Induction and Establishment of Tetraploid Oyster Breeding Stocks for Triploid Oyster Production
Consult the glossary at the end of this publication for explanations of terms in bold and italics.
Abstract
Triploid-tetraploid breeding technology has been applied to oyster aquaculture worldwide. Triploid oysters are preferred by farmers because of their fast growth, better meat quality, and year-round harvest. Tetraploids are essential for triploid oyster aquaculture because commercial production of all-triploid seed requires sperm from tetraploids. This mini-review focuses on tetraploid oyster production and is a follow-up to a previous publication on triploid oyster production for aquaculture (Yang et al. 2018). This publication is intended to convey basic knowledge about tetraploid induction and breeding to shellfish farmers and the general public.
Introduction
The superior performance of triploid oysters has been widely recognized by the commercial oyster culture industry with many facilities created to support their production and commercial oyster farming (Guo 2004; Piferrer et al. 2009; Degremont et al. 2015; Yang et al. 2018). Currently, triploid oysters are commercially cultured primarily in two species: the Pacific oyster Crassostrea gigas, a species cultured worldwide, and the eastern oyster Crassostrea virginica, a species cultured along the East Coast of the United States and in the Gulf of Mexico. Triploid Pacific oysters now account for about 50% of the production along the US Northwest Coast and most of the hatchery seed production in France (Degremont et al. 2016). In 2018, over 2.3 billion triploid Pacific oyster seed were produced in China (Guo, personal observation). In Australia, triploid Pacific oysters account for about 15% of the production (Peachey and Allen 2016). In the United States, triploid eastern oysters account for nearly 100% of seed production in the Chesapeake Bay (Peachey and Allen 2016), and they are the primary seed for the Gulf of Mexico oyster aquaculture (Yang, personal observation). Overall, aquaculture of triploid oysters has become an important component of the oyster industry worldwide.
Commercial production of all-triploid oysters is achieved by crossing (mating) tetraploid (producing "diploid" gametes) males with normal diploid (producing "haploid" gametes) females (Guo et al. 1996). This is the preferred method because direct induction by chemical or physical treatments rarely produces 100% triploids in commercial hatcheries (Yang et al. 2018). Therefore, tetraploid breeding stocks are critical for the production and farming of triploid oysters.
Tetraploid induction in shellfish has been a challenge, and enormous efforts have been undertaken to produce tetraploids (Guo et al. 2009; Piferrer et al. 2009). The primary hurdle is the poor survival of induced tetraploids, a problem that makes the establishment of breeding stocks extremely difficult (see a comprehensive review, Guo et al. 2009). After testing several approaches of tetraploid induction and noting abnormal development, Guo (1991) hypothesized that the problem might be caused by the small size of eggs from diploids and that viable tetraploids could be produced using larger eggs from triploids. Guo and Allen (1994) tested the hypothesis and succeeded in producing viable adult tetraploids using the few eggs produced from triploid females fertilized with normal haploid sperm from diploids, which was then followed by inhibition of the first polar body (PB1). Tetraploid breeding stocks were established using the Guo and Allen method in the Pacific oyster for the first time in 1993. Later, this method was applied to other shellfish species, leading to the production of viable tetraploids in the pearl oyster (He et al. 2000), eastern oyster Crassostrea virginica (Guo et al. 2002), Pacific calico scallop Argopecten ventricosus (Maldonado et al. 2003), Suminoe oyster Crassostrea ariakensis (Allen et al. 2005), and bay scallop Argopecten irradians (Surier et al. 2012). This novel method was patented by Rutgers University (US5824841A) and commercialized worldwide. After 20 years, the Rutgers patent expired in January 2015, and this method for tetraploid production is available for free commercial use (Guo and Allen 1994a).
Approaches for Tetraploid Oyster Production
Many molluscan bivalves, including oysters, clams, mussels, and scallops, release their eggs before completing meiosis (the released eggs are called primary oocytes) (Galtsoff 1964; Gosling 2003). Upon insemination, the eggs resume the maturation process (i.e., meiosis, including meiosis I and II, Figure 1), release polar body I and polar body II, and then start mitosis and embryonic development after uniting with paternal chromosomes from the sperm. The release of eggs before meiosis provides an opportunity to manipulate the meiotic process to change chromosome number and produce polyploid bivalves.
Based on the characteristics of chromosome segregation, tetraploid production can be achieved through different methods. Methods are either direct or indirect, as described below. Since 1991, studies have been conducted on tetraploid induction in 15 shellfish species. Methods used and success of tetraploid induction indicated at both the larval stage and juvenile stage for various methods are listed in Table 1 (updated from Guo et al. 2009).
Similar to methods used for triploid production (Guo et al. 2009; Piferrer et al. 2009; Yang et al. 2018), methods for inhibition of meiosis or mitosis for tetraploid production include heat shock, cold shock, cytochalasin B (CB), 6-dimethylaminopurine (6-DMAP), caffeine, and colchicine. The effectiveness of these methods varies depending on the species and determining which method will be most effective usually requires trials and optimization (Table 1).
Direct Methods: (2N? × 2N? Plus Inhibition of Meiosis or Mitosis)
Direct methods are manipulations applied directly to the fertilized eggs of normal diploids (2N? × 2N?, i.e., diploid female × diploid male). Direct methods have been extensively tested and have resulted in 0 to 94.6% tetraploid production at the embryo and larval stages (Table 1). The survival to the juvenile stage of tetraploid larvae produced by direct methods (beyond metamorphosis) was low, regardless of the method used. The methods examined include:
Inhibition of First Cell Division (Mitosis I)
Tetraploid induction by mitosis inhibition has been attempted with the Pacific oyster (Guo et al. 1994), dwarf surfclam Mulinia lateralis (Yang and Guo 2006a), and hard clam Mercenaria mercenaria (Yang and Guo 2006b). High percentages of tetraploids (up to 86%) were found at the larval stage when using heat shock treatment in Mercenaria mercenaria (Table 1); however, none of the tetraploid larvae survived to the juvenile stage.
Inhibition of Polar Body I Formation (Meiosis I)
Inhibiting meiosis I (i.e., polar body I) with chemical or physical treatments has produced viable tetraploids beyond metamorphosis in several species, including the Mediterranean mussel Mytilus galloprovincialis (Scarpa et al. 1993), Manila clam Tapes philippinarum (Allen et al. 1994), zhikong scallop Chlamys farreri (Yang et al. 2000), dwarf clam Mulinia lateralis (Peruzzi and Guo 2002; Yang and Guo 2004), blue mussel Mytilus edulis (McCombie et al. 2009), two tropical oysters Crassostrea belcheri and Crassostrea iredalei (Tan et al. 2017), and Pacific oysters (Benabdelmouna and Ledu 2015). In general, the number of tetraploid individuals surviving to juvenile stage was low in almost all the studies (Table 1). Poor survival makes it difficult to establish tetraploid breeding stocks.
Recently, it was reported that tetraploids induced by this direct approach in the Pacific oyster (Benabdelmouna and Ledu 2015) had high survival to the adult stage (no exact number reported), and a patent was issued for this method in France (FR patent #2913982-A1).
Other Methods
Other direct methods to create tetraploid Pacific oysters include inhibition of meiosis I and II, combination of meiosis inhibition with or without inactivated sperm, and cell fusion (Guo 1991; Guo et al. 1994). Tetraploid larvae were produced at high percentages (up to 94.6%) but did not survive beyond metamorphosis.
In general, these direct methods were effective at inducing tetraploidy, but the tetraploid larvae had poor survival beyond metamorphosis. The few viable tetraploids that did reach juvenile or adult stage were insufficient to establish tetraploid breeding lines for triploid seed production.
Indirect Method—Fertilized Eggs (3N? × 2N? Plus Treatments)
One hypothesis for the poor survival of tetraploid larvae using direct methods on diploid eggs posits that the tetraploids have a deficiency in the amount of cytoplasm arising from the cleavage of a normal egg with a large tetraploid nucleus, resulting in an abnormal nucleus/cytoplasm ratio (Guo 1991; Guo and Allen 1994a).
Theoretically, triploid oysters are sterile because the three sets of chromosomes do not segregate correctly during meiosis, resulting in no gamete production (i.e., sterility). However, some triploid females do produce fewer but larger eggs than diploids (Yang, unpublished data). It has been hypothesized that viable tetraploids might be produced using eggs from triploids fertilized with sperm from diploids along with polar body inhibition (Guo 1991, Figure 1). Tetraploids produced by this method did survive beyond metamorphosis (Guo and Allen 1994). After the first report, this novel method has been modified for efficiency and greater tetraploid occurrence (Eudeline et al. 2000), as well as being applied to other shellfish species (Table 1), including pearl oyster (He et al. 2000), Pacific calico scallop (Maldonado et al. 2003), eastern oyster (Guo et al. 2002; Peachey and Allen 2016), Suminoe oyster (Allen et al. 2005), and bay scallop (Surier et al. 2012). To date, tetraploid breeding populations have been established in Pacific, eastern, and Suminoe oysters. Triploid seed are now commercially produced for both Pacific and eastern oysters.
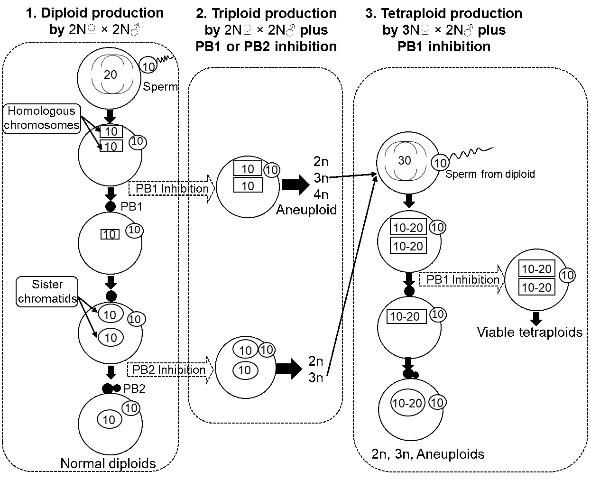
Challenges for Tetraploid Production
It is evident that challenges still exist for tetraploid production. These challenges include:
The Infrequent Occurrence of Fecund Triploid Females
Triploids exhibit abnormal gonad development and the occurrence of fecund triploid females is very rare. Supan (2000) reported that about 1 female out of 1,600 triploid eastern oysters (i.e., 0.06%) had eggs available for tetraploid induction. In contrast, higher proportions of fecund females are observed in triploid Pacific oysters (58%, Guo and Allen 1994c) and triploid dwarf clam Mulinia lateralis (33%, Guo and Allen 1994b).
The Limited Availability of Oocytes from Triploid Females
Oocytes from triploid organisms usually have abnormal meiosis due to the three sets of chromosomes. Thus, the number of oocytes from triploid females is usually low (Gong et al. 2004) and has varied from 100 to 1.6 million per female in the eastern oyster (Yang, unpublished data). Efforts have been made to improve gonad development in triploids, such as treating the eastern oyster with estradiol (Quintana 2005). The availability of oocytes may relate to the age and culture location of triploid oysters.
The Poor Survival of Induced Tetraploid Larvae
The poor survival of induced tetraploid larvae is a major challenge for tetraploid production. In the Pacific oyster, the reported survival from fertilized eggs to putative tetraploid spat was 0% in two replicates and 0.0739% in one replicate (Guo and Allen 1994a). Scarpa et al. (1993) found that tetraploid mussels were smaller than diploid and triploid mussels in the same cohort, which suggests that tetraploid larvae are smaller (Benabdelmouna and Ledu 2015). Smaller larvae (i.e., slower growing) are typically disposed of during larval culture. Extensive care is needed for culture of putative tetraploid larvae by maintaining good culture conditions and saving even slow growers to ensure they survive beyond metamorphosis (juvenile stage) and then to the adult stage.
Performance of Tetraploids
The performance of tetraploids is varied. One early study of the Pacific oyster showed that first-generation tetraploids were larger than diploids and triploids (Guo and Allen 1994a), but in a later study, tetraploids were much smaller than diploids and triploids even at the larval stage (Benabdelmouna and Ledu 2015). Usually, after several generations, tetraploids tend to be stabilized and well-adapted to the environment (Benabdelmouna and Ledu 2015). For the eastern oyster, first-generation tetraploids were smaller than diploids or triploids (Guo et al. 2002). In early spring, when gonadal development normally occurs, tetraploids have higher mortality, which is common for the first few generations; after four generations, the performance of tetraploids is significantly improved (Guo 2012).
Tetraploid oysters are fertile and possess normal gonad development that results in viable "diploid" gametes for 100% triploid production. For the Pacific oyster, fecundity of tetraploids is comparable to that of normal diploids (Guo and Allen 1997). For eastern oysters, tetraploids can develop normal gonads and spawn naturally (personal communication, Dr. J. Supan, Louisiana State University). Furthermore, tetraploids have an approximately 1:1 sex-ratio at one year of age and exhibit the same low level of hermaphrodites as diploids (Guo and Allen 1997). Tetraploid male gonads go through normal meiosis and produce primarily diploid sperm with some aneuploids or mosaics (Guo and Allen 1997; Yang, personal observation). However, sperm motility in tetraploids varies depending on populations and individuals; about one-third of males have sperm showing progressive motility (i.e., proportion of sperm swimming slowly or quickly) comparable to the sperm from diploids (Yang, unpublished data). Triploids produced from tetraploids exhibited better growth than directly induced triploids as detailed in reviews by Guo et al. (2009) and Yang et al. (2018).
Establishment of Tetraploid Breeding Stocks
Establishment of tetraploid breeding stocks requires a sufficient number of tetraploids with broad genetic diversity. Once tetraploid founders are produced through the methods stated above, other methods can be applied to increase the number of tetraploid individuals and enrich the genetic diversity of induced tetraploids (Figure 2). Besides continuation of the method stated above by using 3N? (triploid) × 2N? (diploid) plus inhibition of PB1, the following methods offer alternatives for producing tetraploids.
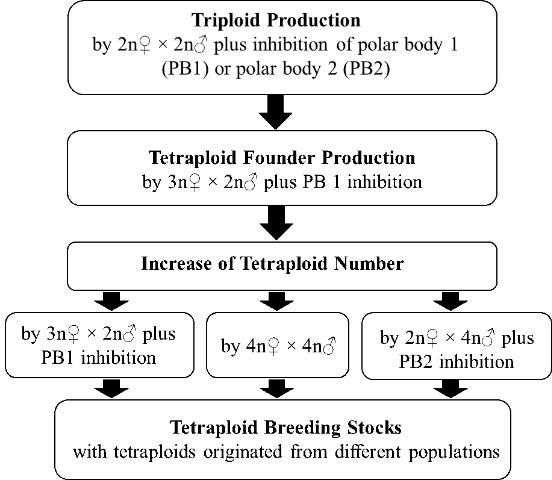
Tetraploids Crossing with Tetraploids (4N? × 4N?)
Tetraploids have normal gonad development and fecundity, therefore, tetraploid founders can be crossed with each other to produce more tetraploids. Special care is needed to maintain tetraploid founders, especially their pedigree information, to prevent crossing of brothers and sisters (i.e., inbreeding). This will ensure the genetic diversity of tetraploid populations.
Diploids Crossing with Tetraploids (2N? × 4N? Plus Inhibition of PB2)
This method is a complementary approach to enrich the tetraploid genetic background. Tetraploids have been produced in the Pacific oyster by fertilizing oocytes from normal diploids with sperm from tetraploids, followed by inhibition of polar body 2 (PB2) (McCombie et al. 2005). This is an approach to diversify, change, and broaden the genetic background of tetraploid populations; 50% of the genetic material in the tetraploids produced by this approach comes from the diploids. However, producing tetraploids using this method is challenging because of the low survival of the resultant tetraploids (Guo, personal experience).
Summary and Perspectives
Triploid oysters are being used for commercial production in many countries, including the United States, Australia, France, Chile, China, and Korea. Tetraploids have played a major role in the commercial production of triploid oysters. In the United States, triploid-tetraploid technology has been applied to the Pacific oyster on the West Coast and to the eastern oyster on the East Coast, especially the Chesapeake Bay. Additionally, oyster aquaculture in the Gulf region is rapidly growing, and triploids are the preferred product. The application of triploid-tetraploid technology is expected to continue contributing to oyster aquaculture worldwide. Triploid-tetraploid technology may also have great potential in aquaculture of other shellfish species, such as scallops, mussels, and clams.
Acknowledgements
This study was supported by funds from the Gulf States Marine Fisheries Commission (ACQ-210-039-2017 UFL and ACQ-210-039-2018-UFL), the National Sea Grant (special workshop project NA17OAR4170227 and Aquaculture Initiative Award NA17OAR4170227), and a USDA NIFA Aquaculture Award (2015-70007-24245).
Glossary
Aneuploids: Aneuploids are organisms whose cells contain an abnormal number of chromosomes that are not in complete sets.
Cell fusion: A process in which cells with a single nucleus combine into one cell with a new, larger nucleus or multiple nuclei.
Chromosome: A threadlike structure of deoxyribonucleic acid (DNA) molecules intermingled with ribonucleic acid (RNA) and proteins in the nucleus of most living cells, which carries genetic information in the form of genes.
Diploid: Naturally, most eukaryotic species, including oysters, have two sets of chromosomes, one set inherited from the organism's father and another set from its mother. These organisms are called diploids (2N).
Gamete: Reproductive cell with a haploid number of chromosomes, such as an egg, sperm, or spore. In sexual reproduction, gametes from males and females form diploid zygotes through fertilization.
Haploid: An organism with a single set of unpaired chromosomes.
Hermaphrodite: An organism that has complete or partial reproductive organs and produces gametes normally associated with both male and female sexes.
Insemination: The contact of sperm to an egg membrane. Sometimes the term fertilization is used, which technically is when the male and female chromosomes align at the first mitotic metaphase.
Meiosis: The cell division process that produces gamete cells (eggs and sperm) or spores and which involves one replication of chromosomes and two nuclear divisions to produce four haploid cells (gametes or plant spores).
Mitosis: The cell division process that results in two daughter cells with the same number and kind of chromosomes as the parent nucleus for tissue growth.
Mosaics (or mosaicism): the presence of two different genotypes, such as different ploidies, in one individual that developed from a single fertilized egg.
Oocyte: A pre-meiotic cell in the ovary (i.e., female gonad). Once meiosis is complete, the oocyte is called an ovum. Typically, the term "egg" is used generally for both of these stages.
Polar body: The cells produced at either the first cell division (polar body I) or the second cell division (polar body II) during the meiotic process in oocytes. Polar bodies usually contain almost no cytoplasm due to unequal cell division.
Polyploidy: Organisms with more than two sets of chromosomes.
Triploid (3N): Organisms with three sets of haploid chromosomes. Accordingly, organisms or gametes with one set of chromosomes are called haploid (N).
Tetraploid (4N): Organisms with four haploid sets of chromosomes.
References
Allen, S. K., A. J. Erskine, E. J. Walker, and G. A. DeBrosse. 2005. "Production of tetraploid suminoe oysters, Crassostrea ariakensis." Aquaculture 247: 3 (Abstract only).
Allen, S. K., M. Shpigel, S. Utting, and B. Spencer. 1994. "Incidental production of tetraploid Manila clams, Tapes philippinarum (Adams and Reeve)." Aquaculture 128: 13–9.
Benabdelmouna, A., and C. Ledu. 2015. "Autotetraploid Pacific oysters Crassostrea gigas obtained using normal diploid eggs: induction and impact on cytogenetic stability." Genome 58: 333–48.
Degremont, L., C. Garcia, and S. K. Allen. 2015. "Genetic improvement for disease resistance in oysters: A review." Journal of Invertebrate Pathology 131: 226–41.
Degremont, L., C. Ledu, E. Maurouard, M. Nourry, and A. Benabdelmouna. 2016. "Effect of ploidy on the mortality of Crassostrea gigas spat caused by OsHV-1 in France using unselected and selected OsHV-1 resistant oysters." Aquaculture Research 47: 777–86.
Eudeline, B., S. K. Allen, and X. Guo. 2000. "Optimization of tetraploid induction in Pacific oysters, Crassostrea gigas, using first polar body as a natural indicator." Aquaculture 187: 73–84.
Galtsoff, P. S. 1964. "The American oyster, Crassostrea virginica Gmelin, Fishery Bulletin Volume 64." United States Government Printing Office, Washington, D. C.
Gendreau, S., and H. Grizel. 1990. "Induced triploidy and tetraploidy in the European flat oyster, Ostrea edulis L." Aquaculture 90: 229–38.
Gong, N., H. Yang, G. Zhang, B. J. Landau, and X. Guo. 2004. "Chromosome inheritance in triploid Pacific oyster Crassostrea gigas thunberg." Heredity 93: 408–15.
Gosling, E. 2003. "Bivalve Mulluscs Biology, Ecology and Culture." Fishing News Books, Malden, MA.
Guo, X. 1991. "Studies on Tetraploid Induction in the Pacific Oyster, Crassostrea gigas (Thunberg). " University of Washington, Seattle, WA.
Guo, X. 2004. "Oyster breeding and the use of biotechnology." Bulletin of the Aquaculture Association of Canada 104: 26-33.
Guo, X. 2012. "Production and breeding of tetraploid eastern oyster Crassostrea virginica." Journal of Shellfish Research 31: 292.
Guo, X., and S. K. Allen. 1994a. "Viable tetraploids in the Pacific oyster Crassostrea gigas Thunberg produced by inhibiting polar body I in eggs from triploids." Molecular Marine Biology and Biotechnology 3: 42–50.
Guo, X., and S. K. Allen. 1994b. "Sex determination and polyploid gigantism in the Dwarf surfclam Mulinia lateralis Say." Genetics 138: 1199–206.
Guo, X., and S. K. Allen. 1994c. "Reproductive potential and genetics of triploid Pacific oysters, Crassostrea gigas (Thunberg)." Biological Bulletin 187: 309–18.
Guo, X., and S. K. Allen. 1997. "Sex and meiosis in autotetraploid Pacific oyster, Crassostrea gigas (Thunberg)." Genome 40: 397–405.
Guo, X., G. A. DeBrosse, and S. K. Allen. 1996. "All-triploid Pacific oysters Crassostrea gigas (Thunberg) produced by mating tetraploids and diploids." Aquaculture 142: 149–61.
Guo, X., W. K. Hershberger, K. Cooper, and K. K. Chew. 1994. "Tetraploid induction with mitosis-I inhibition and cell fusion in the Pacific oyster Crassostrea gigas Thunberg." Journal of Shellfish Research 13: 193–8.
Guo, X., J. Wang, B. J. Landau, L. Li, G. A. DeBrosse, and K. D. Krista. 2002. "The successful production of tetraploid eastern oyster, Crassostrea virginica Gmelin." Journal of Shellfish Research 21: 380–1 (Abstract only).
Guo, X., Y. Wang, Z. Xu, and H. Yang (2009) Chromosome Set Manipulation in Shellfish. In: New Technologies in Aquaculture: Improving Production Efficiency, Quality and Environmental Management (eds. by Burnell G & Allen G), pp. 165–94. Woodhead Publishing Limited, Abington, Cambridge.
He, M., Y. G. Lin, Q. Shen, J. X. Hu, and W. G. Jiang. 2000. "Production of tetraploid pearl oyster Pinctada martensii Dunker by inhibiting the first polar body in eggs from triploids." Journal of Shellfish Research 19: 147–51.
Maldonado, R., A. M. Ibarra, and J. L. Ramirez. 2003. "Induction to tetraploidy in catarina scallop, Argopecten ventricosus (Sowerby II, 1842)." Ciencias Marinas 29: 229–38.
McCombie, H., F. Cornette, and A. R. Beaumont. 2009. "Short sharp shock produces viable tetraploids in crosses of diploid blue mussels Mytilus edulis." Aquaculture Research 40: 1680–2.
McCombie, H., C. Ledu, P. Phelipot, S. Lapegue, P. Boudry, and A. Gerard. 2005. "A complementary method for production of tetraploid Crassostrea gigas using crosses between diploids and tetraploids with cytochalasin B treatments." Marine Biotechnology 7: 318–30.
Peachey, B. L., and S. K. Allen. 2016. "Evaluation of cytochalasin B and 6-dimethylaminopurine for tetraploidy induction in the eastern oyster, Crassostrea virginica." Aquaculture 450: 199–205.
Peruzzi, S., and X. Guo. 2002. "Tetraploid induction by meiosis inhibition with cytochalasin B in the dwarf surfclam, Mulinia lateralis Say: Effects of temperature." Journal of Shellfish Research 21: 677–84.
Piferrer, F., A. Beaumont, J. C. Falguière, M. Flajšhans, P. Haffray, and L. Colombo. 2009. "Polyploid fish and shellfish: Production, biology and applications to aquaculture for performance improvement and genetic containment." Aquaculture 293: 125–56.
Quintana, R. 2005. "Effect of estradiol-17B on the gonadal development of diploid and triploid female eastern oysters. " p. 139. Louisiana State University, Baton Rouge, LA.
Scarpa, J., K. T. Wada, and A. Komaru. 1993. "Induction of tetraploidy in mussels by suppression of polar body formation." Nippon Suisan Gakkaishi 59: 2017–23.
Supan, J. E. 2000. "Tetraploid eastern oysters: an arduous effort." Journal of Shellfish Research 19: 655 (Abstract Only).
Surier, A., R. Karney, X. Guo, and Y. Wang. 2012. "Challenges to creating a tetraploid broodstock for the Bay scallop Argopecten irradians." Journal of Shellfish Research 31: 352.
Tan, S. H. A., C. P. Teh, G. O. Chang, and Z. Yasin. 2017. "Tetraploid induction in tropical oysters, Crassostrea belcheri (Sowerby) and Crassostrea iredalei (Faustino)." Aquaculture Research 48: 1406–12.
Yang, H., and X. Guo. 2004. "Tetraploid induction by meiosis inhibition in the dwarf surfclam Mulinia lateralis (Say 1822): Effects of cytochalasin B duration." Aquaculture Research 35: 1187–94.
Yang, H., and X. Guo. 2006a. "Polyploid induction by heat shock-induced meiosis and mitosis inhibition in the dwarf surfclam, Mulinia lateralis Say." Aquaculture 252: 171–82.
Yang, H., and X. Guo. 2006b. "Tetraploid induction by inhibiting mitosis I with heat shock, cold shock, and nocodazole in the hard clam Mercenaria mercenaria (Linnaeus, 1758)." Marine Biotechnology 8: 501–10.
Yang, H., N. Simon, and L. Sturmer. 2018. FA208. Production and performance of triploid oysters for aquaculture.Gainesville: University of Florida Institute of Food and Agricultural Sciences. https://edis.ifas.ufl.edu/fa208. p. 9.
Yang, H., F. Zhang, and X. Guo. 2000. "Triploid and tetraploid Zhikong scallop, Chlamys farreri Jones et Preston, produced by inhibiting polar body I." Marine Biotechnology 2: 466–75.
Zhang, G., Z. Wang, Y. Chang, J. Song, J. Ding, S. Zhao, and X. Guo. 2000. "Tetraploid induction in the Pacific abalone Haliotis discus hannai Ino with 6-DMAP and CB." Journal of Shellfish Research 19: 540–1.