Fertilization of Sweet Corn, Celery, Romaine, Escarole, Endive, and Radish on Organic Soils in Florida
Introduction
Celery, sweet corn, endive, escarole, romaine lettuce, and radish are important crops on organic soils in Florida. This bulletin summarizes fertilization research results with these crops from the 1940s to the present. New interpretations of these compiled works were used to produce updated UF/IFAS Extension recommendations for fertilization of these six vegetable crops. The recommendations presented in this publication supersede those found in Bulletin 876 (Sanchez, 1990), Bulletin 757 (Guzman et al., 1973), Circular 806 (Hochmuth and Hanlon, 1989), and fertilization recommendations in all crop production guides printed previous to this circular.
Since the body of literature summarized in this document covers more than five decades, perceptions regarding fertilizer use, its impact on the crop, and possible environmental consequences have changed. Use of soil testing was sporadic in the literature, however, if available, soil-test values are reported. This document does not attempt to calibrate the soil- testing procedures used at the UF/IFAS Everglades REC laboratory or the UF/IFAS Extension Soil Testing Laboratory in Gainesville. However, this document includes tables that couple fertilizer rates with soil test results.
It is our intention to show that reasonable fertilizer recommendations can be found to work with a wide range of field conditions. To that end, the indicated literature supports these newer recommendations. Furthermore, no statistical models are imposed upon the datain order to avoid the implied mathematical assumptions upon which each model is based. Often, statistical evaluation of results were not regularly included by the researchers. For some crops, few studies have been conducted, negating the use of rigorous statistics. Lastly, the new fertilizer recommendations are coupled to soil-test values using a procedure patterned after Sanchez (1990). We have made no attempt to substantiate the soil-test calibration. Its existence or validity is beyond the scope of this document. In those situations, data from crops that are physiologically similar were used as aids to modernize fertilizer recommendations. The evaluation of fertilizer recommendations using this approach is strengthened by the use of new information not available when older recommendations were formulated. Fertilization recommendations can be expected to change as management practices, environmental goals, and economical aspects evolve through time. Fertilization enhances crop production. However, neither are static and reevaluation in light of new information improves the process.
Soil pH
The pH of the organic soils used for vegetable production within the Everglades Agricultural Area (EAA) has increased with time (Beverly, 1984a). The increase in pH has resulted from several factors including the practice of summer (fallow) flooding. As the depth of the tilled zone to the underlying limestone decreases (due to oxidative loss of the organic matter), pH may also be expected to increase. Increasing pH reduces availability of phosphorus and certain micronutrients for vegetable production. Several studies have addressed the potential problem of alkaline soil reaction. Early work showed that nutrient availability was most favorable in soils with a pH of about 6.0 (Lucas and Davis, 1961).
Celery
Sulfur (S) application at 1000 lb per acre reduced the pH of an Okeelanta peaty muck from 6.1 to 5.4 (Hortenstine and Forsee, 1960). Celery yield significantly increased from 865 to 910 crates per acre with acidification. In another study (Burdine and Guzman, 1965a), S addition reduced soil pH from an initial range of 6.6 to 6.8 in winter and spring crops. In the winter crop, 'Utah-52-70' and 'Summer Pascal 259-19' yields were not affected by soil pH over the range of 5.4 to 6.3 (at harvest). However, in the spring crop, yields of 'Utah 52-70' and 'Summer Pascal 259-19' decreased as the pH decreased from 6.2 to 4.9 with the largest decrease for 'Utah 52-70' occurring as the pH decreased from 5.2 to 4.9.
Soil pH also had differential effects on celery growth and yield in a later study (Burdine and Guzman, 1968; 1969a). Yield of 'Summer Pascal' was not affected by soil pH in the range of 5.2 to 6.2 while 'Utah 52-70', 'FFVA 2-13', and 'Florida 683' yields decreased as pH dropped over the same range. Blackheart disorder increased, but cracked petioles decreased, as pH was reduced (Burdine and Guzman, 1968).
Most of the above work was on soils with an initial pH of about 6.2 prior to l965. By the mid l980s (Beverly l984a), the average soil pH of vegetable soils of Everglades area, however, had increased to about 7.1. In a pot study, celery growth was not affected by S application to two diverse Histosols (Lauderhill, pH 7.2; Terra Ceia, pH 6.1). Maximum pH decreases were 0.3 for Lauderhill and 0.6 for Terra Ceia (Beverly and Anderson, 1988). In a field study, acidification enhanced manganese availability and yield on a Lauderhill muck with an initial pH of 6.7 (Beverly, 1987). Soil pH was reduced 0.5 unit with 2000 lb S per acre. There were celery yield benefits to soil acidification even with foliar applications of manganese (Beverly, 1984b; Beverly, 1987).
Based on the above work, celery was shown to respond to S application (acidification) when the pH is above 6.5 but probably not when pH is below 6.2. Sulfur application may be detrimental on soils with an initial pH less than 6.0.
Sweet Corn
There were no detailed reports on response of sweet corn to changes in soil pH. No critical soil pH value has been established for sweet corn production on Everglades organic soils.
Romaine Lettuce
High yields and normal plant micronutrient concentrations in leaves were achieved on soils with pH in the range of 5.7 to 5.9 (Burdine et al., 1977). Although little research has been done with romaine lettuce on soils with pH outside this range, a desired pH range of 5.0 to 6.0 was suggested by Sanchez (1990).
Endive and Escarole
Yield of endive was better where S was used to reduce the pH from 6.1 to 5.7 (Forsee et al., 1950). Escarole yields increased as soil pH at harvest was reduced over the range of 6.6 to 5.6 (Burdine and Guzman, 1968). Similar results were obtained in other studies with escarole (Burdine and Guzman, 1965b). The above studies support a desired pH range of 5.0 to 6.0.
Radish
On a Pahokee muck with an initial pH of 7.0, various acidifying materials including elemental S were evaluated for effects on radish production (Lockhart and Sanchez, 1989). Phosphoric acid, ammonium sulfate, ammonium nitrate, and urea ammonium nitrate, applied at 2000 lb per acre reduced soil pH more compared to elemental S. Maximum pH reduction (to 6.0) occurred with ammonium sulfate 41 days after application. Acidification had no effect on radish yield; however, there was a linear increase in split radishes as pH increased from 6.0 to 7.0. Interpretation of results of this study is difficult because effects of fertilizer sources also on soil pH is confounded with variable effects of fertilizer sources on soil-soluble salt and nitrogen status. In addition, the imposed variable, soil-soluble salt conditions, probably led to unpredictable effects on soil pH measurement. In another radish study, reducing pH below 6.0 increased radish yield (Beverly, 1986). Foliar Mn was effective in increasing radish yield, and some acidifying sources increased yield even more when used in combination with foliar Mn.
Soil pH Recommendations
Desired soil pH ranges for several vegetables grown in the EAA are presented in Table 1. The soil pH can be adjusted by use of elemental S; however, large reductions in pH (Sanchez, l990) require large amounts of S. Large S applications are expensive and repeat applications might be needed since the organic soil is highly buffered against changes in pH. Flooding almost totally removes any previous benefit from acidification of the soil (Beverly, 1984b). Therefore, S applications need to be evaluated economically by the grower. For some crops, it might be more economical to use foliar sprays or banding fertilizers to correct micronutrient deficiencies caused by high pH fertilizers.
Many research studies indicated that Mn deficiency was a major limiting factor caused by high pH. Foliar Mn treatments were usually effective in correcting Mn deficiencies in most studies. Banding S plus Mn appeared to have promise for correcting Mn deficiencies (Beverly, 1984b; Beverly, 1986).
Toxicities due to excess plant-available Mn or Al can be problems in mineral soils with pH below 5.0. Although not common in Histosols, Mn and Al toxicities might be found in certain isolated situations, for example, shallow mucks overlaying sand. Excessive use of Mn fertilizers or Mn-containing fungicides contributes to Mn buildup in soils that might lead to Mn toxicity.
Micronutrients
Several studies have been conducted since the 1920s on micronutrient fertilization of vegetables on organic soils. Not all crops have been studied for all micronutrients (Locascio and Fiskell, 1988) so that micronutrient research will be summarized here by nutrient.
Copper (Cu)
Early research by Allison et al. (1927) revealed the importance of Cu for vegetable production on new peat soils. Further research led to the recommendation of 12 lb Cu per acre for new organic soils (Forsee, 1940; Forsee, 1949; Kretschmer and Forsee, 1964). Up to four lb Cu per acre should be applied to the second crop after which no further Cu additions are required. Copper oxide was similar to copper sulfate as a source of Cu (Forsee et al., 1954; Kretschmer and Forsee, 1964).
Manganese (Mn)
In a study on a previously uncultivated EAA Histosol, a broadcast application of 5.6 lb Mn per acre was made. Celery yields did not respond to an additional 1.5 lb Mn per acre applied in six foliar sprays (Burdine and Guzman, 1965a) indicating that 5.6 lb Mn per acre was adequate for celery. Unfortunately, no preplant soil-test Mn concentrations were reported. In another study on soil previously planted to potatoes, celery did not respond to foliar Mn (Burdine and Guzman, 1965a). In the above studies, celery responded negatively to reductions in pH over the range of 4.9 to 6.2 (from treatment with S) and Mn concentration in the outer petiole increased. On a soil to which 7 lb Mn had been applied, celery did not respond to foliar Mn. The pH of the soil at harvest was between 5.4 and 6.3 (Burdine and Guzman, 1968). Foliar Mn, at a rate of 0.25 lb Mn per acre in each of seven weekly sprays did not improve celery yield compared to that from soil-applied Mn at 6.2 lb per acre. The soil had an initial pH of 7.0. Manganese concentration in the outer petiole was above the deficiency level of 4.0 ppm Mn. Likewise, celery did not respond to foliar Mn in pot studies (Beverly and Anderson, 1988). In field studies on a soil with a pH of 6.7, Mn was broadcast at 11 lb per acre to all plots (Beverly, 1987). Foliar applications of Mn increased yields even when the soil pH was reduced with acidifying materials.
Locascio and Fiskell (1988) reported that sweet corn yield responded positively to Mn application on mineral soils with pH above 6.5.
Burdine and Guzman (1968) found no benefit to treating escarole with 6 lb Mn per acre applied with the broadcast preplant fertilizer mix. Soil pH at harvest was 6.6 in that study. In a study of P and K fertilization of escarole and endive (soil pH 5.9), Mn was applied uniformly at 8 lb per acre. Manganese concentration of escarole and endive leaves remained within the adequate range throughout the season (Sanchez and Burdine, 1989). Application of 8 lb Mn per acre also maintained romaine lettuce leaf Mn at adequate levels (Burdine et al., 1977).
Radish yield was increased by foliar Mn and soil application of Mn with sulfur (Beverly, 1986). Radish growth and yield were not affected over a wide range of soil pH (Lockhart and Sanchez, 1989) suggesting Mn nutrition was not a factor. These divergent radish responses show a need for more work on pH and Mn interactions affecting radish yield.
For the above crops, no Mn should be needed on soils with a pH less than 5.7 (Sanchez, 1990). Where soil pH is above 5.8, broadcasting 8 lb Mn per acre is recommended. Manganese deficiency can be alleviated by using water soluble sources of Mn applied to the soil preplant (Gascho et al., 1971). Application of S and Mn together in a band may be beneficial for celery and radish (Beverly, 1984b;1986). When soil pH is above 6.5, foliar application of 0.75 to 1.0 lb Mn per acre may be beneficial.
Zinc (Zn)
Zn deficiencies were first noted by Allison et al. (1927). Early work showed that beans grown on Histosols responded to Zn (Townsend, 1936; Forsee, 1950), but little work has since been conducted on Zn nutrition of vegetables on Histosols. Therefore, Zn recommendations are largely based on estimated crop requirements (Sanchez, 1990). Recommendations are for 8 lb Zn per acre for soil application In some instances, soil-applied Zn might not be effective, and foliar application of up to 0.25 lb Zn per acre may be needed twice per week for two weeks as indicated by tissue analysis.
Boron (B)
Several celery disorders have been associated with B deficiency. Cracked petiole of celery was reduced by broadcasting 1.0 to 1.5 lb B per acre (Beckenbach, 1939). Cracked petiole was reduced, but not eliminated by foliar B applications. When B foliar sprays were used, there was a linear decrease in yield with decreasing soil pH (6.2 to 4.9). Benefits to foliar B occurred in conjunction with soil-applied rates up to 2.8 lb B per acre (Burdine and Guzman, 1965a). Despite soil application of 2.2 lb B per acre, celery cracked petiole and nodal cracking were reduced, but not eliminated, after seven foliar sprays of B at 0.2 lb B per acre each (Burdine and Guzman, 1969a). Nodal cracking was not affected by soil B applications, but was reduced following six sprays of 0.35 lb B per acre each (Burdine and Guzman, 1969b).
Little research has been reported for B fertilization of sweet corn, endive, escarole, romaine, or radish on Histosols. Recommendations for B fertilization are based on apparent crop nutrient requirements. Up to 2 lb B per acre should be applied to the soil for all crops on newly cultivated Histosols. For celery, up to 2.5 lb B per acre should be applied with the preplant fertilizer mix (Sanchez, 1990). For endive, escarole, and romaine, 1.5 lb B per acre should be applied. Only 1.0 lb B per acre is needed for sweet corn and radish, which are low-B requiring crops. No B is recommended for crops with a low B requirement that follow crops that have been fertilized with B.
Iron (Fe)
Deficiencies of Fe on organic soils are rare and little research has been conducted on Fe fertilization. Soil-applied Fe has not been effective in correcting Fe deficiency symptoms for vegetables grown on Histosols (Burdine, 1976). Foliar Fe applied at 0.25 lb Fe per acre twice per week for two weeks is recommended to control the deficiency (Sanchez, 1990).
Micronutrient Summary
A summary of micronutrient fertilization is presented in Table 2 for celery, sweet corn, endive, escarole, romaine, and radish. These recommendations are based on estimated crop nutrient requirements since little fertilization research has been conducted for micronutrients. The efficiency of micronutrient fertilization might be enhanced by banding alone or with an acidifying source compared to broadcasting. Fertilization practices should be validated and guided by crop response and following tissue analysis trends (Hochmuth et al., 1991).
Calcium, Magnesium, and Sulfur
Histosols usually contain adequate amounts of calcium (Ca), magnesium (Mg), and Sulfur (S). Therefore, no recommendations are made for these nutrients for most crops.
The incidence of celery blackheart, a calcium-related physiological disorder, decreased with the use of Ca sprays or drenches directed at the heart of the plant (Geraldson, 1953; Guzman et al., 1973). Research has shown that blackheart is most serious where soluble salt concentration of the soil is high (Westgate et al., 1954; Geraldson, 1953; Westgate, 1951). Crops excessively fertilized with nitrogen or potassium appear to be most subject to blackheart (Westgate, 1951). For crops growing under warm conditions (after April), blackheart can be reduced with weekly sprays or drenches of 5 lb of calcium chloride in 100 gal. water per acre, directed at the celery plant heart. Spraying should begin 50 days after transplanting (Sanchez, 1990). At 70 days after transplanting, drenching with 5 lb calcium chloride in 200 gal. water might be needed. Avoiding overfertilization might minimize the need for Ca foliar sprays and drenches.
Other factors affecting blackheart include water and cultivation practices. Inadequate irrigation can worsen blackheart. Since the outer leaves transpire more than heart leaves, Ca is preferentially moved to the outer leaves at the expense of the heart. Reduced Ca levels in the heart promote blackheart of celery.
Calcium is absorbed via young root tips. Damage to these tips through excessive irrigation (flooding) or root pruning during late cultivation can reduce Ca uptake. Reduced Ca uptake can promote blackheart.
Phosphorus (P)
Celery
Celery has shown moderate responses to broadcast P. Summaries of research (Table 3) with P are presented in Figure 1. One of the earliest detailed studies on celery response to P was by Hortenstine and Forsee (1960). The study was conducted on a previously cropped commercial farm on Okeelanta peaty muck with a P water index (Pw) of 22. Yields were at or above 95% relative yield with P fertilization between 160 and 320 lb P2O5 per acre. Another early study (Burdine and Guzman, 1969c) produced similar yield responses. Similar responses were reported by Sanchez et al. (1990a), Espinosa (1992), and Espinosa et al. (1993). In most studies reported in Figure 1, 95% relative yield was obtained with 100 lb P2O5 per acre or more. Based on the above research results, the maximum recommendation for P for celery is 260 lb P2O5 per acre. There does not appear to be any large benefit from banding compared to broadcasting P for celery (Figure 2) (Espinoza, 1992; Espinoza et al., 1993). The new P fertilization recommendations are shown with soil-test index values (Pw) in Table 4.
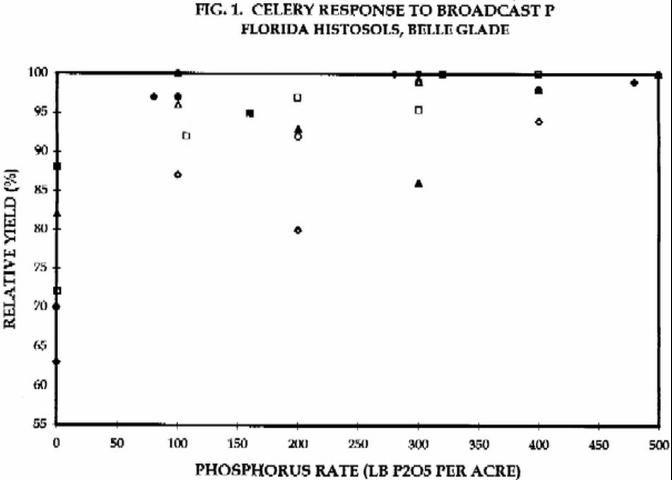
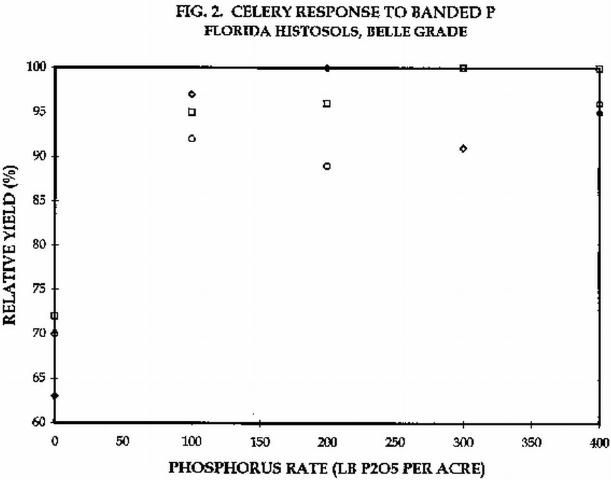
Sweet Corn
Summaries of research results for sweet corn response to broadcast P fertilization are presented in Figure 3. Response to broadcast P appeared to level off between 150 and 200 lb P2O5 per acre (Sanchez et al., 1991a). Response to banded P leveled off between 50 and 100 lb P2O5 per acre in one early study (Figure 4) (Burdine and Deen, 1974). In another early study, banding P was reported to be superior to broadcasting P (Forsee et al., 1952). No responses to P were observed on soils with a P water index of 8 or above; however, there were positive responses to 70 lb P2O5 per acre on a soil with an index of 5 and up to 100 lb P2O5 on a soil with an index of 4 (Forsee et al., 1950; Forsee et al., 1952). In a comparison of banding and broadcasting, the relative efficiency of banded to broadcast was greater than 3:1 for soils with a Pw less than 4, and was equal for soil test indices greater than 22 (Sanchez et al., 1991a).
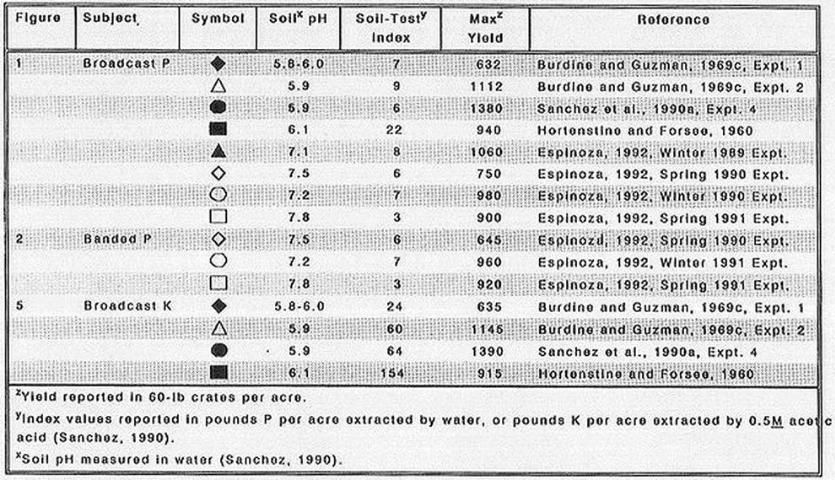
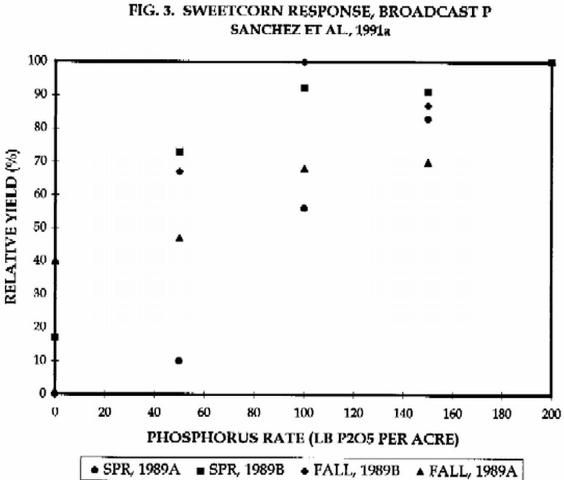
Based on the above research results, broadcasting P is not recommended, especially in light of increasing soil pH in the EAA. The maximum P recommendation for sweet corn is 160 lb P2O5 per acre banded at planting two to three inches to the side of the row and two to three inches deep. The P2O5 fertilizer recommendations are coupled with Pw indices in Table 4.
Endive, Escarole
In earlier work with endive (chicory) and escarole, no responses were observed to P fertilization of soils with Pw indices of 4 or 11 (Forsee et al., 1950, pH = 6.1; Forsee et al., 1952, pH = 5.8). In more recent research with both crops, responses to broadcast P appeared to level off after 300 lb P2O5 per acre (Burdine et al., 1977, soil pH = 5.9, Pw = 2; Sanchez and Burdine, 1989).
Research with crisphead lettuce showed that P rate could be reduced by banding (Sanchez et al., 1990b). Due to similar production practices for lettuce, endive, and escarole, it should be possible to obtain efficiencies by banding P at 200 lb P2O5 per acre for endive and escarole. The P fertilization recommendations for endive and escarole are presented in Table 4 with Pw indices.
Romaine Lettuce
Only two studies of romaine response to P fertilization have been reported. Response to P was quadratic in one study (soil pH = 5.9, Pw = 2) (Burdine et al., 1977) and response was not significant in another (Pw = 8) (Guzman et al., 1989). This research showed that romaine lettuce probably does not need more P than endive or escarole. Maximum P recommendation for romaine lettuce is 200 lb banded P2O5 per acre. The P fertilization recommendations are presented in Table 4.
Radish
No response to P fertilization was observed in the first crop on a soil with a P water index of 6 (Burdine and Guzman, 1967). In this research, 20, 100, and 180 lb P2O5 per acre were applied and three crops of radishes were grown in succession without further fertilization. The P water indices for the start of the second season were 6, 12, and 17 for the 20, 100, and 180 lb P2O5 per acre treatments, respectively. Yield responses were apparent in the second season with the 180-lb P2O5 plots providing 100 percent relative radish yield, the 100-lb plots providing 97 percent, and the 20-lb plots providing 94 percent.
The P water indices for the start of the third crop were 8, 14, and 19 for the 20, 100, and 180-lb per acre treatments. Yield responses also were significant in the third season. Yields were 92, 97, and 100 percent for the 20, 100, and 180-lb P2O5 per acre treatments.
Based on the above responses to P, the maximum P recommendation is 100 lb P2O5 per acre. This amount is for one to three crops grown in succession. The P fertilization recommendations appear in Table 4.
Potassium (K)
Celery
Minimal responses to K have been observed for celery, see Table 3 and Figure 5. All but one mean observation were above 94 percent relative yield. In an early study (Hortenstine and Forsee, 1960), 95 per cent relative yield resulted with no K fertilization of a soil with an acetic acid-extractable K index (Koac ) of 161. Small responses to K fertilization were observed on soils with K indices of 24 and 60 (Burdine and Guzman, 1969c). In another study, small responses to K were observed on a soil with a K index of 51 (Sanchez et al., 1990a). In that same report, 29 out of 39 mean observations across eight experiments were above 92 percent relative yield for soil K indices above 135. Relative yields reached nearly 100 percent at K indices above 270.
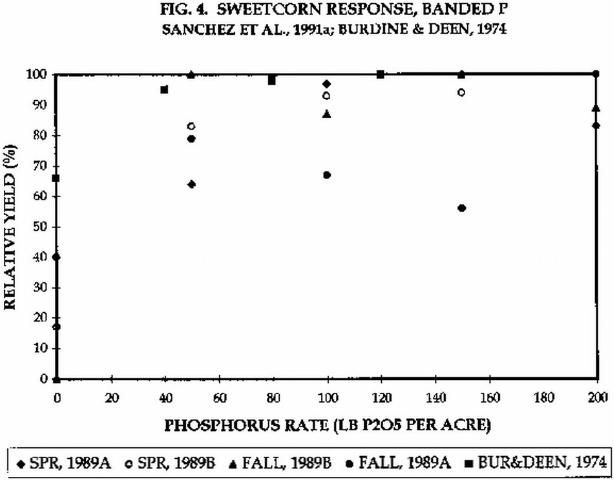
Based on above research, the maximum K recommendation is 300 lb K2O per acre. Guzman (1969) showed that applying all K broadcast before planting was acceptable during dry growing seasons. Split-applications were better during the fall season when rainfall might leach K. Since K can leach and since rates as large as 300 lb K2O per acre could lead to soluble salt injury to plants, the K applications should be split into three portions. One-third should be broadcast before planting and the remainder can be applied in two side dressings one and two months after transplanting (Forsee and Hoffman, 1948; Guzman, 1969). The first sidedressing can be applied in bands two to three inches to the side and two to three inches deep. The last sidedressing can be applied at the same depth in the center between the rows (Guzman, 1969). The soil test values for K fertilization of celery are presented in Table 5.
Sweet Corn
Little research has been conducted for K fertilization of sweet corn. In one study, on a soil with a K index of 58, the maximum response was to 80 lb K2O per acre (Forsee et al., 1950). In another study on a soil with an acetic acid extractable K index of 127, there was no response to K fertilization. In two other studies on soils with K indices of 58 and 80, there were no responses to K fertilization (Forsee et al., 1952).
The maximum K recommendation for sweet corn is 120 lb K2O per acre broadcast at planting. The acetic acid soil test values and fertilization recommendations are presented in Table 5.
Endive, Escarole
Minimal responses to K were observed with endive and escarole on a soil with an acetic acid K index of 45 (Burdine et al., 1977). Results of five studies (three endive and two escarole) were reported by Sanchez and Burdine (1989). For the two escarole studies, the soil K indices were 119 and 148 after fertilization with 120 lb K2O per acre. Yield response was linear at the first site and nonsignificant at the second. Endive responses were opposite to those of escarole at the same sites. Endive and escarole yields at site two were less than one-half of the yields at site one. In a third endive study on a site with a prefertilization Koac of 165, there was no yield response to K fertilization.
In earlier research on a soil with an acetic acid K index of 83, there was no endive yield response to K fertilization (Forsee et al., 1952) In two other studies, endive responded to applications up to 300 lb K2O per acre applied on a soil with K index of 48, and up to 80 lb K2O per acre applied to a soil with K index of 36 (Forsee et al., 1950).
Most of the above research results support a maximum K fertilization recommendation for endive and escarole of 200 lb K2O per acre broadcast before planting. The acetic acid soil test values for K fertilization of endive and escarole are presented in Table 5.
Romaine Lettuce
Only two studies of K fertilization of romaine lettuce were reported (Burdine et al., 1977, Koac = 50; Guzman et al., 1989, Koac = 72). In one study on a soil with an Koac index of 81, there was no response to K fertilization (Guzman et al., 1989). In the other study, there was a linear response over the range of 100 to 320 lb K2O per acre (Burdine et al., 1977). In this study, 93 percent relative yield was realized at 200 lb K2O per acre.
Maximum K fertilization response for romaine lettuce is probably similar to the crisphead lettuce recommendation of 200 lb K2O per acre because both crops have similar biomass yields and are grown with similar cultural practices. Acetic acid extractable K values with K fertilization recommendations are presented in Table 5.
Radish
Only two K fertilization studies have been conducted with radish. Only one report contained data on prefertilization acetic acid K index (Burdine and Guzman, 1967). On a soil with a K index of 69, there was no response to K in the first crop for K applications of 20, 140, and 260 lb K2O per acre. After germination of the second crop, the Koac indices were 53, 86, and 122 for the three K treatments. No fertilizer was added for the second crop. Relative yields were 88, 98, and 100 per cent for the three residual K levels. At the beginning of the third season, Koac indices were 41, 64, and 95 for the three original K treatments. Relative yields of the third crop were 74, 91, and 100 percent with the three K treatments. Results from a second study, conducted in containers with Florahome peat from northern Florida agreed with the above work. Radish yield responded to up to 120 lb K2O per acre for one crop (Stephens and Thompson, 1963; soil pH = 6.0; Pw = 4; Koac = 16).
Maximum K fertilization recommendations for radish are 100 lb K2O per acre on a "per crop" basis to be broadcast before the crop. The acetic acid extractable K soil test values and K fertilization recommendations are presented in Table 5.
Other Soil Extractants
The use of other extractants for determining fertilization need is an ongoing research effort. For example, the Mehlich-1 (M-1) extractant has been in use at the UF/IFAS Extension Soil-Testing Laboratory, Gainesville, since the late 1970s (Hanlon et al., 1990), (Table 6). The effective use of this extractant continues to be a focus of numerous field studies on mineral soils (Hanlon and Hochmuth, 1992). Additional work with this extractant on a muck soil (pH=5.0) in the Fellsmere, FL, area showed that the M-1 accurately predicted lettuce response during several seasons (Diaz et al., 1988). However, on soils with a wider range in soil pH, the M-1 and water extraction methods were not as effective as the Mehlich-3 extractant (Sanchez and Hanlon, 1990) in predicting lettuce response in the Everglades Agricultural Area.
Both the water and M-1 methods are sensitive to soil pH, especially when the pH exceeds 6.5. The acids that comprise the M-1 extractant are partially neutralized by carbonates within the soil, decreasing the chemical effectiveness of the M-1 to that of water. To that end, research with extractants, such as the Mehlich-3 and a modification of the 0.5 M acetic acid methods, has been initiated (Hanlon and Neal, unpublished data for vegetables; Anderson et al., 1990). These initial research efforts appear to confirm that the Mehlich-3 and modified acetic-acid methods are satisfactory extractants over a wider soil-pH range than either the M-1 or water extractants. However, in five field studies in the Lake Apopka region (Crnko et al., 1993) with a range in soil pH from 5.3 to 6.2, the M-1 correctly identified soils with high levels of P. No response to fertilizer P was predicted, and no crop response (sweet corn and carrots) was found.
As technological advances improve analytical methods within soil-testing laboratories, it is reasonable to expect that field-verified research will identify better soil-test methods. Soil testing is only one tool upon which more informed management decisions might be based. It is logical to assume that better extraction methods and more precise fertilizer recommendations will evolve with time to improve fertilizer use efficiency.
Nitrogen
Celery
Guidelines for N fertilization were provided by Sanchez (1990), and were based on research by Burdine (1976) and Guzman (1969). For crops to be harvested before December 1, two split-applications of 40 lb N per acre are recommended one and two months after transplanting. For crops to be harvested between 1 December and 31 March, two applications of 60 lb N per acre are recommended, and for crops to be harvested after 1 April, two sidedressings of 30 lb N per acre are recommended.
Sweet Corn
The recommendation is to topdress 40 lb N per acre before the tassel appears in the whorl (Sanchez et al., 1989; Figure 6).
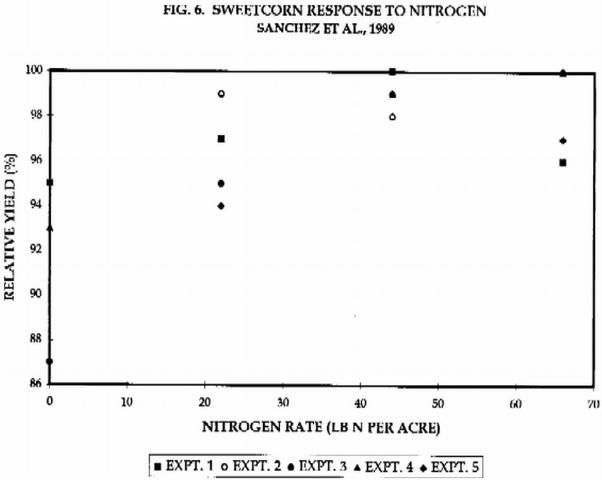
Endive, Escarole, and Romaine Lettuce
Up to 60 lb N per acre can be applied for crops to be harvested between 15 December and 15 March (Burdine et al., 1977; Burdine and Guzman, 1960). Nitrogen should be reapplied after rainfall of three inches or more and up to one-half (30 lb N per acre) should be reapplied after 1.5 to 3.0 inches of rain (Burdine et al., 1977; Burdine and Guzman, 1960).
Radish
No response to N was observed in eight studies (Sanchez et al., 1991b). Nitrogen application is not recommended for radishes.
Literature Cited
Allison, R. V., O. C. Bryan, and J. H. Hunter. 1927. The stimulation of plant response on raw peat soils of the Florida Everglades through the use of copper sulfate and other chemicals. Fla. Agr. Exp. Sta. Bull. 190.
Anderson, D.L., R. N. Raid, R. S. Irey, and L.J. Henderson. 1990. Association of sugar cane rust severity with soil factors in Florida. Plant Disease 74:683–686.
Beckenbach, J. R. 1939. Fertility program for celery production on Everglades organic soils. Fla. Agr. Exp. Sta. Bull. 333. p. 11–12.
Beverly, R. B. 1984a. Nutritional survey of the Everglades vegetable industry. Proc. Fla. State Hort. Soc. 97:210–205.
Beverly, R. B. 1984b. Managing high soil pH and Mn availability in Everglades vegetable production. Univ. Fla. Everglades Res. Ed. Ctr. Res. Rpt. 11. 17 pp.
Beverly, R. B. 1986. Radish responses to foliar nutrient sprays and pH adjustment of an organic soil. J. Fert. Issues 3:75–79.
Beverly, R. B. 1987. Celery response to foliar nutritional sprays and acidification of a Histosol. HortScience 22(6):1271–1273.
Beverly, R. B., and D. L. Anderson. 1988. Response of pot-grown celery to foliar Mn, soil P, and soil acidification of two Histosols. Soil Crop Sci. Soc. Fla. Proc. 47:49–52.
Burdine, H. W., and V. L. Guzman. 1960. Fertility studies with vegetable crops on the organic soils of the Everglades. Fla. Agr. Exp. Sta. Ann. Report. p. 282.
Burdine, H. W., and V. L. Guzman. 1965a. The response of some green celery varieties to pH adjustment with sulfur on Everglades organic soil. Proc. Fla. State Hort. Soc. 78:148–155.
Burdine, H. W., and V. L. Guzman. 1965b. Response of escarole to pH adjustment and some other nutritional factors on new sawgrass peat. Univ. Fla. Everglades Res. Ed. Ctr. Res. Rpt. EV-65-6.
Burdine, H. W., and V. L. Guzman. 1967. Radish response to phosphorus and potassium fertilizers on Everglades organic soil. Univ. Fla. Everglades Station Mimeo Rpt. EES 67-12. 6 pp.
Burdine, H. W., and V. L. Guzman. 1968. Some soil pH effects on soil tests and growth of some vegetable crops on Everglades organic soil. Univ. Fla. Everglades Sta. Mimeo Rpt. EES 68-9. 8 pp.
Burdine, H. W., and V. L. Guzman. 1969a. Celery cultivar responses to pH adjustment on Everglades organic soil. J. Amer. Soc. Hort. Sci. 94:520–523.
Burdine, H. W., and V. L. Guzman 1969b. Nutritional factors affecting nodal cracking of some celery cultivars. Soil Crop Sci. Soc. Fla. Proc. 29:351–362.
Burdine, H. W., and V. L. Guzman. 1969c. Some celery responses to fertilizer levels and soil test results. Univ. Fla. Everglades Sta. Mimeo Rpt. EES 69-17. 13 pp.
Burdine, H. W., and W. W. Deen. 1974. Responses of three sweet corn hybrids to liquid polyphosphate applied in the band. Univ. Fla. Belle Glade Agr. Res. Ed. Ctr. Res. Rpt. EV-1974-6. 9 pp.
Burdine, H. W. 1976. Vegetable fertilizer recommendations and practices for the Everglades Histosols. Univ. Fla. Everglades Res. Ed. Ctr. Rpt. EV-76-5.
Burdine, H. W., C. B. Hall, and J. R. Hicks. 1977. Response of some leafy vegetables to varying fertility levels on Terra Ceia muck. Soil Crop Sci. Soc. Fla. Proc. 36:46–51.
Crnko, G.S., A. Ferrer, E.A. Hanlon, C.A. Neal, and J.M. White. 1993 Carrot and sweet corn yields when fertilized according to soil test results. Proc. Fla. State Hort. Soc. 106:199–201
Diaz, O.A., E.A. Hanlon, G.J. Hochmuth, and J.M. White. 1988. Phosphorus and potassium nutrition of lettuce on a Florida muck. Soil Crop Sci. Soc. Fla. Proc. 47:36–41.
Espinoza, L. A. 1992. Response of celery to phosphorus rate and placement on Histosols. Masters thesis, Univ. Fla. Grad. School. 39 pp.
Espinoza, L., C. A. Sanchez, and T. J. Schueneman. 1993. Celery yield responds to phosphorus rate but not to phosphorus placement on Histosols. Hort Science 28:1168–1170.
Forsee, W. T., Jr. 1940. Recent plant responses to some micronutrient deficiencies on Everglades peat. Soil Crop Sci. Soc. Fla. Proc. 2:53–58.
Forsee, W. T., Jr., T. W. Erwin, and A. E. Kretschmer. 1954. Copper oxide as a source of fertilizer copper for plants growing on Everglades organic soils. Fla. Agr. Exp. Sta. Bull. 552.
Forsee, W. T., Jr. 1949. Copper availability test. Fla. Agr. Exp. Sta. Ann. Rpt. p. 192.
Forsee, W. T. 1950. Spray and dust trials with nutritional mixtures applied at various concentrations and formulated from various sources of manganese and zinc compounds. Fla Agr. Exp. Sta. Ann. Rpt. p. 191–192.
Forsee, W. T., and J. C. Hoffman. 1948. Fertility investigations under field and greenhouse conditions: celery. Fla. Agr. Exp. Sta. Ann. Rpt p. 189.
Forsee, W. T., E. A. Wolf, and W. A. Hills. 1950. Soil fertility investigations under field and greenhouse conditions. Fla. Agr. Exp. Sta. Ann. Rpt. p. 180–183.
Forsee, W. T., E. A. Wolf, R. H. Webster, W. A. Hills, R. J. Allen, and N. C. Hayslip. 1952. Soil fertility investigations under field and greenhouse conditions. Fla. Agr. Exp. Sta. Ann. Rpt. p. 197–199.
Gascho, G. J., H. W. Burdine, and F. A. Taha. 1971. Availability of manganese in Everglades peat as influenced by soil pH, source, and rate of application. Soil Crop Sci. Soc. Fla. Proc. 31:106–109.
Geraldson, C. M. 1953. Field control of blackheart of celery. Proc. Fla. State Hort. Soc. 66:155–159.
Guzman, V. L. 1969. Celery yield and quality in relation to methods of fertilizer application and climate. Soil Crop Sci. Soc. Fla. Proc. 29:301–305.
Guzman, V. L., H. W. Burdine, E. D. Harris, J. R. Orsenigo, R. K. Showalter, P. L. Thayer, J. A. Winchester, E. A. Wolf, R. D. Berger, W. G. Genung, and T. A. Zitter. 1973. Celery production on organic soils of south Florida. Fla. Agri. Exp. Sta. Bull. 757. 79 pp.
Guzman, V. L., C. A. Sanchez, and R. T. Nagata. 1989. A comparison of transplanted and direct-seeded lettuce at various levels of soil fertility. Soil Crop Sci. Soc. Fla. 48:26-28.
Hanlon, E.A., and G.J. Hochmuth. 1992. Recent changes in vegetable phosphorus and potassium recommendations for tomato, pepper, muskmelon, watermelon, and snapbean in Florida. Commun. Soil Sci. Plant Anal. 23:2651–2665.
Hanlon, E.A., G. Kidder, and B.L. McNeal. 1990. Soil-test interpretations and recommendations. Fla. Coop. Ext. Serv. Circ. No. 817. 49 p.
Hochmuth, G. J., and E. A. Hanlon. 1989. Commercial vegetable crop nutrient requirements. Fla. Coop. Ext. Serv. Circ. 806. 8 pp.
Hochmuth, G. J., D. N. Maynard, C. Vavrina, and E. A. Hanlon. 1991. Plant tissue analysis and interpretation for vegetable crops in Florida. Fla. Coop. Ext. Serv. Spec. Ser. SS-VEC-42. 62 pp.
Hortenstine, C. C., and W. T. Forsee, Jr. 1960. Research and application of soil testing for organic soils. Soil Crop Sci. Soc. Fla. Proc. 20:363–370.
Kretschmer, A. E., and W. T. Forsee. 1964. The use and effectiveness of various copper bearing materials for application to Everglades organic soils. Soil Sci. Soc. Amer. Proc. 18:471–474.
Locascio, S. J., and J. G. A. Fiskell. 1988. Vegetable needs for micronutrients in perspective. Soil Crop Sci. Soc. Fla. Proc. 47:12–18.
Lockhart, J. M., and C. A. Sanchez. 1989. Acidification of an Everglades Histosol using several fertilizer sources. Proc. Fla. State Hort. Soc. 102:344–345.
Lucas, R. E., and J. F. Davis. 1961. Relationship between pH values of organic soils and availabilities of 12 plant nutrients. Soil Science 92:177–182.
Sanchez, C. A., and H. W. Burdine. 1989. Soil testing and plant analysis as guides for the fertilization of escarole and endive on Histosols. Soil Crop Sci. Soc. Fla. Proc. 48:37–40.
Sanchez, C. A., R. T. Nagata, and V. L. Guzman. 1989. Response of sweet corn to nitrogen source and rate on Histosols. HortScience 24:925–927.
Sanchez, C. A., H. W. Burdine, and V. L. Guzman. 1990a. Soil testing and plant analysis as guides for the fertilization of celery on Histosols. Soil Crop Sci. Soc. Fla. Proc. 49:69–72.
Sanchez, C. A., S. Swanson, and P. S. Porter. 1990b. Banding P to improve fertilizer use efficiency of lettuce. J. Amer. Soc. Hort. Sci. 115:581–584.
Sanchez, C.A., and E.A. Hanlon. 1990. Evaluation of selected phosphorus soil tests for lettuce on Histosols. Commun. Soil Sci. Plant Anal. 21:1199–1215.
Sanchez, C. A. 1990. Soil-testing and fertilization recommendations for crop production on organic soils in Florida. Fla. Agr. Exp. Sta. Bull. 876. 44 pp.
Sanchez, C. A., P. S. Porter, and M. F. Ulloa. 1991b. Relative efficiency of broadcast and banded phosphorus for sweet corn produced on Histosols. Soil Sci. Soc. Amer. J. 55:871–875.
Sanchez, C. A., H. Y. Ozaki, K. Shuler, and M. Lockhart. 1991a. Nitrogen fertilization of radishes on Histosols: response and 15 N recovery. Hort Science 26:865–867.
Stephens, J. M., and B. D. Thompson 1963. The effect of nitrogen, potassium, and moisture levels on the yield and quality of radishes (Raphanus sativus L.) grown on organic soils. Proc. Fla. State Hort. Soc. 76:139–142.
Townsend, G. R. 1936. Zinc sulfate sprays for vegetable crops. Fla. Agr. Exp. Sta. Bull. 488. p. 1–2.
Westgate, P. J. 1951. Preliminary results and observations on blackheart of celery. Proc. Fla. State Hort. Soc. 64:87–92.
Westgate, P. J., W. G. Blue, and C. F. Eno. 1954. Blackheart of celery and its relationship to soil fertility and plant composition. Proc. Fla. State Hort. Soc. 67:158–163.
Tables
Water-extractable P soil-test values and fertilization recommendations for several vegetables on Histosols.
Acetic acid soil test K values and fertilization recommendations for several vegetables on Histosols.