Introduction
Both agricultural crops and landscapes are extensively irrigated in Florida, and sprinkler irrigation is widely used for both types of plants. In 1992, approximately 625,000 acres of commercial agricultural crops in Florida were irrigated with sprinklers. This figure represents almost 31 percent of Florida's 2,033,000 irrigated crop acres. In addition, sprinklers are used to irrigate approximately 1 million acres of turf and landscape, including golf courses, commercial landscapes, and home lawns.
Method of Water Distribution
When irrigation is applied by the sprinkler method, water is distributed over the irrigated area by spraying it through the air. Nozzles may be rotated to cover circular or part-circle land areas, or they may be fixed and equipped with deflectors that break up the water stream and deflect it onto the area to be irrigated. High uniformity of water application is achieved by overlapping the spray patterns from adjacent sprinklers.
During sprinkler operation, a stream of water is discharged into the air at high velocity. Friction between the air and the water stream causes the stream to break apart into water droplets that fall to the surface, similar to rainfall. When there is no wind, the sizes of droplets and their distribution patterns primarily depend on the nozzle design and any associated stream breakup mechanisms, the sprinkler's operating pressure, and the height of the sprinkler above the ground surface. Under windy conditions, wind speed and direction also affect droplet sizes and distribution patterns.
Evaporation from Sprinklers
During sprinkler operation, water evaporates from droplets sprayed through the air. The amount of evaporation depends on three factors: (1) the climate demand; (2) the time available for evaporation to occur; and (3) the surface area of the water droplets.
Climate Demand
The climate demand is a measure of the energy available for evaporation and the capacity of the air to store and transmit water vapor. The evaporation process requires 580 calories of energy to convert 1 gram of water from the liquid to the vapor form. This energy must be available from the environment surrounding the sprinkler. Energy is readily available during summer months, as indicated by high air temperatures and high levels of solar radiation. Conversely, low temperatures and low levels of solar radiation during winter months provide less energy for evaporation.
Air humidity is often expressed as relative humidity, which ranges from near 0 to 100 percent, with low values indicating dry air and high values indicating moist air. Since dry air has a greater capacity for moisture, evaporation will occur more rapidly when the air is dry than when it is moist. When the relative humidity is 100 percent, the air is saturated at its current temperature and cannot hold more water; thus, no further evaporation occurs. This condition exists after dewfall at night, during foggy conditions, and during rainfall. Also, since humidity levels are normally high during early morning and early evening hours in humid areas such as Florida, evaporation rates are low during these times on most days.
Wind increases evaporation rates by transporting water vapor away from the evaporating surface. Wind also increases evaporation by transporting warmer or drier air from surrounding areas to displace the moist, cool air above an irrigated surface.
Time Available for Evaporation to Occur
The time available for evaporation to occur is the relatively short time beginning when a water droplet leaves the nozzle and ending when it falls on the ground or vegetated surface. When water is sprayed at greater heights and over greater distances, this opportunity time for evaporation is increased. Thus, more evaporation would be expected to occur from a sprinkler installed on a tall riser than from the same sprinkler (operated at the same pressure so that the drop size distribution is the same) installed on a short riser.
Wind speeds are faster at greater heights above the ground surface, where there are few obstacles to air movement. Thus, evaporation loss from sprinklers mounted on tall risers is also increased because of these higher wind speeds.
Surface Area of Water Droplets
Because evaporation occurs from the surface of the water droplets, the total surface area of the water droplets greatly affects the amount of evaporation loss. For a unit volume of water, the surface area doubles as the droplet diameter decreases by half. For this reason, evaporation rate increases as droplet size decreases if other factors remain constant, and the factors that cause droplet size to decrease will cause evaporation loss to increase.
Wind Drift Loss
Wind drift loss occurs when wind carries water droplets away from the irrigated area. Droplets may either evaporate while they are being transported or may fall outside the irrigated area. In either case, the water is lost because it is not available to the plants being irrigated.
Wind drift loss increases as wind speeds increase and as droplet sizes decrease. At higher wind speeds, larger droplets can be transported by the wind, and droplets can be transported greater distances.
Estimating Sprinkler Evaporation Loss
Many studies of evaporation loss during sprinkler irrigation have been conducted, and some are listed as references to this publication. The most extensive early work was done by Frost and Schwalen (1955, 1960) in Arizona. In their 1960 article, Frost and Schwalen summarized the results of 700 field tests conducted under a variety of climate conditions. They developed a nomograph that enables the user to estimate the percentage of evaporation loss during sprinkler irrigation as a function of sprinkler characteristics, operating pressure, and climate factors. They concluded that evaporation loss could be accurately estimated using three climate factors: air temperature, relative humidity, and wind speed.
The Frost and Schwalen nomograph has been widely used to estimate sprinkler evaporation loss. It has been published in several irrigation textbooks (Pair et al., 1983; Schwab et al., 1981).
Seginer (1971) found strong correlations between sprinkler evaporation loss in Israel and both solar radiation and air temperature. He found weaker correlations with relative humidity and wind speed. He concluded that evaporation loss due to wind drift was negligible for the 3 to 4 mph wind speed conditions of his study.
Myers et al. (1970) conducted wind tunnel tests of sprinkler evaporation loss for typical Florida climate conditions. They stated it is unlikely that evaporation from water droplets in transit from the sprinkler to the ground or plant surface could represent more than 5 percent of the water applied by typical sprinkler irrigation systems under Florida climate conditions.
Evaporation Nomograph
The Frost and Schwalen (1960) nomograph can be used to estimate evaporation loss for specific climate and operating conditions. This nomograph allows the user to estimate the amount of evaporation loss for different times of day or for daytime and nighttime irrigation.
The Frost and Schwalen nomograph is shown in Figure 1. It consists of vertical columns numbered 1 through 9. Data must be entered in columns 1, 2, 5, 7, and 9. The nomograph is used by drawing straight lines that connect the input data to calculate intermediate factors in columns 3, 4, and 8. Finally, a straight line is drawn between columns 4 and 8 to provide an estimate of the percentage of evaporation loss in column 6. The use of the nomograph is illustrated by the following example: Estimate the percentage of evaporation loss from a sprinkler with a 3/16-inch nozzle diameter operated at 40 pounds per square inch (psi) when wind speed is 5 miles per hour (mph), temperature is 90°F, and relative humidity is 10 percent. The sprinkler discharge rate in this example is 6.3 gallons per minute (gpm).
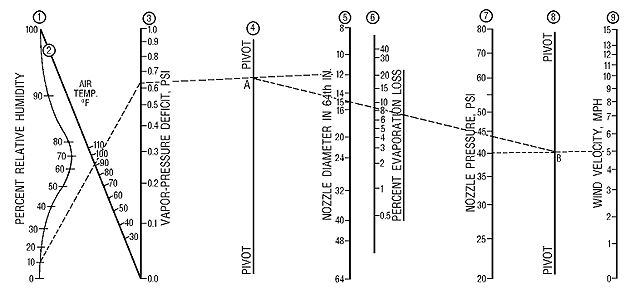
This example represents very extreme climate conditions for a humid area such as Florida. In most cases, the relative humidity would be much higher than 10 percent. Therefore, the normal amount of sprinkler evaporation loss in Florida would be expected to be much smaller than that calculated in this example.
The solution is illustrated by the broken lines in Figure 1. The calculations are done in the following steps:
-
Draw a straight line from the point representing 10 percent relative humidity in column 1 through the mark for 90°F temperature in column. Extend this line to indicate a vapor-pressure deficit of 0.63 psi in column 3.
-
Draw a straight line from the point representing 0.63 psi vapor-pressure deficit in column 3 to the mark that indicates 3/16-inch (12/64-inch) nozzle diameter in column 5. Locate point A where this line intersects the pivot line in column 4. Point A will be used in step 4 below.
-
Draw a straight line from the mark for 40 psi nozzle pressure in column 7 to the point representing 5 mph wind speed in column 9. Locate point B where this line intersects the pivot line in column 8. Point B will be used in step 4 below.
-
Draw a straight line from point A on the pivot line in column 4 to point B on the pivot line in column 8. Read the percentage of evaporation loss where this line intersects column 6. For this example, evaporation loss is 8.5 percent.
Thus, 8.5 percent of the water discharged from an irrigation system using 3/16-inch nozzle diameters and operating at 40 psi would evaporate under these extreme climate conditions. Under typical Florida climate conditions, evaporation loss would not be expected to exceed this value. For the 6.3 gpm sprinkler discharge rate, 8.5 percent, or 0.54 gpm, would be expected to evaporate, while the remaining 5.76 gpm would be expected to reach the soil surface or plant canopy.
Sprinkler Evaporation Loss in Florida
Despite typical high temperatures, there is relatively little sprinkler evaporation loss in Florida because of high humidity levels and low wind speeds. As an example, long-term average climate data for Gainesville, Florida are listed in Table 1 (Whitty et al., 1992).
Long-term daily maximum, average, and minimum daily temperatures are shown for each month and as annual averages. Daily average temperatures range from 56°F in winter to 81°F in summer. Daily maximum temperatures range from 68°F in winter to 92°F in summer. Table 1 also lists long-term minimum and average daily relative humidity values for each month. Maximum values (not shown) are near 100 percent for each month. Relative humidity values do not change greatly throughout the year. Minimum relative humidity values range from 40 percent in April to 53 percent in August. Average relativehumidity values range from 70 percent in April to 77 percent in August.
Long-term daily and daytime average wind speeds also are listed in Table 1. Daytime averages are shown because most evaporation takes place during daylight hours, when wind speeds and temperatures are highest and relative humidity values are lowest. Daytime wind speeds were estimated as being 1.3 times the daily (24-hour) average values. In Gainesville, wind speeds are highest (3.2 mph) during February and March and lowest (1.6 mph) in August.
The combined effects of temperature and relative humidity determine the vapor pressure deficit. The vapor pressure deficit is a measure of the dryness of the air; thus, it is an index of the evaporation rate. Figure 2 shows the general relationships among air temperature, relative humidity, and vapor pressure deficit from the Frost and Schwalen (1960) nomograph. This figure illustrates that the vapor pressure deficit (and evaporation rate) will be highest when temperatures are high and the relative humidity is low. Notice that when temperatures are low, the vapor pressure deficit will be low, despite the humidity level. Also, when the relative humidity is high, the vapor pressure deficit will be low, despite the temperature.
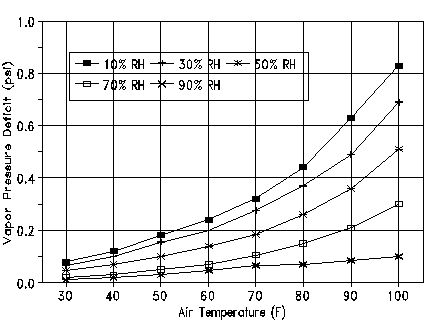
Because high humidity levels are typical in Florida, the vapor pressure deficit is typically low despite the high temperatures. Figure 3 shows monthly vapor pressure deficits for Gainesville. These were calculated from the temperature and relative humidity data in Table 1 using the Frost and Schwalen nomograph (Figure 1). Both maximum and average monthly values were calculated. The maximum values were calculated from the maximum temperature and minimum relative humidity data. These values would be expected to represent peak vapor pressure deficits occurring during the early afternoon, when temperatures are highest and humidities are lowest. The average values were calculated from average temperatures and average relative humidity readings. These values would be expected to represent average daily vapor pressure deficits, the average of both daytime and nighttime conditions.
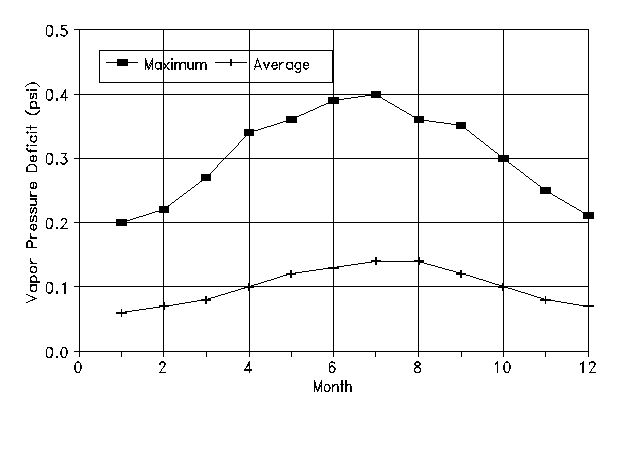
In Gainesville, average daily vapor pressure deficits are low, ranging from 0.06 psi in January to 0.14 psi in July and August. Maximum daily vapor pressure deficits are larger and more variable, ranging from 0.2 psi in January to 0.4 psi in July.
Monthly evaporation percentages for Gainesville were calculated using the vapor pressure deficit data from Figure 3 and the wind speed data from Table 1. A mid-range sprinkler nozzle diameter (5/16-inch) and a mid-range pressure (45 psi) were assumed. Figure 4 shows the monthly distribution of average and maximum daily evaporation percentages. The average daily values were low and uniform at about 1.5 percent throughout the year. The maximum (early afternoon) values were more variable, ranging from 2.1 percent in January and December to 3.1 percent in July.
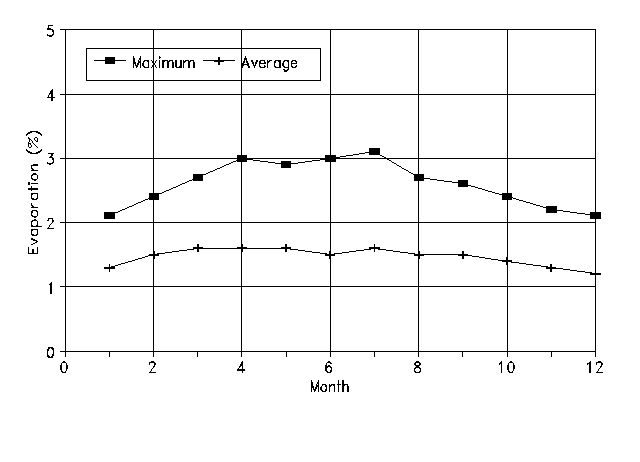
Reducing Evaporation Loss from Sprinklers
Evaporation loss from a sprinkler irrigation system can be reduced by changing the sprinkler operating conditions to increase the sizes of water droplets or by irrigating when climate demands are low.
Evaporation loss will be reduced by changing either or both of the following operating conditions: (1) increasing the nozzle diameter, or (2) decreasing the operating pressure. Both of these changes will increase the proportion of large droplets, thus decreasing the surface area from which evaporation can occur. However, sprinkler users must follow the manufacturer's recommendations when selecting nozzle diameters and operating pressures. Otherwise, poor distribution patterns will occur, reducing the uniformity of water application.
The effect of changing the nozzle diameter is illustrated in Figure 5. Calculations for this example were made using Gainesville climate data for June and sprinklers operated at 45 psi. As shown in Figure 5, the evaporation percentage is large when small nozzles are used. It is small when large nozzles are used and other factors remain constant. Notice that the evaporation percentage is not linearly related to the nozzle diameter. It increases rapidly when nozzle sizes are decreased below 5/16-inch but decreases slowly when nozzle sizes are increased.
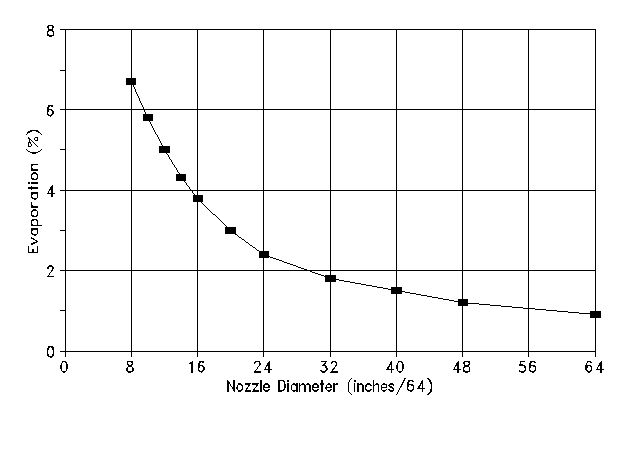
The effect of changing the operating pressure is shown in Figure 6. Calculations for this example were made using Gainesville climate data for June and 5/16-inch nozzle diameters. As Figure 6 illustrates, the evaporation percentage is small when small pressures are used; it is large when large pressures are used and other factors remain constant. Also, the relationship between evaporation percentage and pressure is approximately linear. It increased from 1.4 percent at 20 psi to 5 percent at 80 psi.
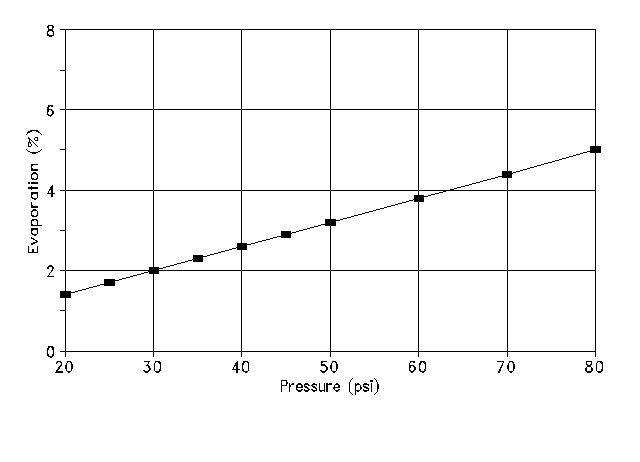
The use of excessively large nozzles or excessively small pressures must be avoided. Excessively large nozzles will increase sprinkler and distribution pipeline flow rates, making the system cost too high. Also, soil infiltration rates may be exceeded, causing runoff. Thus, allowable increases in nozzle sizes will be limited by economic considerations and production system characteristics.
Excessively low pressures will reduce the uniformity of water application and waste water because of low application efficiencies. Sprinkler mechanisms will not operate properly at pressures that are too low. Excessively low pressures also will produce droplet sizes that are too large, creating the potential for crop damage or soil erosion from the impact of the droplets. Thus, allowable reductions in pressures will be limited by sprinkler operating characteristics and the requirement for uniform water applications.
Evaporation loss can be reduced by operating sprinklers only when climate demand is low. This means operating sprinklers when relative humidity is high and air temperature and wind speed are low. In Florida, the relative humidity is very high during nighttime, early morning, and late evening hours on most days. At those times, air temperatures are also low and wind speeds are normally reduced, minimizing sprinkler evaporation loss. Figure 7 illustrates the effects of wind speed on evaporation loss, using Gainesville climate data for June, sprinklers with 5/16-inch nozzle diameters, and operating pressures of 45 psi. For this example, the relationship between evaporation percentage and wind speed ranging from 0 to 15 mph was approximately linear. Evaporation loss increased from 2.1 percent to 8.0 percent as wind speed increased from 0 to 15 mph.
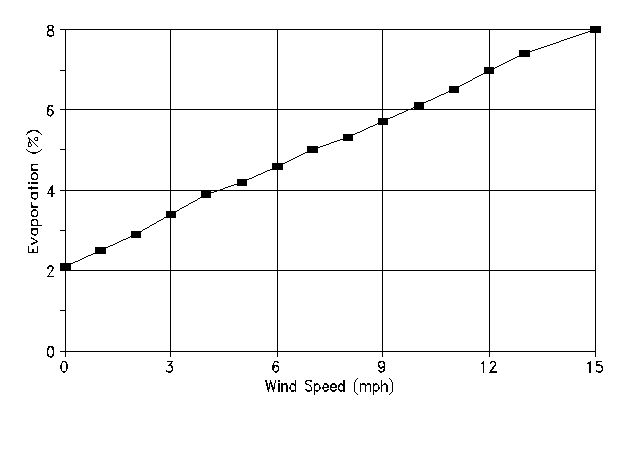
Reducing Wind Drift Loss
Wind drift loss occurs when small water droplets are transported from the irrigated area by wind. This occurs when droplets are small and wind speed is high.
If the wind is blowing when irrigation occurs, some wind drift loss is unavoidable. To avoid excessive wind drift loss, irrigation systems should not be operated when wind speeds are high. Also, a sprinkler should be operated only within the operating pressure range for which it was designed. If operating pressures are too high, too many small droplets will be created and wind drift loss will increase. Most sprinkler companies manufacture nozzles specifically designed to reduce the number of small droplets and minimize wind drift loss.
Increasing Evaporation Loss from Sprinklers
Under some conditions, it may be desirable to increase the amount of evaporation loss from sprinklers. Such increases would be desirable when sprinklers are being used for crop cooling, as is the case during the establishment of transplants, or when water disposal is the objective -- for example, in the design of sprayfields for land applications of wastewater.
Evaporation from sprinklers cools plant and ground surfaces and the surrounding air. Thus, sprinkler irrigation is used to prevent excessive water stress while plant roots (normally, those of young plants or transplants) are developing. It is often required to establish such fruits and vegetables as strawberries and cabbage, and many ornamental plants.
In the design of sprayfields for wastewater disposal, the size of the required sprayfield can be reduced if evaporation loss is increased. Such reductions are possible because some of the water discharged from sprinklers evaporates before it reaches the ground or plant surface.
To increase evaporation loss, small nozzles should be used and sprinklers operated at high pressures and when climate demand is greatest. Climate demand will normally be greatest during the early afternoon, when relative humidity is lowest and air temperature and wind speed are highest.
Excessively high operating pressures should be avoided because both diameter of coverage and water application uniformity will be reduced if the pressure is too high. Also, sprinkler mechanisms may not operate properly at excessively high pressures.
Under windy conditions, the distribution of the very small water droplets created by excessive pressures will be easily distorted by wind. These small droplets may be transported to surrounding areas by wind drift. Under calm conditions, these small droplets will fall nearer the sprinkler, reducing the effective diameter of coverage and possibly reducing the uniformity of water application. Thus, the maximum operating pressure should be limited based on the sprinkler manufacturer's recommendations so that acceptable diameters of coverage and uniformity of water application may be achieved.
Summary
During sprinkler operation, some evaporation occurs from water droplets as they are sprayed through the air. The amount of evaporation depends on (1) the climate demand; (2) the time available for evaporation to occur; and (3) the surface area of the droplets. A nomograph for estimating evaporation loss from sprinklers was presented. For climate conditions typical of Gainesville, Florida, the average daily evaporation loss would be expected to be about 1.5 percent. The average loss would be expected to be about 3 percent for sprinkler operation during early afternoon hours on typical summer days. The amount of loss would be very small for operation during nighttime, early morning, and early evening hours.
Sprinkler evaporation loss can be reduced by changing sprinkler operating conditions to increase water droplet size or by operating the system under conditions of low climate demand. Climate demand is low at night and during early morning and early evening hours.
Evaporation loss can be increased by using small nozzles and operating sprinklers at high pressures to produce small water droplets, and by operating systems when climate demand is greatest. Climate demand will normally be greatest during the early afternoon, when relative humidity is lowest and air temperature and wind speed are highest. Sprinklers should not be operated outside the manufacturer's recommended pressure range because they may perform poorly under such conditions.
References
Myers, J.M., C.D. Baird and R.E. Choate. 1970. Evaporation losses in sprinkler irrigation. Florida Water Resources Research Center Publication No. 12. University of Florida, Gainesville. 41 pp.
Frost, K.R. and H.C. Schwalen. 1960. "Evapotranspiration during sprinkler irrigation." Transactions of the ASAE 3: 18-20, 24.
Frost, K.R. and H.C. Schwalen. 1955. Sprinkler evaporation losses. Agricultural Engineering 36: 526-528.
Pair, C.H., W.H. Hinz, K.R. Frost, R.E. Sneed, and T.J. Schiltz. 1983. Irrigation. The Irrigation Association. Silver Spring, MD. 686 pp.
Schwab, G.O., R.K. Frevert, T.W. Edminster and K.K. Barnes. 1981. Soil and Water Conservation Engineering. 3rd Edition. John Wiley & Sons, Inc. New York. 525 pp.
Seginer, I. 1971. "Water losses during sprinkling." Transactions of the ASAE 14: 656-659, 664.
Whitty, B., R. Hill and D. McCloud. Gainesville, Florida 11WNW climatological data: 1992. Agronomy Dept., Univ. Fla., Gainesville, FL.