Introduction
This publication addresses the influence of irrigation scheduling on water use, plant growth, and development for young pongamia trees (Millettia pinnata (L.) Panigrahi). Young trees are defined as those below bearing age (three years and younger). The information provided is based on data collected during 2021–2022 in Florida flatwood soil. We compared three irrigation scheduling methods: soil moisture (SM)-based; evapotranspiration (ET)-based; and calendar (GR)-based. There are no irrigation guidelines for pongamia in Florida. Therefore, growers are currently following the calendar-based recommendations for young citrus trees. This document is intended for stakeholders dealing with pongamia irrigation management, including pongamia growers, land managers, certified crop advisors, water management districts, and other parties interested in pongamia irrigation management.
An Overview of Millettia pinnata (L.) Panigrahi
Pongamia, or pongam oil tree (Millettia pinnata (L.) Panigrahi), is a fast-growing legume tree originally from India that can grow on unproductive land and is adaptable to a wide range of agro-climatic conditions. For more information on pongamia, consult the Ask IFAS publication ENH657, “Pongamia pinnata: Pongam.” The tree belongs to the family Fabaceae and produces oval to flat seed pods with curved and pointed tips. It can adapt to elevated temperatures, high solar radiation, and arid environments (Rezazadeh 2022). In addition, the crop is used successfully for agricultural and environmental management. Studies have found pongamia to have the potential to grow as a restoration species in highly degraded forest areas and uptake nitrogen, water, and phosphorus (Biswas et al. 2011). Pongamia can fix about 47.4 mg of nitrogen per plant in 16 weeks under greenhouse conditions (Calica and Gresshoff 2019). Although pongamia is regarded as a drought-tolerant species with dense lateral root growth, the physiological traits that make the tree thrive under water-limited conditions are still poorly understood. Studies have reported that continuous water-limiting conditions for more than six months could negatively affect tree trunk diameter and height (Murphy et al. 2012), but drought thresholds have not been identified.
To cultivate more than two acres of a non-native species in Florida, it is necessary to obtain a permit from the Florida Department of Agriculture and Consumer Services and follow their crop management best management practices. For more information about permitting, consult the Ask IFAS publication SS-AGR-453, “Navigating the Non-Native Planting Rule: Permit Requirements for Large-Scale Plantings of Non-Native Species in Florida.” Pongamia best management practices, especially those related to water use, should be implemented to: increase yields in new and established plantings; comply with the best management regulation to grow the crop in Florida; and promote environmental stewardship by reducing the use of water and nutrients.
Pongamia Water Consumption Considerations
The literature on water requirements for optimal seed and oil production of pongamia is limited, but experts state that irrigation is crucial during the establishment phase for dry tropical and subtropical areas (Usharani 2019). In a hot, semi-arid climate, 45 gallons per tree per month are recommended, and the average water consumption per gram of biomass is about 0.31 gallons (Chaturvedi et al. 1984). Although water consumption and crop coefficients (Kc) have been evaluated for several tree crops in Florida, there is no information available on the Kc of pongamia. The Kc coefficient indicates the water requirements of the tree. For more information on crop coefficients, consult the Ask IFAS publication AE456, “Evapotranspiration-Based Irrigation for Agriculture: Crop Coefficients of Some Commercial Crops in Florida.”
Pongamia irrigation scheduling in Florida is currently based on citrus recommendations as a management baseline. For information on citrus irrigation guidelines, consult the Ask IFAS publication CPG12, “2023–2024 Florida Citrus Production Guide: Irrigation Management of Citrus Trees.” Note that citrus may have higher water requirements than pongamia. Citrus crop coefficients range from 0.7 to 1.1, with an average water use of 165 gallons per tree per month (Morgan et al. 2006). Preliminary calculations have shown that young pongamia crop coefficients range from 0.4 to 0.9 at this growth stage (Figure 1). A lower crop coefficient means that the tree requires less water.
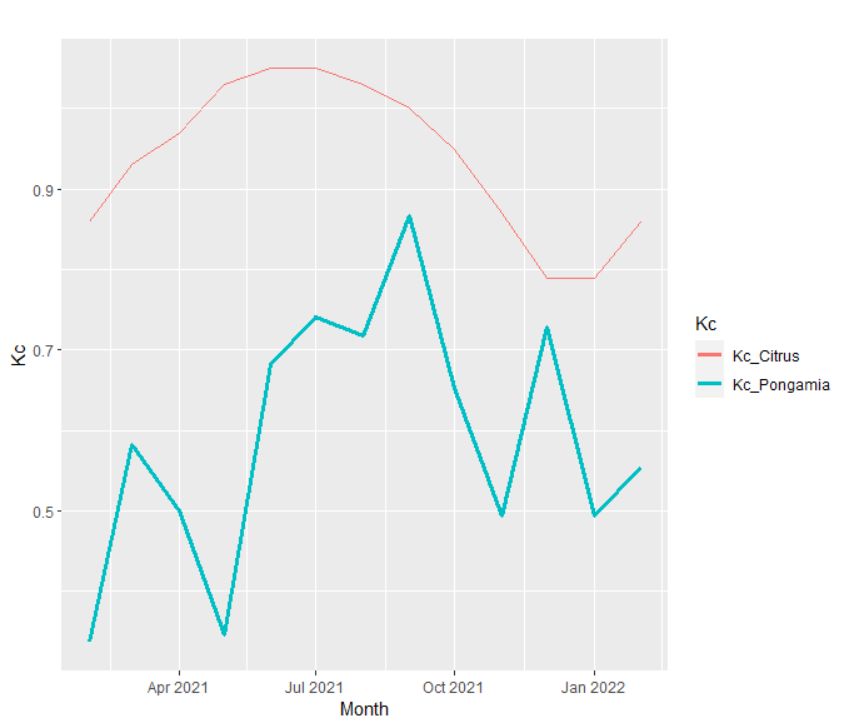
Credit: Daniel Palacios, UF/IFAS
Irrigation Trial
In February 2021, soil moisture sensors were installed in a pongamia field trial at the UF/IFAS Indian River Research and Education Center to evaluate water management strategies for the crop (Figure 2a–b). The trial consisted of three rows, each with 30 trees, representing different irrigation scheduling systems: SM-based, ET-based, and GR-based (citrus irrigation).
The SM-based irrigation schedules were initially calculated using soil water thresholds obtained from the Web Soil Survey and were later adjusted based on in-field sensor data. The field capacity threshold was adjusted following sensor installation and an irrigation event that led to soil saturation. After the irrigation event, when the sensor readings slowed down below the saturation peak, we obtained the practical field capacity. This value was then compared with the results obtained from gravimetric soil sampling. For more information on how to practically determine soil field capacity using soil moisture readings, consult the Ask IFAS publication AE460, “Interpretation of Soil Moisture Content to Determine Soil Field Capacity and Avoid Over-Irrigating Sandy Soils Using Soil Moisture Sensors.” The evaluated soil was Pineda sand, with a field capacity of 10.5% and a permanent wilting point of 3.8%. The ET-based schedules were calculated using the Penman-Monteith equation, based on the average of the previous 48 hours, and real-time data were collected from a weather station located at the trial site (Figure 2c). For more information on how to calculate evapotranspiration using the Penman-Monteith equation, refer to the Ask IFAS publication AE446, "Smart Irrigation Controllers: Operation of Evapotranspiration-Based Controllers." The GR-based schedules were set at a fixed schedule of two hours of irrigation three times per week. The trial used the same irrigation infrastructure and land configuration as typical citrus lands, with a micro-sprinkler irrigation system that had a flow and pressure of 10.5 gallons/h and 20 psi, respectively. Each tree was irrigated by one emitter located one foot from the trunk, and the trees were spaced 8 ft apart. In-field soil and weather sensors were connected to a datalogger and solenoid valve to control irrigation for each row automatically.
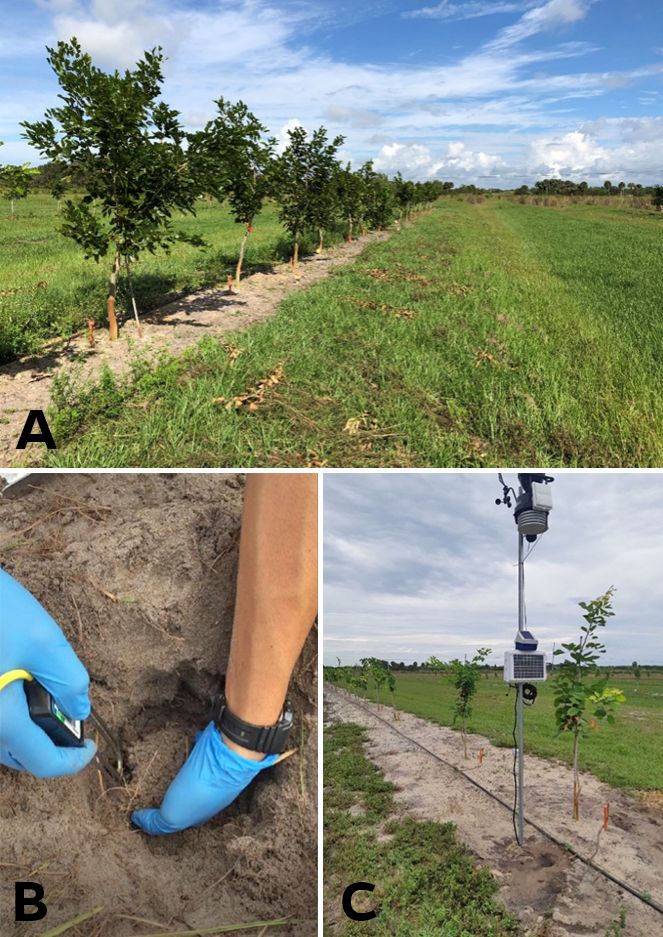
Credit: Daniel Palacios, UF/IFAS
Results
Water Use
In 2021, the SM-based and ET-based irrigation scheduling methods showed water savings of 23.3% and 23.9%, respectively, compared to the GR-based irrigation schedule (Table 1). Similarly, in 2022, the SM-based and ET-based irrigation scheduling methods showed water savings of 34.5% and 21.6%, respectively, compared to the GR-based irrigation schedule (Table 1). Figure 3 illustrates the volumetric water content (VWC) for each irrigation scheduling method. The SM-based (blue line) and ET-based (red line) methods show VWCs within the soil water-holding capacity thresholds and more frequent, but shorter irrigations. The GR-based method indicates soil saturation (higher than 20% VWC in Figure 3) and a volume of irrigation that exceeds the soil’s capacity to retain water. To optimize irrigation and maintain soil water availability for the trees, we recommend using the SM- or ET-based methods because they assist irrigation managers in irrigating more frequently and for shorter periods. For more details on how to interpret soil moisture content, refer to the Ask IFAS publication AE460, “Interpretation of Soil Moisture Content to Determine Soil Field Capacity and Avoid Over-Irrigating Sandy Soils Using Soil Moisture Sensors.”
Table . Summary of annual water applied per treatment.
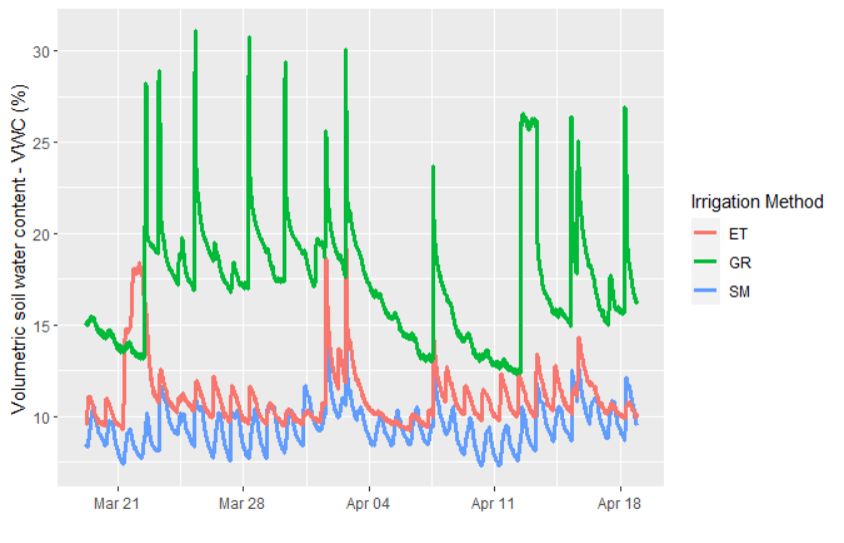
Credit: Daniel Palacios, UF/IFAS
Pongamia Physiological Responses per Irrigation Scheduling Method
The analysis of physiological development variables showed differences among irrigation methods. Specifically, the GR-based treatment showed significant differences in trunk diameter compared to the SM-based treatment. However, no significant differences were found between the GR-based and ET-based treatments. In terms of stomatal conductance, a significant distinction was observed between the ET-based and the SM-based methods, while no significant differences were noted between the SM-based and GR-based methods. Conversely, for transpiration rate, there was a significant difference between the SM-based and the ET-based and GR-based schedules. Despite the reduced water volume applied in the SM-based schedule resulting in a lower stomatal conductance rate, there were no substantial differences observed in the rate of carbon exchange among the treatments. This indicates that a lower rate of transpiration and stomatal conductance does not interfere with the physiological development of the tree. A 20% reduction in water application from the GR-based scheduling method appears viable for young trees without compromising tree and root growth at this early stage.
It is important to consider the impact of frequent rainstorms, extreme weather events, and water-table elevation when determining the best water management practices. Moreover, the management of pongamia pods is crucial to prevent them from being transported into waterways and causing potential environmental risks. This study, although still in its early stages, demonstrates that pongamia young trees can thrive with a reduction in water application of over 20%. Either soil moisture- or evapotranspiration-based irrigation scheduling is recommended for promoting healthy canopy and root growth, reducing environmental impacts, and minimizing nutrient losses.
References and Additional Resources
Biswas, B., P. T. Scott, and P. M. Gresshoff. 2011. “Tree Legumes as Feedstock for Sustainable Biofuel Production: Opportunities and Challenges.” Journal of Plant Physiology 168 (16): 1877–1884. https://doi.org/10.1016/j.jplph.2011.05.015
Calica, P. N., and P. M. Gresshoff. 2019. “Comparative Assessment of Biological Nitrogen Fixation in Pongamia pinnata, a Biofuel Legume Tree.” Philippine Agricultural Scientist 102 (1): 17–26.
Chaturvedi, A. N., S. C. Sharma, and R. Srivastava. 1984. “Water Consumption and Biomass Production of Some Forest Trees.” The Commonwealth Forestry Review 63 (3): 217–223. https://www.jstor.org/stable/42606415
Degani, E., M. Prasad, A. Paradkar, R. Pena, A. Soltangheisi, I. Ullah, B. Warr, and M. Tibbett. 2022. “A Critical Review of Pongamia pinnata multiplea: From Land Remediation and Carbon Sequestration to Socioeconomic Benefits.” Journal of Environmental Management 324:116297. https://doi:10.1016/j.jenvman.2022.116297
Dukes, M. D., M. L. Shedd, and S. L. Davis. 2022. "Smart Irrigation Controllers: Operation of Evapotranspiration-Based Controllers." AE446. Gainesville: University of Florida Institute of Food and Agricultural Sciences. https://edis.ifas.ufl.edu/ae446
Garg, K. K., S. P. Wani, and A. V. R. K. Rao. 2014. “Crop Coefficients of Jatropha (Jatropha curcas) and Pongamia (Pongamia pinnata) Using Water Balance Approach.” Wiley Interdisciplinary Reviews: Energy and Environment 3 (3): 301–309. https://doi.org/10.1002/wene.88
Gilman, E. F., D. G. Watson, R. W. Klein, A. K. Koeser, D. R. Hilbert, and D. C. McLean. 2019. “Pongamia pinnata: Pongam.” ENH657. Gainesville: University of Florida Institute of Food and Agricultural Sciences. https://edis.ifas.ufl.edu/publication/ST498
Herrera, E., S. M. Guzmán, and E. Murcia. 2021. “Common Questions When Using Soil Moisture Sensors for Citrus and Other Fruit Trees: AE551, 02/2021.” EDIS 2021 (2): 4. https://doi.org/10.32473/edis-ae551-2021
Kadyampakeni, D. M., K. T. Morgan, M. Zekri, A. W. Schumann, S. Guzmán, F. Alferez, and T. A. Obreza. 2022. “2022–2023 Florida Citrus Production Guide: Irrigation Management of Citrus Trees: CPG Ch. 15, CG093/CPG12, Rev. 4/2022.” EDIS 2022 (CPG). https://doi.org/10.32473/edis-cg093-2022
Kisekka, I., K. W. Migliaccio, M. D. Dukes, J. H. Crane, B. Schaffer, S. M. Guzmán, and H. K. Bayabil. 2019. “Evapotranspiration-Based Irrigation for Agriculture: Crop Coefficients of Some Commercial Crops in Florida: AE456/AE456, Rev. 6/2019.” EDIS 2019 (3). https://doi.org/10.32473/edis-ae456-2010
Lieurance, D., E. Rohrig, and S. Enloe. 2021. “Navigating the Non-Native Planting Rule: Permit Requirements for Large-Scale Plantings of Non-Native Species in Florida: SS-AGR-453/AG454, 06/2021.” EDIS 2021 (3). https://doi.org/10.32473/edis-ag454-2021
Morgan, K. T., T. A. Obreza, J. M. Scholberg, L. R. Parsons, and T. A. Wheaton. 2006. “Citrus Water Uptake Dynamics on a Sandy Florida Entisol.” Soil Science Society of America Journal 70 (1): 90–97. https://doi.org/10.2136/sssaj2005.0016
Murphy, H. T., D. A. O’Connell, G. Seaton, R. J. Raison, L. C. Rodriguez, A. L. Braid, D. J. Kriticos, T. Jovanovic, A. Abadi, M. Betar, H. Brodie, M. Lamont, M. McKay, G. Muirhead, J. Plummer, N. L. Arpiwi, B. Ruddle, S. Saxena, P. T. Scott, C. Stucley, B. Thistlethwaite, B. Wheaton, P. Wylie, and P. M. Gresshoff. 2012. “A Common View of the Opportunities, Challenges, and Research Actions for Pongamia in Australia.” Bioenergy Research 5:778–800. https://doi.org/10.1007/s12155-012-9190-6
Rezazadeh, A. 2022. “Pongamia: An Alternative Crop with Great Potential in Florida.” Specialty Crop Industry Magazine. https://specialtycropindustry.com/pongamia-an-alternative-crop-with-great-potential-in-florida/
UF/IFAS. 2022. “Assessment of Non-Native Plants in Florida's Natural Areas: Millettia pinnata.” https://assessment.ifas.ufl.edu/assessments/millettia-pinnata/
Usharani, K. V., D. Naik, and R. L. Manjunatha. 2019. “Pongamia pinnata (L.): Composition and Advantages in Agriculture: A Review.” Journal of Pharmacognosy and Phytochemistry 8 (3): 2181–2187.
Zotarelli, L., M. D. Dukes, and K. T. Morgan. 2019. “Interpretation of Soil Moisture Content to Determine Soil Field Capacity and Avoid Over-Irrigating Sandy Soils Using Soil Moisture Sensors.” AE460. Gainesville: University of Florida Institute of Food and Agricultural Sciences. https://edis.ifas.ufl.edu/publication/AE460