in common usage by agricultural soil testing labs in the United States, but not all extractants are useful for all agricultural regions. Each extractant was developed to meet particular goals, but some extractants were developed to have wide applicability among soil types and tested nutrients. These latter extractants are called universal extractants, and Mehlich-3 is one such extractant. The Mehlich-3 extractant is more applicable than Mehlich-1 (used by UF/IFAS Extension until August 2013) for Florida's high-pH agricultural soils (Mylavarapu et al. 2014).
Interpreting the results of the soil test index
As mentioned earlier, the concentration of nutrients extracted from the soil sample is called an index. The soil test index is an indication of the soil's nutrient-supplying capacity and its expected relative yield (Table 1). The total amount of a nutrient in the soil is of little importance in determining fertilizer recommendations, because only a portion of a nutrient may be available for plant use during the growing season. For example, a soil's nutrient availability includes a myriad of chemical reactions that a nutrient may undergo with time, and a nutrient may reside in multiple forms (some insoluble). Therefore, the soil test index is often referred to as an availability index. The availability index tells us, based on previous research, the relative level of a nutrient that will probably contribute to the crop nutrient requirement during the growing season.
The extractant used by a lab must be correlated with crop response (Mitchell and Mylavarapu 2014). This correlation means that if the extracting process results in a low interpretation, then that unfertilized soil will produce a low-yield crop. If the extracting process results in a high interpretation, then the unfertilized soil will produce a high-yield crop. Further, the extractant must be calibrated, which means that the lab using the extractant can accurately associate a fertilizer recommendation with each soil test result interpretation. The greatest amount of fertilizer will be recommended for low-testing soil, less for medium-testing soils, and likely no fertilizer for high-testing soils.
Sometimes farmers send a portion of the same sample to several labs and question why the soil test indexes are different among labs. The use of different extractants probably explains the difference. There must be considerable soil testing and crop response research conducted to develop the soil test. Farmers should ask the lab about the particular soil test extractant and its research base. We will discuss correlation and calibration in more detail in EDIS publication SS622, How a Soil Test Is Developed—Correlation and Calibration (Hochmuth, Mylavarapu, and Hanlon 2014).
Important guidance about the soil test index
The soil test index is usually expressed as a nutrient concentration in the air-dry soil. For example, it may be expressed in parts per million (ppm) or milligrams per kilogram (mg/kg). These two expressions are equivalent. The instruments accurately determine the nutrient concentration in the soil using these units of expression.
However, these determinations are occasionally converted into other units for making fertilizer recommendations. In doing this, sometimes an inaccurate and faulty assumption is made—that an acre of six-inch-deep surface soil weighs 2 million pounds. Using that faulty assumption, the concentration value (ppm) is multiplied by 2 to result in the new expression of "pounds per acre." The inaccuracy occurs because soils of different textures and organic-matter contents result in different bulk densities of soils and will, therefore, have differing mass per unit volume.
Another potential fallacy of this particular conversion approach is that the expression "pounds per acre" may be open to misuse in making fertilizer recommendations. Even if the expression "pounds per acre" is employed, it is still an index and must be interpreted as low, medium, or high. The index "lb per acre" cannot be used directly to determine a fertilizer amount by arithmetic.
Example
Let's assume the maximum phosphorus (P2O5) for a crop is 150 lb per acre (this rate would only be recommended on a low index), and further assume that the soil test index was 25 ppm for a submitted soil sample. The index was converted to 50 lb/acre of P by multiplying the concentration index by 2 as explained above. Next, to convert the index from lb/acre P to lb/acre P2O5, the index is multiplied by 2.3 to get 115 lb per acre P2O5. Then, 115 is subtracted from 150 to get 35 lb per acre P2O5, and this rate is used as the fertilizer recommendation.
This series of calculations and assumptions result from a misunderstanding of the soil test index. Using the current IFAS Mehlich-3 interpretation, the index of 25 would be interpreted as low and a recommendation of 150 lb per acre of P2O5 would be recommended, not 35 lb. So, a concentration index should not be converted to a rate value such as lb per acre, because the index is a concentration and must be interpreted before a recommended fertilizer rate can be determined. Conversion of the index in ppm to another unit (such as "pounds per acre") is unnecessary, and it does not matter if the index is in elemental or oxide form, in the case of phosphorus or potassium.
Frequency of soil testing
Soil testing should be a regular, annual process in most cases. However, for high-value crops, soil testing should be carried out on a seasonal basis. Records (see "Soil test and fertilization records" section below) of soil testing results are important to help determine sampling frequency. For example, if several successive years of soil testing show no decline in the index for a particular nutrient, then sampling frequency can be reduced to every two or three years. Unless farmer experience and records indicate otherwise, annual soil testing is recommended in Florida. Buildup of nutrients is less likely to happen in our sandy, low cation-exchange-capacity soils, so annual soil testing will help you avoid planting crops on low nutrient-content soils.
Soil test and fertilization records
Farmers should maintain records of a field's soil test history and fertilization practices. These records will help track fertilizer inputs and can help increase the efficiency of fertilizer use. Records will also help track buildup of certain nutrients that may be detrimental to crop productivity and may have negative environmental impacts. For example, if phosphorus builds up to excessive levels, then loss of soil by erosion could result in phosphorus enrichment of a nearby water body. Or, as another example, leaching may be a problem in some sandy soils of Florida.
Summary
Soil testing is important for determining the portion of the crop nutrient requirement that can be supplied from the soil. Soil testing is most effective in regard to nutrients that are not highly mobile in the soil. Soil testing is an important best management practice. Farmers practicing correlated and calibrated soil testing will benefit from proper fertilizer-rate applications and will protect the environment from nutrient pollution due to inappropriate fertilization practices.
Other publications in this series on soil testing
Hochmuth, George, Rao Mylavarapu, and Ed Hanlon. 2014. Developing a Soil Test Extractant—The Correlation and Calibration Processes. Gainesville: University of Florida Institute of Food and Agricultural Sciences. https://edis.ifas.ufl.edu/ss622.
Hochmuth, George, Rao Mylavarapu, and Ed Hanlon. 2014. Fertilizer Recommendation Philosophies. Gainesville: University of Florida Institute of Food and Agricultural Sciences. https://edis.ifas.ufl.edu/ss623.
Hochmuth, George, Rao Mylavarapu, and Ed Hanlon. 2014. The Four Rs of Fertilizer Management. Gainesville: University of Florida Institute of Food and Agricultural Sciences. https://edis.ifas.ufl.edu/ss624.
References
Barker, A. V., and D. J. Pilbeam. 2007. Handbook of Plant Nutrition. CRC Press, Taylor and Francis Group. Boca Raton, FL.
Mitchell, C. C., and R. Mylavarapu. 2014. Soil Test Correlation and Calibration for Recommendations. In Soil Test Methods from the Southeastern United States. Southern Cooperative Series Bulletin No. 419. USDA-SERA-IEG-6. ISBN 1-58161-419-5.
Mylavarapu, R., and W. D. Lee. 2014. UF/IFAS Nutrient Management Series: Soil Sampling Strategies for Precision Agriculture. Gainesville: University of Florida Institute of Food and Agricultural Sciences. https://edis.ifas.ufl.edu/ss402.
Mylavarapu, R., T. Obreza, K. Morgan, G. Hochmuth, V. Nair, and A. Wright. 2014. Extraction of Soil Nutrients Using Mehlich-3 Reagent for Acid Mineral Soils of Florida. Gainesville: University of Florida Institute of Food and Agricultural Sciences. https://edis.ifas.ufl.edu/ss620.
Sikora, F. J., and K. P. Moore (eds.). 2014. Soil test methods from the southeastern United States. Southern Cooperative Series Bulletin No. 419. ISBN 1-58161-419-5.