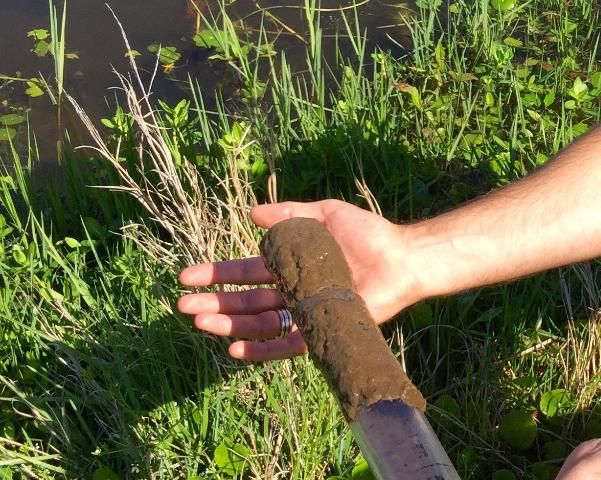
Credit: Mark Hoyer, UF/IFAS
Informational Circular 112
Florida LAKEWATCH
School of Forest, Fisheries, and Geomatics Sciences, Program in Fisheries and Aquatic Sciences
UF/IFAS
Gainesville, Florida
January 2017
This publication was produced by:
Florida LAKEWATCH 2017
UF/IFAS
School of Forest, Fisheries, and Geomatics Sciences, Program in Fisheries and Aquatic Sciences
7922 NW 71st Street
Gainesville, Florida 32653-3071
Phone: 352/392-4817
FAX: 352/392-3672
E-mail: lakewat@ufl.edu
Website: https://lakewatch.ifas.ufl.edu
Copies are available for download from the LAKEWATCH website: https://lakewatch.ifas.ufl.edu/extension/information-circulars/
Introduction
Muck is both the popular and the scientific term for the material found on the bottom of many depressions that have held water for any length of time. It is of great importance to many recreational lake users because its presence can ruin their enjoyment of the water body. Its dark color and "oozy" feel lead many people to believe muck could be an indicator of a polluted system.
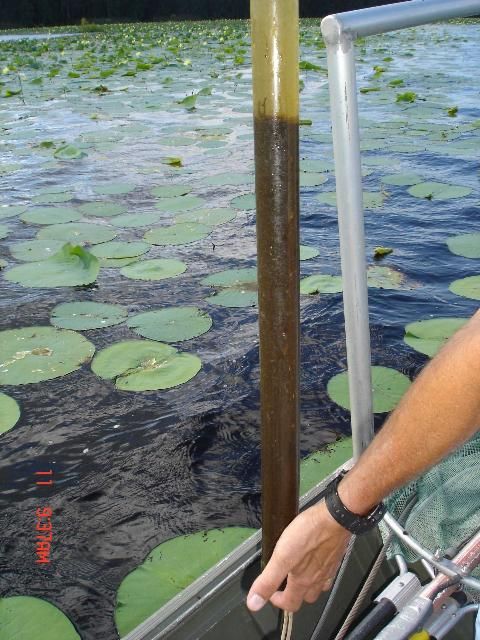
Credit: Florida LAKEWATCH
Another cause for people's distaste for muck is the stink. Bacterial decomposition of the organic matter in muck can release gases such as methane and hydrogen sulfide (often called marsh gas or sewer gas), the latter possessing a characteristic rotten egg odor. Adding to the list of problems, larvae of occasionally problematic insects such as mayflies and blind midges live in muck and often emerge as adults in swarms prior to mating. These swarms can create nuisances by coating lights, equipment, homes, roads, and bridges. The insects, especially blind midges, also swarm around people and are often confused with mosquitoes. The midge adults (flies), however, do not bite, suck blood, or carry diseases like true mosquitoes do, so they are simply a nuisance, rather than a health hazard.
It may seem simple: get rid of the muck; get rid of the problems. However, there is more to this muck-raking story. Excessive amounts of muck in the wrong places certainly can cause problems, but just enough muck in the right places is essential for a healthy lake that supports diverse wildlife and fishing. That's because, in addition to midge and mayfly larvae, muck provides habitat for numerous other aquatic organisms called macroinvertebrates (Pennak 1953; e.g., other insect larvae, leeches, worms, snails, mussels). These organisms provide food for a variety of fish, insects, amphibians, and water birds. In Florida, aquatic insects and other invertebrates are extremely important food sources for the recreational bream (i.e., bluegills, redear sunfish) and speckled perch (black crappie) fisheries in lakes. Thus, the goal for resource managers is not to eliminate muck entirely, but to keep the right amount of muck in the lake for proper ecosystem function and human uses of the water body.
Striking the proper balance for each lake can be problematic because each system is distinct, with different ecological characteristics and designated human uses. Some waters naturally have little accumulated muck, whereas others have a lot. Some have muck only in deep-water areas, but display sandy beaches and muck-free shallow zones. How old the basin is, i.e., how long it has continuously held water, and whether it has input streams or rivers, influences the quantity of bottom muck. In fact, many factors conspire to determine the amount of muck on a lake bottom, but for many lakeside dwellers and lake users, the only question about a lake with substantial muck accumulation is: "How do I get rid of the stuff?"
Considering the complexities that face Florida communities and individuals tackling muck issues, this publication explains what muck is and what determines its distribution, provides an understanding of the origins of muck in aquatic systems and the ontogeny (individual development) of aquatic systems, and lists possible corrective actions, including approaches for muck removal. So if you say "ew," "ick," or "yuck" when your toes encounter muck, this publication will help you learn more and perhaps make partial peace with this richly complicated, if sometimes off-putting, natural material. Before you begin, however, we encourage you to peruse the following LAKEWATCH circulars to obtain a more comprehensive understanding of how aquatic systems function:
Circular 101: A Beginner's Guide to Water Management—The ABCs
Circular 102: A Beginner's Guide to Water Management—Nutrients
Circular 103: A Beginner's Guide to Water Management—Water Clarity
Circular 104: A Beginner's Guide to Water Management—Lake Morphometry
Circular 109: A Beginner's Guide to Water Management—Oxygen and Temperature
Circular 110: A Beginner's Guide to Water Management—Fish Communities and Trophic State in Florida Lakes
Circular 111: A Beginner's Guide to Water Management—Aquatic Plants in Florida Lakes
These publications can be downloaded free from the Florida LAKEWATCH website: https://lakewatch.ifas.ufl.edu/extension/information-circulars/.
Part 1: What is muck?
Muck, to the non-scientist, is nothing more than the black or brown ooze on the bottom of lakes. The scientific community, on the other hand, distinguishes among various types of muck, depending on their origin and composition. One common characteristic, however, is the presence of a large amount (typically 20% to >80%) of organic matter.
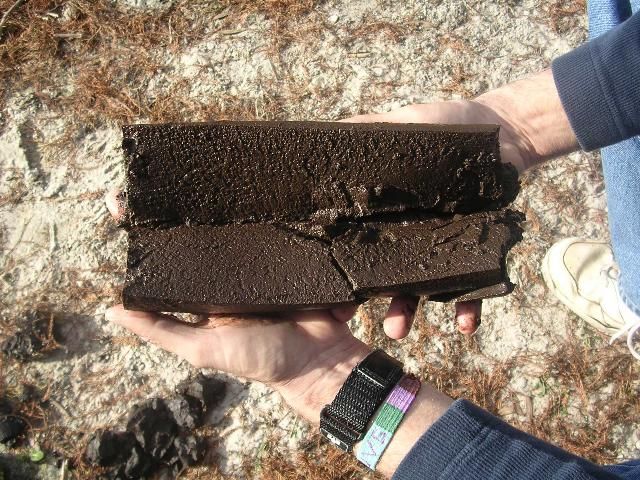
Credit: David G. Buck
Sources of Organic Matter
Organic matter in muck is composed of plant and animal residues. In aquatic systems, the organic matter comes from either outside or inside the water body. Organics that originate outside the aquatic environment (e.g., soils, plant leaves, pollen grains, and grass clippings that wash into the lake) are called allochthonous materials. Organics originating inside the aquatic system (e.g., aquatic plants that grow and die within the waterbody, algae that settle on the bottom, zooplankton remains, and aquatic animal feces) are called autochthonous materials.
When considering the muck on the bottom of lakes, ponds, wetlands, and other aquatic systems, one must remember that bottom sediments, including muck, are composed of a mix of allochthonous and autochthonous materials. The allochthonous component typically consists of soil with differing amounts of organic material, as well as leaves, grass blades and other organic material carried by inflowing water. Allochthonous components may also enter an aquatic system as wind-borne material, like leaves/needles, seeds, twigs and pollen. Autochthonous muck components, on the other hand, originate from algae, large aquatic plants (both submerged and emerged), dead zooplankton, insects, fish and other animals, and their feces. Autochthonous materials can also contain biogenic silica (essentially "glass") produced by algae called diatoms and freshwater sponges. In some lakes, minerals like calcium carbonate are precipitated from the water column and accumulate on the lake bottom.
Types of Muck
Not all muck is the same. The sources (allochthonous or autochthonous) of organic material influence the type of muck present in an aquatic system, but muck type is also influenced by its percent organic versus inorganic matter content, the size of the individual sediment particles, and the amount of time it has had to decay.
Interest in lake muck first arose because farmers recognized its value to agriculture when lakes were drained. The "reclaimed" land from drained lakes formed the economic base for many communities during the early development of the United States and "reclaiming" wetlands to create productive farmland became a political imperative in wetland-rich states like Florida. The Internal Improvement Act of 1855 initiated Florida's efforts to reclaim vast amounts of land by constructing canals, dikes, and pump stations. The reclaimed soils were highly valued by early agriculturalists and are still used for many crops, such as celery, carrots, cabbage, sweet corn, and sugar cane (Coale et al. 1994). Those who cultivate on these soils in Florida became known as "muck farmers."
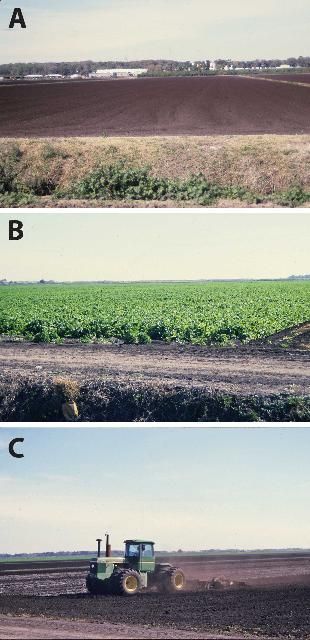
Credit: Gregory Macdonald
Early American soil scientists who worked at the end of the 19th century classified wet soils into two categories: 1) muck and peat, and 2) swamp, tidal swamp, and marsh.
- Muck and peat soils were described as composed largely of organic matter in various conditions of decay, with the muck representing an advanced stage of transformation in peat areas. It was recognized that these soils were relatively limited in extent and poorly drained, but good for agriculture.
- Swamp, tidal swamp, and marsh soils were those covered with water for the greater part of the year and unfit for agriculture, except where drained and protected from tidal or fluvial overflow. When reclaimed, much of this land could become very productive.
Early soil scientists were only interested in wet organic soils for their agricultural potential. Reclamation simply involved draining the water so that normal agricultural methods could be used to farm. If not drained, such water-covered lands were deemed worthless. Over time, however, as people began to understand the importance of wetlands, society began to recognize wetlands as valuable natural resources. By the end of the 20th century, American soil scientists, after examining soil profiles from sites all over the continental United States, had developed a comprehensive soil classification system (Evett and Cheng 2007).
Beginning in the late 19th century, limnologists (scientists who study inland waters) were developing a classification system for bottom sediments in aquatic systems. Limnologists, especially in Europe, began their studies, not for "land reclamation," but rather to understand the role of sediment in the functioning of aquatic ecosystems. Sediment corers and hand dredges (e.g., Ponar and Ekman dredges) were the primary tools used to collect sediment samples. Paleolimnologists (scientists who study ancient inland waters) extracted long sediment sequences with specialized corers, to determine past environmental conditions and predict the trajectory an ecosystem in the future. These efforts, however, resulted in a new vocabulary to describe the organic/mineral sediments that accumulate on the bottom of aquatic ecosystems. Though there are differences between the classification systems used by soil and aquatic scientists to describe soil and sediment, respectively, there is some overlap.
All soils originate from a base, geologic material. Geologists have standardized how they report the size of inorganic soil particles (1 inch = 25.4 millimeters (mm), and 1 mm = 1000 microns):
- Boulder: 256 (mm)
- Cobble: 64 mm
- Pebble: 2 mm
- Sand: 62 microns
- Silt: 4 microns
- Clay: 1 micron
These size categories are simplified from what is called the WENTFORD Scale of Grade Sizes (Strahler 1975).
For soil scientists, soil profiles are composed of a combination of sand, silt, and clay. Non-scientists who live around water recognize near-shore soils with a large percentage of sand as sand beaches, those with a high percentage of clay as gumbo, and soils with a high percentage of silt as a type of muck. Silt may be in muck, but by itself it is not muck because it has no organic component. Rather, it is made up of rock and mineral particles (inorganic) that are larger than clay but smaller than sand. It is a solid, dust-like sediment that water and wind (ice in northern and mountainous areas) can transport and deposit.
The United States Department of Agriculture's Natural Resources Conservation Service (formerly Soil Conservation Service) now classifies all soils saturated with water, including those now drained, as hydric soils (Soils Survey Staff 1999). Wet soils with lots of organic matter are now labeled as muck, mucky-peat, and peat:
- Muck is defined as an organic soil material in which virtually all the organic material is decomposed, precluding identification of plant forms. It is also called sapric organic material.
- Mucky-peat is an organic material with decomposition of the organic component that is intermediate between that of muck and peat. When rubbed between the fingers, some fibrous material from plants is observed. It is also called hemic organic material.
- Peat is a fibrous organic soil material in which many plant fibers are observed when it is rubbed. Plant forms making up the peat can also be identified. It is also called fibric organic material.
From their early studies, limnologists, like soil scientists, recognized that lake sediments were derived from both external (allochthonous) and internal (autochthonous) sources. They concluded that most of the sediment came from the water body's drainage basin but that the presence of dead organic matter and chemical precipitates such as carbonates and diatom frustules produced within the aquatic system could be extremely important at different points in the developmental history (ontogeny) of the water body. In some lakes, they found distinct light and dark layers, called laminations, in sediment. If couplets (a light and a dark layer) were deposited within an annual cycle, they were referred to as varves. Through the years, several bottom-sediment classification systems have been developed. For instance, the Troels-Smith Scheme was developed in 1955, and describes the components of the sediment, its physical properties, and the degree of humification, or decay of organic matter, in the sediment. Developed for temperate lakes in Europe and published in German, the scheme has not been widely adopted in the United States. More recently (2003), Doug Schnurrenberger and colleagues created a classification scheme based on the sediment components (Schnurrenberger, Russell, and Kelts 2003).
Sediments in aquatic systems consist of three primary components: 1) organic matter in different decomposition states, 2) mineral matter (clays, carbonates, and silicates), and 3) inorganic components of biological origin (e.g., diatoms, snail shells, sponge spicules). After analyzing the characteristics of many different types of lake sediment, limnologists, like geologists and soil scientists, recognized that the particle sizes of both inorganic and organic sediments are of major importance. Furthermore, they recognized that the bottom sediments of aquatic systems were affected by biotic interactions (changes caused by living organisms), the presence or absence of oxygen, and water movement. Consequently, they coined a plethora of new words to describe different types of bottom sediment.
Words used by limnologists or other aquatic scientists to describe sediments now include 1) rock (i.e., bed rock, boulders, rubble, gravel), 2) sand, 3) clay, 4) silt, 5) marl, 6) gyttja, 7) dy, 8) sapropel (faulschlamm, hot mud), 9) detritus, 10) fibrous peat, 11) pulpy peat, 12) muck, 13) fluid mud, and many more:
- Rock, sand, clay, and silt are essentially separated using the size-class definitions developed by geologists. Most rock components in Florida, unless imported from elsewhere by humans, are derived from the state's underlying base limestone. Clay is important in some areas of Florida where geologic formations are composed of clay and sand.
- Muck, fibrous peat, and pulpy peat are distinguished, for the most part, using the definitions developed by soil scientists. These materials are very common in Florida because much of the state had extensive wetlands before development.
- Marl is calcium/magnesium carbonate sediment, precipitated biogenically in some cases by plants and animals. It tends to occur in waters rich in dissolved calcium and magnesium and is often associated with marl-forming algae (e.g., Chara sp., also called stonewort) or rooted aquatic plants. It is typically white to grey in color and is often found underlying deep muck deposits.
- Gyttja is an organic sediment containing remains of particulate organic matter, inorganic precipitates and other mineral material. It is soft and contains large amounts of water (hydrous) with a dark grayish green to black color in its fresh state. The organic matter content of gyttja is <50%. Geologists describe it as a coprogenous organic sediment (which means that it is formed mainly from small animal fecal pellets).
- Dy is a gyttja mixed with humic colloids (a dark-brown or black organic substance made up of decayed plant or animal matter). Colloids are particles that do not settle and cannot be separated out by ordinary filtering or centrifuging. Fresh dy is hydrous and brown with an organic content >50%.
- Sapropel is a foul-smelling, gel-like neutral (non-acid) humus formed in the presence of iron sulfide under anaerobic (no oxygen) conditions. It contains large amounts of hydrogen sulfide (the rotten egg smell) and methane gas that often form gas pockets in the sediments. It is occasionally called "hot mud" colloquially because when people step into the gas pockets, they sometimes feel a burning sensation on their feet and legs. When dried, the sediment will harden and exhibit many cracks.
- Detritus is dead particulate organic material (as opposed to dissolved organic material) that includes the bodies or fragments of dead organisms as well as fecal material. (Note, to confuse matters, geologists define detrital material as that created by the breakdown of rocks, i.e. inorganic matter).
- Fluid mud is often defined as a mixture of fine sediments with water that has practically no shear strength. Shear strength defines the material's ability to resist forces that can cause the internal structure of the material to slide against itself. Fluid mud layers were originally described for coastal systems, but have also been found in two of Florida's largest lakes, Apopka and Okeechobee. Fluid mud is formed by wave-induced liquefaction of a cohesive sediment bed in wave-dominated environments that can be easily pumped (Bachmann et al. 2005).
Conclusion
What is muck? Muck is many things. It comes in many shapes, colors, and sizes, is composed of many different materials, is called many different names, and is defined many ways. The definition of muck most useful to communities and natural resource managers in the initial stages of dealing with the stuff is probably the least scientific one: muck is sediment that is sometimes located in amounts and places where people do not want it. Before acting on that definition and jumping into the process of removing unwanted muck, however, aspiring muck removers must consider the factors that influenced the amount and distribution of muck in the water body. Muck removal projects can be deemed failures if muck quickly reoccupies a cleaned area!
Part 2: Muck Distribution
Distribution within a water basin of sediment, including muck, is influenced by multiple, interacting factors including 1) the size of the watershed, 2) the surface area of the basin, 3) the shape and orientation of the basin, 4) water currents, 5) the configuration of the original and current basin depth profile, and 6) the size of the rooted aquatic plant zone (see Circular 104; Lake Morphometry). Despite possible complex interactions, two things are certain. First, water-holding basins act as natural sediment traps, and second, muck sediments settle at locations where water movement (hydrodynamics) lacks sufficient energy to move the sediment particles to a different location. Additionally, the faster the water movement, the larger the particles that can be transported.
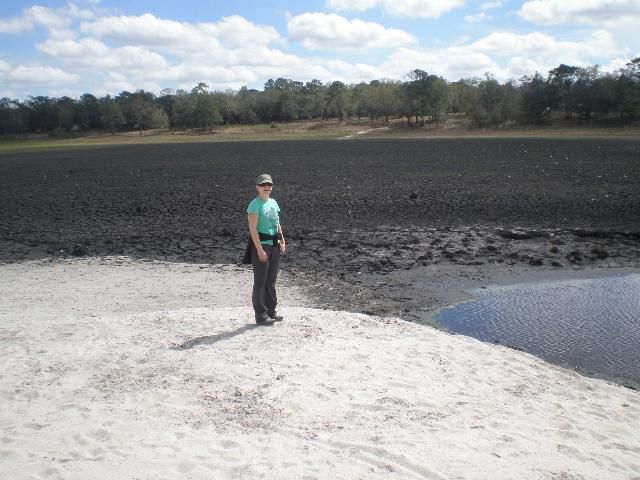
Credit: Mark Brenner, University of Florida
External Forces
When a basin (e.g., a lake or pond) first forms, the composition of the bottom sediments is strongly influenced by the geomorphology of the basin and the watershed. Geomorphology is the scientific study of the origin and evolution of topographic and bathymetric features (contours) created by physical, chemical, or biological processes operating at or near the Earth's surface. For example, Florida's many sinkhole lakes typically had sandy bottoms when first formed, but ponds created by fire (lightning strike fires in peat areas) in wetlands often have mucky bottoms.
Although most sediment in a basin reflects regional geology, biological processes in the watershed and in the pond or the lake itself modify the bottom deposits. Watershed influences are most noticeable and dominate if a stream or streams enter the basin. Much of the muck found on the bottom of these basins is composed of allochthonous inorganic and organic materials carried by the streams.
When water enters or leaves a lake, water movement occurs within the water body. Currents sort the sediment particles with respect to size, density, and the energy available for their transport. Larger particles settle to the bottom as currents begin to slow, and finer muck material is carried further and deposited in the deepest part of the basin, which usually has the slowest current, causing what is called sediment focusing. Sediment focusing is often apparent when sediment from a broad area is ultimately deposited in the small area of a water body's deep hole. In the life of a water body, it is common for deep-water areas to fill first and accumulate the most sediment.
For most shallow Florida basins, the dominant external force that affects sediment distribution is wind. The wind's impact, however, is influenced by the size, shape and orientation of the basin. If wind can blow across a large basin's surface unimpeded by a landform like an island or peninsula, large waves can develop. The part of the lake the wind blows over is called its "fetch." The longer the fetch and stronger the wind, the stronger the waves and the deeper into the water column the wave movement will reach. In such a situation, a sandy beach will likely occur at the downwind end of the fetch, and the shoreline will erode inland, sometimes causing major damage. This erosion zone is an area where muck does not accumulate. Muck does not accumulate because the wave action and the resultant water currents move the finer materials to deeper areas where the sediments are focused. This process occurs until all deep areas are filled, and then muck is relatively evenly distributed across the basin bottom.
Once the sediment muck layer accumulates to the point where the lake becomes so shallow that wave action reaches the sediments, material is re-suspended into the water column. Wind re-suspension can then move considerable amounts of bottom sediments and dramatically affect water quality. For example, Hurricane Wilma in 2005 increased the total suspended solids concentration in Lake Okeechobee, through the resuspension of bottom sediments, from 28 to 124 mg/L, a more than four-fold increase. Total phosphorus increased from 188 µg/L to 296 µg/L, a 57% increase. The hurricane also uprooted and dislocated aquatic plants from near-shore waters (Havens et al. 2007).
Internal Forces
To understand the internal forces that influence the distribution of muck in a basin, a good first step is to obtain a contour map (a bathymetric map) of water depths at different locations. Florida lakes come in different sizes and shapes, and lakes that originated as cone-shaped sinkholes typically have their deepest area near the middle of the lake. Other lakes, however, may have multiple deep areas, some of which hold considerable muck and are near the shore.
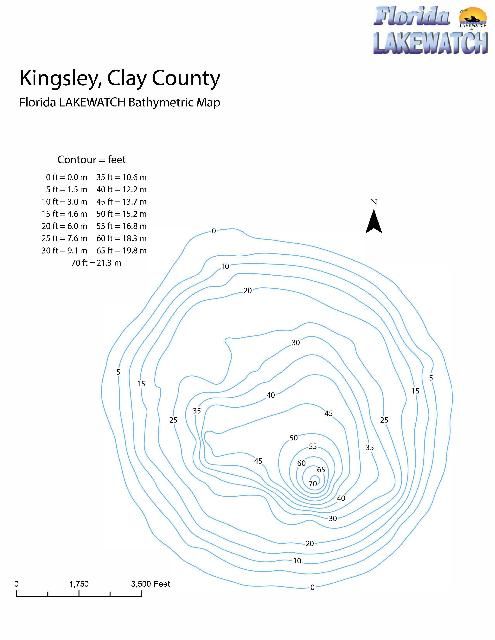
Credit: Florida LAKEWATCH
The slope of the lake bottom also has a tremendous influence on the distribution of muck particles. Lakes with steep bottom slopes typically accumulate little mud, especially if the slope is in an area where wave action reaches the bottom. Sometimes there are shallow underwater ridges that are free of muck because muck particles have been transported to deeper water on either side of the ridge. In lakes with shallow slopes, depth contours are widely spread apart, and muck particles tend to settle if there is no deep area nearby. Absent deep areas, muck tends to accumulate across the entire bottom of the pond or lake. If the water depth is such that the bottom sediments interact with waves, sediments will be mixed upward into the water column by wind re-suspension.
Muck can also accumulate in bays protected from the wind or on the leeward side of islands. Fallen trees along the shoreline can disrupt water movement, allowing muck to settle. Human structures such as docks and dead-end canals also create quiescent (motionless) zones where muck can accumulate (Whitmore et al. 1996).
There is perhaps no greater internal factor for determining the distribution of muck in Florida lakes than the presence of rooted submerged and emergent plants (aquatic macrophytes). These plants reduce water movement and allow particles to settle. Emergent macrophytes are particularly problematic for shoreline residents because they produce great amounts of organic matter and trap other fine sediments among their roots, allowing muck to build up and the shoreline to extend towards open water. This process is called shoreline accretion.
In Florida, aquatic plant management helps maintain the usability of many waters, especially when floating aquatic macrophytes like water hyacinths completely cover a water body. A major concern for most user groups is that aquatic plant control by use of herbicides adds organic matter to the basin bottom when the plants die. Although this is true, living, growing plants produce much more organic matter, often leading agencies like the Florida Fish and Wildlife Conservation Commission to implement major muck removal projects, through either dredging or the scraping of shorelines (Hoyer et al. 2008: e.g. Lakes Panasoffkee and Tohopekaliga).
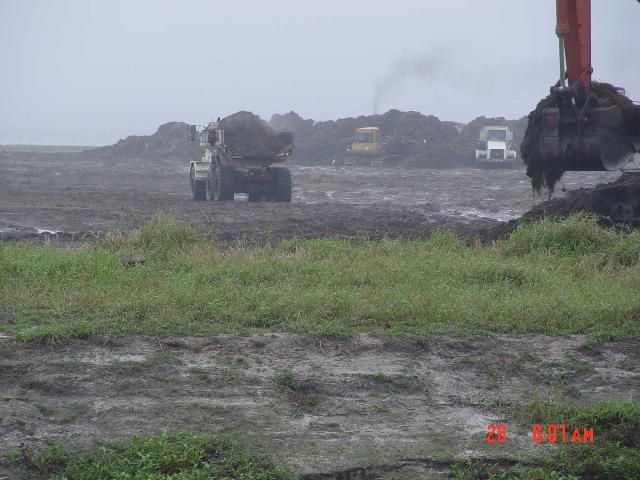
Credit: Florida LAKEWATCH
When an aquatic plant management program is implemented or a water body undergoes substantial nutrient enrichment, the most frequent question asked by the concerned citizen is "how much muck do the aquatic plants produce?" A definitive answer is difficult to provide because so many environmental factors determine muck accumulation rates, but generally, algae produce the least muck, submerged macrophytes produce a little more than algae, and emergent, floating-leaf, and floating plants produce the most muck.
The amount of muck accumulated on the bottom of a basin depends, in part, on the age of the basin. The first plants to colonize newly formed lakes and ponds are suspended algae (phytoplankton). New waters often display high biological productivity as nutrients are leached from the watershed soil. The algae typically produce an algal gyttja, ultimately converting the bottom sediments from a more mineral-rich deposit to an increasingly organic-rich sediment. The rate at which sediment thickness increases is dependent, in part, on the productivity in the water column. For nutrient-poor (oligotrophic) waters, the sediment accumulation rate is generally less than 0.3 mm per year, but in nutrient-rich (eutrophic) waters the rate approaches 1 mm year. Waters receiving nutrient-rich sewage have rates approaching 10 mm per year! Organic material arising from suspended algae typically does not accumulate rapidly because organisms higher up the food web readily eat algae, and the organic material is thus easily decomposed (Hoyer et al. 2016).
Sediment accumulation rates change dramatically when the water becomes shallow enough to support extensive growths of aquatic macrophytes, especially peat-forming macrophytes like bulrush (Scirpus spp.), cattails (Typha spp.), and water hyacinth (Eichhornia crassipes). Rates change in large part because many of these macrophytes are rooted in the bottom sediments where nutrients can be extracted, and because they support the growth of attached algae (periphyton). Within the plant beds, oxygen concentrations just above the bottom sediments are often depleted, permitting organic matter to accumulate rapidly, ultimately transforming the water body into a wetland. In Florida, wetlands accumulate peat at rates on the order of centimeters per year.
The transition from open water to a wetland, however, is not unidirectional because there are both natural events (e.g., droughts, fires, and water level fluctuations) and human activities (e.g., dredging, water drawdowns) that will reset a water body's long-term trajectory.
Conclusion
Of all the factors that influence muck distribution, lack of water movement is most important. In Florida, basin depth and the presence of aquatic macrophytes are perhaps the two most important natural factors that influence muck distribution, but the construction of structures like docks can also induce muck accumulation by reducing currents. These basic factors are important to remember because the location of a nearby deep area can not only impact sediment distribution, but provide a concentration point where muck can be targeted and continually removed.
Part 3: The Ontogeny of Aquatic Systems—Mother Nature and Time
No lake can last forever because the basin will ultimately fill with sediment. The life expectancy of a lake or pond can be very short or long on a geologic time scale. Most basins will go through different successional stages during their life. These changes, however, need to be placed in a temporal context with respect to the relatively small number of years that humans have interacted with the water body.
Limnologists once discussed the "aging" of lakes and their successional stages (lake ontogeny) as a unidirectional process, which ultimately leads to the lake's disappearance by infill. The original lake ontogeny concept had the lake starting as a biologically unproductive (oligotrophic) system with a limited amount of organic matter on the bottom. As this oligotrophic system aged, organic matter accumulated on the bottom, reducing lake depth. The system became more biologically productive, and the trophic status of the basin shifted from oligotrophic to mesotrophic and then to eutrophic and hypereutrophic as the aging process continued. The process was thought to speed up when humans added nutrients to the water (cultural eutrophication) via the disposal of sewage or fertilizer input from the watershed. Ultimately, the lake would evolve into a wetland and then a terrestrial system as the basin filled completely with organic matter.
Ontogeny of Florida Aquatic Systems
Often, riparian landowners in Florida comment that when they bought their property, the nearshore area was free of muck and aquatic plants, but the shoreline is now plant-filled and mucky. It is a situation that they cannot understand because sources of pollution are not easily identified. The situation may relate, in part, to Florida's natural wet/dry cycles. Precipitation over many consecutive years can be above the long-term average, causing water levels to rise. Alternatively, multiple years of below-average rainfall will cause water levels to decline.
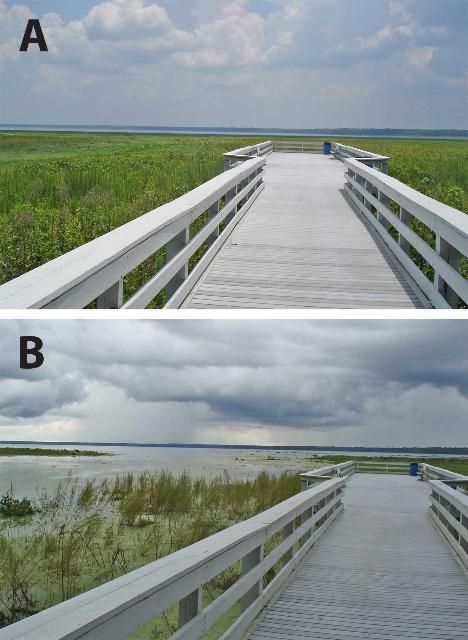
Credit: Mark Hoyer, UF/IFAS
Florida is a land of floods and droughts. Although Florida has more than 7,700 lakes and innumerable ponds, many of these water bodies are shallow. During severe droughts, some of these lakes and ponds experience severe reductions in water level and, in some cases, go completely dry. In central Florida, many shallow lakes dried completely in the 1950s, and again during the first decade of the 21st century. Severe drought, when sufficiently long, permits drying of the bottom muck. Dried muck consolidates and, when exposed to strong winds, is blown from the basin, often exposing a sand bottom. This consolidation and loss of muck is one reason why fish and game organizations advocate the use of water-level drawdowns to improve fisheries. If sufficient drying takes place, the muck can be mineralized or swept away by strong winds.
Extreme Floods
Besides being known for its periodic droughts, Florida is also the lightning capital of the United States. Sometimes when lightning strikes the dried muck in a basin, a so-called "muck fire" can be started. Muck fires can burn below the surface and continue for long periods once buried, decomposing vegetation is ignited. Muck can also combust spontaneously during vegetation decomposition. Heat builds up to the point where it can ignite drier material adjacent to the area of decomposition.
Muck fires can burn for weeks or sometimes months, and are extremely hard to extinguish. Many muck fires smolder underground, creating smoke and odors that can persist until heavy rains clear the air and re-flood the basin. Occasionally, the subterranean fires work their way to the surface and ignite above-ground material. Burning muck often lowers the ground elevation enough to deepen the basin, and convert swamps into lakes or ponds. Muck fires, therefore, can reverse the normal ontogeny of a water body and clean shorelines to the point that a new riparian land owner will be unaware of the natural lake condition. Mother Nature and time will reestablish muck conditions that may then require human intervention to restore the usability of the aquatic system.
Mother Nature and time can also redistribute muck in shallow Florida systems when water levels rise after droughts. If a lake's water level is lowered sufficiently for plants to root but not to the point where the muck dries out, aquatic and terrestrial plants may root in the muck. Given sufficient time for the root systems to develop, floating islands (tussocks) will form when the water level rises. Wind can then push the islands over great distances. When that happens, muck can be deposited at locations far from where it was formed when the tussock settles or disintegrates. If the water rises sufficiently to flood the land beyond the shoreline, tussocks can float landward of the traditional shoreline. If these tussocks originated in shallow, nearshore waters, a sandy bottom will be seen when the water returns to its normal stage. This is another reason why some professionals do not recommend maintaining stable lake water levels. The primary question is how long people will wait for Mother Nature and time to do their thing!
Part 4: Muck Removal
Floridians have been removing muck from aquatic basins for more than 150 years. Ten years after Florida became a state in 1845, the General Assembly passed the Internal Improvement Act. The preamble of the act stated: The Constitution of the State declares that a liberal system of internal improvements being essential to the development of the resources of the country, shall be encouraged by the government of this State and it shall be the duty of the General Assembly, as soon as practicable, to ascertain by law proper objects of improvements in relation to roads, canals and navigable streams, and to provide for a suitable application of such funds as may be appropriated for such improvements. Dredging was used to create canals and deepen waterways for draining and reclaiming the land. Land reclamation was promoted to open land for agriculture and help Florida develop a strong economic foundation.
Dredging canals and access points also provided early Florida the needed transportation network for both commercial and military uses. As Florida continued to develop and the population grew, dredging was used to create new land to support the building of waterfront property. Housing became a major economic driver. With this construction activity, additional canals were dredged for flood control and for water conservation during droughts. Massive water control structures and numerous locks and dams were built in association with this activity.
Dredging, along with air conditioning and mosquito control, allowed for the development of Florida. Without it, it would not be the state that we know today. Before the 1970s, dredging could be done by virtually anyone with equipment and money. As Florida's population expanded, however, concerns arose regarding the appropriateness of dredging practices and the draining of wetlands. Because of these concerns and others, the state of Florida and the US Army Corps of Engineers were given the authority to issue permits for dredging activities. Given concerns about the destruction of wetlands, many Floridians continue to look upon dredging unfavorably (Rey et al. 2012).
Dredging, however, is still needed in some areas of Florida to maintain the usability of water bodies because sediments, including muck, continue to accumulate. Dredging is the only management approach that guarantees the removal of muck. Effective and sustainable dredging becomes increasingly challenging as the human footprint on the Florida landscape becomes increasingly massive. The biggest challenge for dredging projects is finding methods to dispose of dredged sediments cost-effectively.
Large-Scale Dredging
Major dredging projects in Florida involve excavation. Such projects use several types of heavy equipment. Dredging approaches can include grab dredging with a clam-shell bucket, dragline dredging, and suction dredging. Grab dredging, dragline dredging, or other mechanical approaches require adjacent land for disposal of the sediments, an adjacent barge hopper that can transport the collected sediment to a deep-water disposal site, or trucks that can transport material to distant land disposal sites. Unless the sediment can be spread on adjacent land, transportation is a major cost. In the case of suction dredging, the bottom sediments are vacuumed from the bottom either directly or after being disrupted by a cutter head. The sediment disposal site can be located at considerable distance from the dredge area because the slurry is pumped to a large settling basin. Pumping over long distances and land costs for the settling basin are important economic considerations for this type of excavation activity.
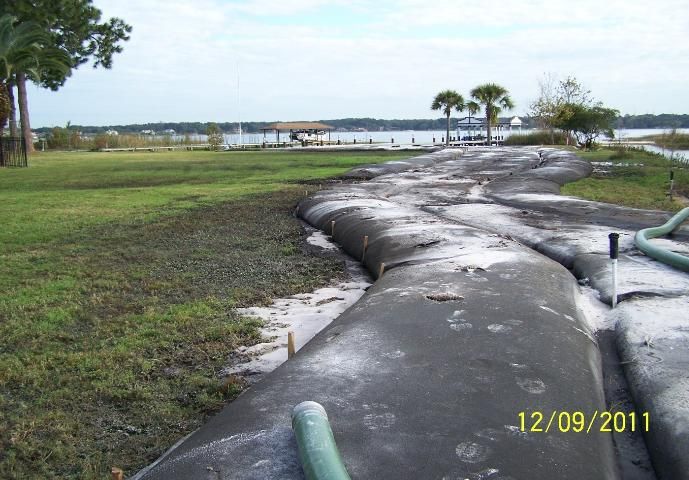
Credit: Sergio Duarte
The sediment slurry, once pumped into the settling basin, is allowed to settle, and the water, once cleaned of sediments to meet water quality standards, can often be returned to an aquatic system, unless it has been totally evaporated. Lack of land or the high cost of land in an urban area can preclude the use of large-scale suction dredging. If the water level in a water body can be lowered sufficiently to allow nearshore bottom sediments to dry, bottom muck can be scraped down to hard bottom using conventional construction equipment (i.e., bulldozers, track hoes, and trucks) and piled up to form in-lake islands. This approach was used at Lake Tohopekaliga. Creating multiple islands greatly reduced the cost of sediment disposal (Hoyer et al. 2007).
Large-scale dredging projects, however, tend to cost many millions of dollars and are typically carried out under the direction of the federal and/or state government. These excavation projects need strong political support to overcome the many logistical, permitting, and political barriers to dredging. Consequently, the time between the first proposal to dredge and the start of dredging may be many years.
Small-Scale Dredging
Local government agencies or homeowner associations often sponsor dredging projects. These projects are typically small-scale and cost less than a million dollars because a limited amount of material is removed. Excavations may be conducted using readily available road maintenance equipment like track hoes and back hoes, with trucks transporting the material away, or with specialized muck removing equipment (e.g., walking/spider excavators) that can operate in the water.
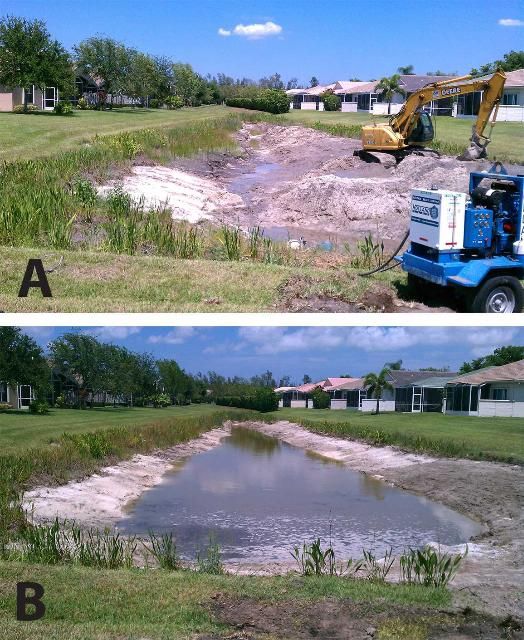
Credit: Allstate Resource Management Inc.
For some situations, smaller hydraulic dredgers can be employed. Sediment disposal is often accomplished by placing muck into an on-land container called a geotube. A geotube container is a specially designed geotextile fabric for dewatering dredge slurries. The geotube retains the sediment but allows water to exit and return to the system. Sediments are held within the bag until dry (often weeks to months) and then removed by truck. Geotubes are expensive, and because preparation costs of using geotubes are high (i.e., grading land before and after geotube placement, and establishment of performance bonds), new approaches are being developed.
By the start of the 21st century, efforts were undertaken to develop rapid sediment dewatering systems that could operate within a small land footprint, and return water directly to the system from which sediments were removed. The primary problem with existing sediment dewatering systems is the inability to rapidly dewater the residual fine sediments that remain at the end of the treatment process. Fine sediments increase the turbidity of the return water to the point that it often exceeds water quality standards, thus delaying not only the return of the treated dredge water, but reducing the amount of time the dredge can operate. To overcome this problem, the industry now uses different polymers to coagulate the fines into larger particles and vertical geotube equipment like the Dewatering Tower patented by Blue River Technologies of New Castle, Indiana, to remove the residual sediment. Other approaches that use self-cleaning screens are also being developed and could ultimately lead to a cost-effective, small-footprint dredging system (Allhands 2005).
The Do-It-Yourself Approach
Many individuals confronted with a muck problem become frustrated because most muck removal approaches mentioned above are too expensive and take too long to implement. Some other approaches are applicable in small areas, but before starting any muck removal program in Florida, determine whether a permit is required from the proper State Agency.
One common technique uses water currents to move the muck into deeper water, if such a deep-water area is available. Several products are available to the homeowner that generate sufficient currents to move sediment from dock and beach areas, including rotating electrical blowers like Aqua-sweep™ or Muck Blaster™, which continuously suspend muck and enable currents to move it into deep water. If there is sufficient water current, rotating sweeps, which also reportedly remove aquatic plants by disturbing the bottom sediments, enable water currents to move the muck to deeper water. The cost of this type of muck management varies depending on the size of the area being managed, the cost of equipment, and operational costs for electricity.
Sometimes people attempt to remove muck by hand using special rakes, shovels or hand dredges. This tedious, physically taxing approach is certainly not recommended for older individuals. It is sometimes feasible to use shore-based portable trash pumps to vacuum the muck out or even suction muck and plants out with the assistance of a diver (another young person's job). These approaches are now more viable because small, self-cleaning, wedge-wire screens are available for rapidly dewatering the muck. The dried muck can be carted away quickly, and the water returned over the yard to the water body.
Keeping muck under control requires fairly arduous and unpleasant effort, and many people understandably seek an approach that requires less or no work. There is no such work-free approach, unfortunately, but if you keep searching, sooner or later somebody will suggest you try using aeration and bacterial additions—beneficial bacteria, they say, will "eat up the muck." Proceed with caution if you try this approach, and be prepared for unimpressive to disastrous results: although this approach is supported by testimonials, as yet there is little scientific evidence to suggest it is effective.
Aeration is a crucial component of this approach and promoters point out that addition of oxygen to a water body stimulates the aerobic bacteria that are added. It is true that the addition of oxygen to a pond or lake can be good for animals like fish, but there will already be plenty of aerobic bacteria in any water body with normal levels of oxygen. The few scientific studies on the role of aeration in treatment of muck seem to show that any muck reduction is likely related to the redistribution of the bottom material, and not bacterial metabolism. The redistribution of bottom muck is related to water currents generated by the aerators, which move material to deep water.
The failure of added bacteria to remove deep muck deposits is related to the inability of oxygen to penetrate deep below the sediment surface. Deeper oxygen penetration will only occur if the muck is stirred and re-suspended into the water column, an undesirable side effect because it makes the water murky. Furthermore, massive re-suspension of anaerobic sediments can consume oxygen in the overlying water column, with predictable negative consequences for fish and other aquatic fauna.
There is also a fundamental problem regarding how much of the muck is biodegradable. The most readily decomposed material in a water body is the organic matter produced by algae. Most muck sediments have an organic content less than 50%. For waters that do not receive substantial inputs of treated municipal wastewater, sediment accumulation on the bottom is on the order of millimeters per year. Even if 90% of the material were biodegradable, the thickness of the muck would only decrease by a few millimeters, making it very difficult to measure any loss. More importantly, some muck will remain even under the best decomposition scenario because not all the organic material will decompose. Thus, purchase of bacterial pellets or solutions for muck control is likely not the wisest possible investment.
Conclusion
Before deciding on any approach for muck removal, determine the magnitude of the muck problem and the amount of money available for the muck-removal project. Just dredging a "little bit," when dealing with a system-wide problem may make some individuals feel better, but will not solve the problem. System-wide dredging, however, is not always needed; instead, a site-specific project might be appropriate and enable the use of traditional road maintenance equipment. For the homeowner concerned with a small area, vacuuming the bottom and removing most of the sediments with a self-cleaning wedge-wire screen might be a workable solution. Alternatively, an outboard engine to blow the muck into deeper water may be the quickest and most inexpensive solution for the homeowner. Both approaches, however, will have to be repeated.
References
Allhands, M. N. 2005. Removing solids with automatic self-cleaning filters. The 15th Annual Produce Water Seminar. Houston TX.
Coale, F. J., F. T. Izuno, and A. B. Bottcher. 1994. Phosphorus in drainage from sugarcane in the everglades-agricultural areas as affected by drainage rate. Journal of Environmental Quality. 23:121–126.
Evett, J., and Cheng L. (2007), Soils and Foundations (7 ed.), Prentice Hall, pp. 9–29, ISBN 0132221381
Bachmann, R. W., M. V. Hoyer, S. B. Vinzon, and D. E. Canfield, Jr. 2005. The origin of the fluid mud layer in Lake Apopka, Florida. Limnology and Oceanography. 50: 629–635.
Havens, K. E., K. R. Jin, N. Iricanin, and R. T. James. 2007. Phosphorus dynamics at multiple time scales in the pelagic zone of a large shallow lake in Florida, USA. Hydrobiologia. 581:25–42.
Hoyer, M. V., R. W. Bachmann and D. E. Canfield Jr. 2008. Lake management (muck removal) and hurricane impacts to the trophic state of Lake Tohopekaliga, Florida. Lake and Reservoir Management 24: 57–68.
Hoyer, M. V., M. D. Netherland, and D. Jones. 2016. Littoral sediment accumulation 10-years after muck removal in Lake Tohopekaliga, FL, USA. Journal of Aquatic Plant Management. 54: 87–94.
Pennak R. W. 1953. Fresh-Water Invertebrates of the United States. The Ronald Press Company. New York.
Rey, J. R., D. B. Carlson, and R. E. Brockmeyer. 2012. Wetlands Ecological Management (2012) 20: 197. doi:10.1007/s11273-011-9235-1
Ruttner, F. 1953. Fundamentals of Limnology. University of Toronto Press. Canada.
Schnurrenberger, D. S., J. M. Russell, and K. R. Kelts. 2003. "Classification of lacustrine sediments based on sedimentary components." J. Paleolimnology 29: 141–154.
Soil Survey Staff. 1999. Soil Taxonomy: A Basic System of Soil Classification for Making and Interpreting Soil Surveys. USDA Natural Resources Conservation Service, Agric. Hdbk. 436, U.S. Government Printing Office, Washington, D.C. 869 pp.
Strahler, A.N. 1975. Physical Geography. 4th ed. John Wiley and Sons. New York. page 371.
Whitmore, T.J., M. Brenner, and C.L. Schelske. 1996. "Highly variable sediment distribution in shallow, wind-stressed lakes: a case for sediment mapping surveys in paleolimnological studies." Journal of Paleolimnology 15:207-221.