Terms in bolded text appear in a glossary preceding the references section in this publication.
Introduction
Florida’s abundance of the eastern oyster, C. virginica, hereafter referred to as “oysters,” in regions of its historical wild fisheries has declined in the past decade due to a multitude of factors including intense fishing, algal blooms, named storm events, and drought (Walters et al. 2021). One area of Florida that has exhibited a pronounced oyster decline is Apalachicola Bay. This area was once Florida’s leading wild oyster-producing estuary until a fishery crash in 2012 (Figure 1). Subsequent population declines followed, dropping landings (harvests) by 98% from 460,000 bushels per year to just 10,000 bushels in 2018 (Botta et al. 2020).
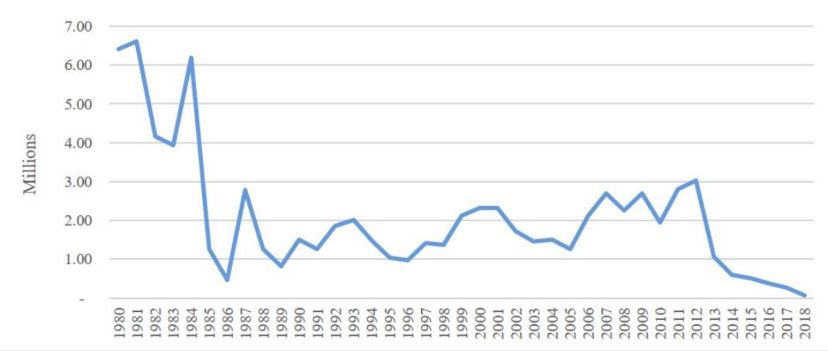
Credit: Botta et al. (2020)
One major cause of the Apalachicola Bay oyster fishery crash was the actions taken after the 2010 Deepwater Horizon oil spill. Farmers in Apalachicola Bay were encouraged to harvest their oysters before the oil reached the bay. Although the oil did not impact Apalachicola Bay, the concern was that it would result in an almost complete removal of harvestable oysters. This removal of biomass, combined with high salinity conditions due to low river flows from drought events and upstream freshwater demands, created inadequate environmental conditions for juvenile growth and recruitment (FLSGP 2013). (See Petes et al. 2012 for Apalachicola-Chattahoochee-Flint (ACF) Basin water wars.) The Florida Fish and Wildlife Conservation Commission (FWC) suspended this wild oyster fishery from December 2020 through December 31, 2025 (FFWCC 2013; Pine III et al. 2015; Brown et al. 2021; FDACS 2021). The lack of harvestable oysters from this area increased demands on other harvestable areas in Florida, contributing to overfishing throughout the state. Growth of oysters could not sustain demand.
Without a reliable wild oyster fishery, Florida has turned to commercial aquaculture to provide oysters to market through the expansion of aquaculture capacity and oyster habitat restoration programs. In 2019, 719 commercial shellfish leases were issued among 336 producers. Of the 336, 56% produced only clams, 16% produced only oysters, and 28% produced both (FDACS 2020a). Currently, no-gear bottom culture and harvesting via hand tonging remains Florida’s conventional form of oyster production, but off-bottom culture has been explored in more recent years starting in 2015 when regulations allowed for the use of the water column for oyster farming. Methods of oyster farming remain rudimentary and low-cost with high labor inputs. To advance the oyster industry, the introduction of new culture technologies can provide an opportunity for increased production (Walton and Swann 2021).
This publication will identify current practices used in oyster aquaculture in Florida and innovative technological approaches that can improve oyster production. It is relevant to commercial aquaculture for the consumer market and restoration aquaculture to improve wild fisheries and ecosystem quality. A glossary of the terms that appear in bolded text throughout this publication appears before the references section in this publication.
Traditional Aquaculture
Aquaculture of oysters follows a 3-step process: hatchery production of seed, nursery rearing of juveniles to appropriate planting size (25 mm or 1 in), and growout to market size on shellfish leases. In some regions, oysters may be grown to adult sizes via oyster gardening programs by local communities for restoration purposes only (FDACS 2021; FOS 2021).
Hatcheries provide spat to shellfish farmers as spat-on-shell or single-seed that were produced in a controlled environment designed to mimic natural environmental cues (e.g. water temperature, salinity) to facilitate reproduction and growth. Spat-on-shell oysters are achieved by settling larvae onto cultch, typically a whole adult oyster shell. Several spat can grow on a single shell, eventually resulting in clusters of oysters (Figure 2). Single-seed oysters (Figure 2) are accomplished by settling larvae onto a micro cultch, usually ground oyster shell 250 to 300 µm in diameter, upon which a single larva settles (Wallace et al. 2008). Once the oyster grows, it appears to be a “single” oyster as opposed to the cluster of oysters seen in spat-on-shell.
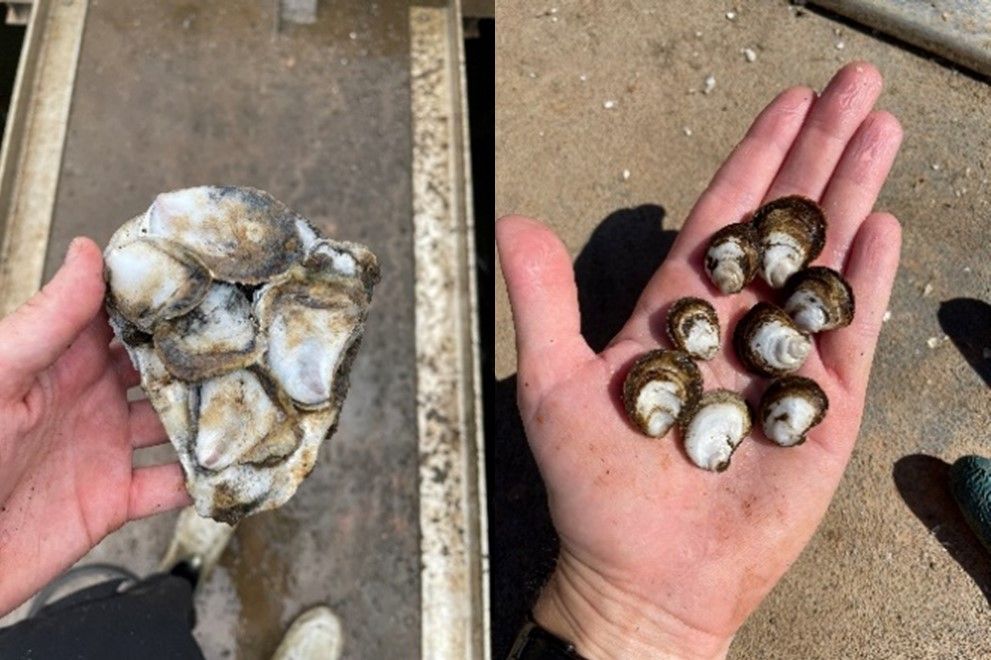
Credit: Emilly Caffrey
The hatchery process involves conditioning adult broodstock oysters to release gametes. Gametes from both male and female oysters are spawned and fertilization occurs in the water column. Free-swimming larvae subsequently develop through three larval stages (Figure 2) over the next 2–3 weeks, beginning with the non-feeding trochophore stage. After 1–2 days, trochophores transition into the feeding veliger stage with development of cilia, making them more mobile. Thin-shelled veligers then grow a foot and eye spots, bringing the larvae to its final pediveliger stage. The pediveliger uses its foot to search for cultch, or adequate substrate to settle upon. After detecting its desired hard substrate, the pediveliger will settle and attach itself by secreting a cement-like substance, signifying metamorphosis into spat, the juvenile life stage (Wallace 2001; Wallace et al. 2008).
After seed production, oysters are grown in nurseries until they are large enough to outplant at 1 inch (25mm). After outplanting, an oyster is raised to its harvest size (market size) of 3 inches (76 mm). A spat can grow to its harvest size in 12 to 36 months depending on temperature, salinity, dissolved oxygen, and culture technique. Optimal water quality parameters include a temperature range of 20–28 degrees Celsius, 10–30 ppt salinity, and at least 3 ppm dissolved oxygen. There must be adequate water flow for oysters to gather phytoplankton as food sources (Wallace 2001; ORP 2021).
No-Gear Bottom Culture
No-gear bottom culture places later spat and adult spat-on-shell oysters onto a submerged, suitable bottom environment for growout. This is the least intensive, lowest-cost option to culture oysters. A base of substrate underneath the planted spat-on-shell must be used to elevate the growing oysters to avoid direct contact with the bottom and minimize siltation, where oysters may be smothered by mud and sand (CRC 2008). A layer of already shucked oyster shells without spat, also called “green shell,” can function as the base level (Webster and Meritt 2004).
In addition to the risks posed by siltation and physical damage, open exposure to the environment makes bottom cultured oysters vulnerable to predation by crabs, fish, and snails who readily feed on small benthic organisms (Love et al. 2021). Anoxic conditions (<2 ppm) may also occur more frequently on the seafloor in the summer months, subjecting oysters to periods of anoxia.
For Restoration
No-gear bottom culture is the final placement for reef restoration projects where oysters are outplanted after growing in gardening programs for about 9 months. The gardening process uses wild diploid oysters, rather than hatchery-reared seed, by collecting their spat from local reefs. Wild spat are first collected via strategically placed “oyster T’s,” a PVC pipe constructed in a T shape. Blank oyster shells are hung from the oyster T’s to collect naturally settling spat recruits.
After spat is collected, the spat-on-shell is placed in cages and hung from docks maintained by local volunteers. This off-bottom “gardening” helps prevent predation and disease on young oysters, giving them a higher chance of maturation into adulthood. Once they grow to less vulnerable sizes, adult oysters are returned to their respective wild reefs by placing them on top of the existing reef. This process is executed in areas where oyster habitat is sparse; expansion of the wild reefs increases reef density, allowing for increased survival of recruits and improved spawning numbers. Reef recovery eventually enhances water clarity, shoreline stabilization, and biodiversity as the reefs act as a sanctuary for other aquatic organisms (FOS 2021).
Bottom Cage Culture
Bottom cage culture involves placing young oysters in protective cages before bottom planting. Juvenile oysters as small as 1 inch (25 mm) may be placed in culture cages equipped with mesh to avoid predation, increasing their chance of survival. The cages still allow for adequate water flow for feeding. The cages are positioned directly onto or just above bottom habitat. A base layer of material is not needed for this technique (CRC 2008).
Off-Bottom (Suspended) Culture
Similar to bottom cage culture, suspended culture places oysters in protective cages or bags; however, they are hung in the water column or at the surface by floats or rack systems. Raising oysters in the water column improves the flow of water around them, which helps them grow faster because it increases their access to food particles in the water. Other advantages include protection from predators, improved shell shape, control of density stocking and biofouling, and increased product consistency (Walton and Swann 2021).
Closer proximity to the surface increases sunlight exposure, providing better conditions for biofouling organisms (e.g. barnacles, sponges, tunicates, macroalgae) to attach and grow on the cages and gear. Regular husbandry and cleaning helps mitigate biofouling. In cases where the cages are at the surface, periodically flipping gear is a best practice for biofouling mitigation that causes the biofouling organisms to desiccate, or dry out, whereupon they die and slough off. While there is ease of access associated with floating and hanging bags and cages, the flipping and cleaning process can be quite laborious.
Off-bottom culture is relatively new to oyster farming in Florida. A 2019 UF/IFAS study compared the survival and quality of triploid oysters grown in Cedar Key, Florida, using different commercially available floating cages and bag systems including OysterGro® cages and GoDeep bags. OysterGro® cages have a top and bottom compartment, while GoDeep bags have a single compartment. The results of the study showed similar survival rates across all gear types. Shell height and length were marginally greater for oysters grown in top compartment cages. OysterGro® cages also exhibited the most biofouling. Floating bags produced oysters with higher wet and dry meat weight and improved condition index (UF/IFAS 2019).
Innovations in Technology
The innovations described below aim to minimize the manual labor associated with oyster aquaculture, while building off of traditional culture techniques. These techniques use technology to automate the biofouling management and tumbling processes.
Solar Oyster Production System (SOPS)
Solar Oysters LLC, located in Baltimore, Maryland, engineered a prototype vessel, the Solar Oyster Production System (SOPS), with the aim of maximizing oyster output and minimizing manual labor. This model keeps the advantages of traditional, intensive suspended culture and adds automated technology to further enhance growth rate and quality of oysters within a small, vertical footprint. The 40 ft x 25 ft platform is outfitted with 12 375-watt roof-mounted solar panels and 5 rotatable ladders. Each ladder contains 23 rows with the capacity to hold 5 SEAPA cages per row (Figure 3). Each 25 L cage contains up to 120 spat-on-shell oysters (20 mm mesh cage used) or 1000 small single-seed oysters (6 mm and 12 mm mesh cages used depending on seed size) for growout. Seed oysters may be transferred to larger mesh sizes with growth, while spat-on-shell oysters may be split into additional 20 mm cages with growth to prevent overweighting of the cage and overcrowding of oysters. The system uses solar energy to rotate shellfish cages to a working level and throughout the water column to water depth of about 16 ft. As of 2023, SOPS has completed two growing seasons during which oysters were continuously monitored for compatibility with the automated system.
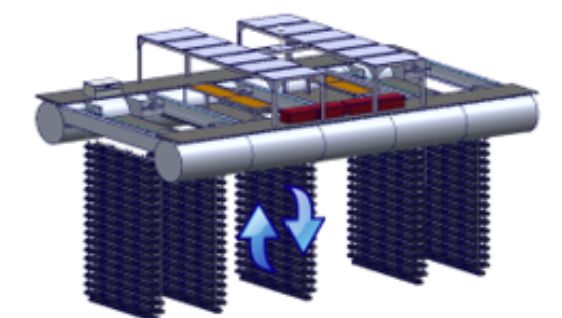
Credit: Solar Oysters, LLC, used with permission.
GRAINOCEAN ROLL’BAG®
Based in France where tidal depth fluctuations can range to 40 ft, GRAINOCEAN created an innovative cage, the patented ROLL’BAG® (Figure 4). The 3-inch-thick flat cage hangs from a stand. It is equipped with a lateral float to raise or lower itself with the tidal cycle; at high tide, the float keeps the cage hanging vertically upside down for uniform oyster exposure to nutrients. During changing tidal cycles, oysters roll down the cage surface, which mimics tumbling.
Depending upon the goal of the farmer, the ROLL’BAG® may be set to allow for free or limited movement of the cage. Free movement allows the cage to travel 360 degrees around the stand. As mentioned, this tumbles the oysters, which hardens their shells and improves their shape. Limited movement engages a restrictor link, keeping the oysters in position for shell growth (Fish Farmer Magazine 2022).
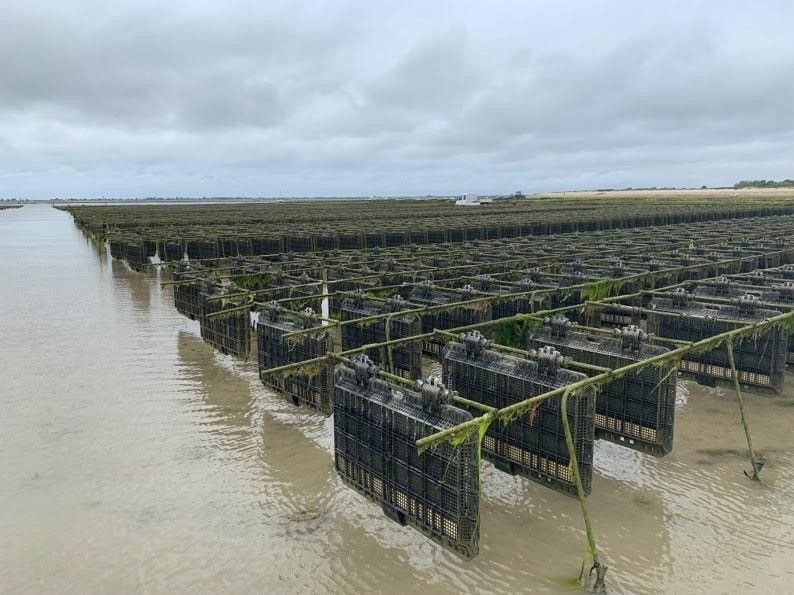
Credit: Grainocean International, used with permission.
FlipFarm Oyster System
FlipFarm Systems Ltd, based in New Zealand, attaches oyster cages in series along polypropylene rope. This long string of cages is equipped with floats to keep oysters near the surface. To reduce biofouling, cages are flipped periodically via a “flipper.” A vessel is equipped with a looped track, and when the cage line is shuttled through the track loop while the boat runs parallel to the line, the cages are flipped onto their opposite sides.
A slightly different track is used to load and offload oysters within the cages. This track holds cages upright at waist level and allows farmers to open the cage door on its side (Figure 5). Farmers can either fill cages with oysters or offload oysters onto a sorting conveyor belt without having to completely remove cages from their floating line. The FlipFarm technology now has distribution and successful operation at oyster farms in Maine (FlipFarm 2022).
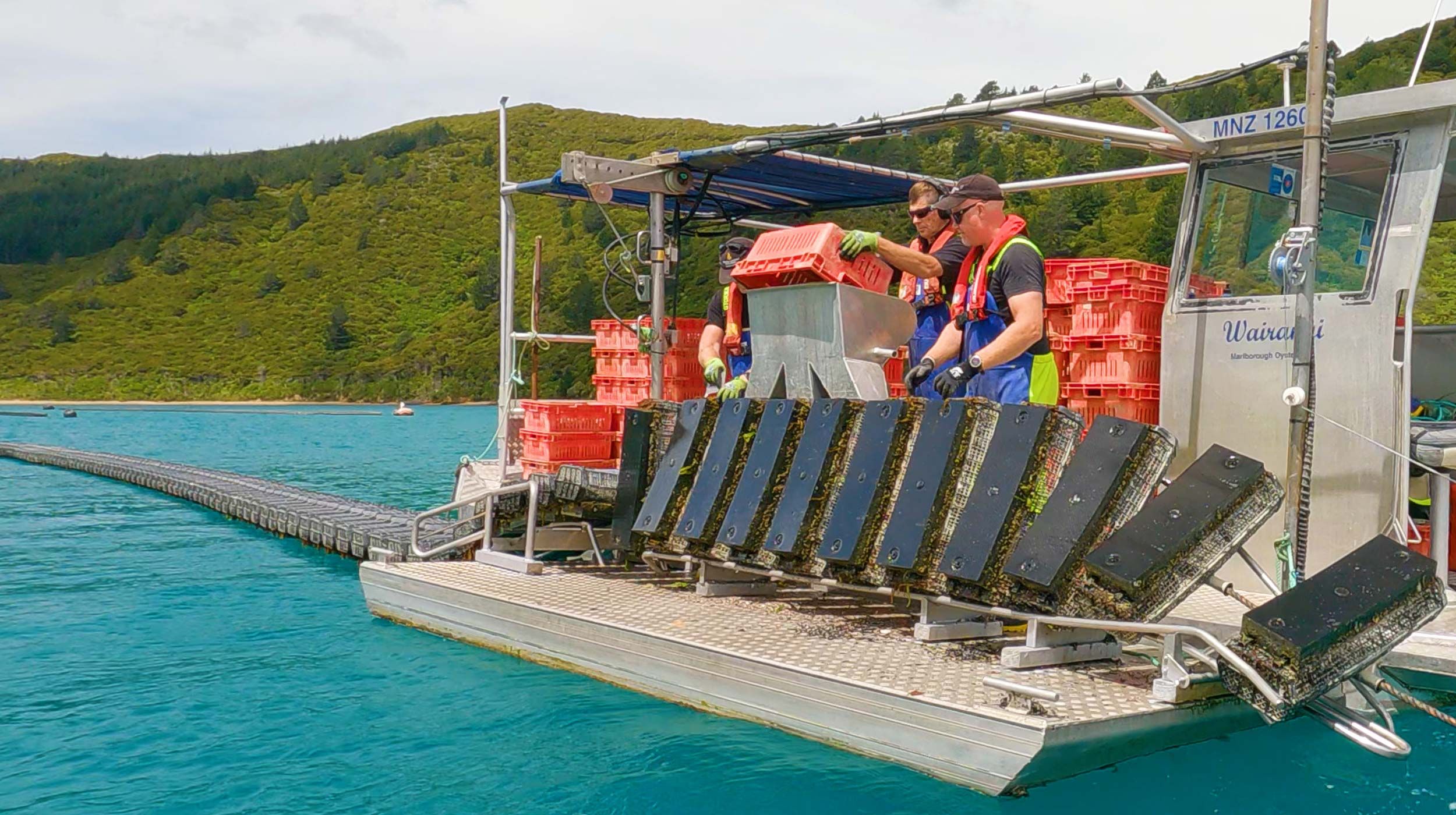
Credit: FlipFarm USA, used with permission.
Permitting
The Florida Department of Agriculture and Consumer Services (FDACS) Division of Aquaculture issues and regulates all aquaculture leases within state waters. Only native species may be cultured in Florida. There are currently 26 aquaculture use zones in 10 coastal counties for submerged land leases. Each submerged land lease must be located in “approved” and/or “conditionally approved” shellfish harvesting areas; these classifications are defined and maintained by strict water quality guidelines. “Approved” areas are typically open to harvesting but may close due to sporadic events like harmful algal blooms (e.g., red tide), extreme storm events, and sewage spills, while “conditionally approved” areas are more regularly closed for harvesting due to more predictable pollution events driven by rainfall or flooding. Other classifications of shellfish harvesting areas include “prohibited” (harvesting not permitted) and “unclassified” (harvesting not permitted due to the lack of a management plan for the area) (FDACS 2021).
All commercial aquaculture operations must obtain an Aquaculture Certificate of Registration and comply with all Aquaculture Best Management Practices (BMPs) put forth by FDACS. Upon an approved lease application, FDACS will request a title determination from the Florida Department of Environmental Protection (DEP). The DEP will schedule a resource assessment (i.e., a visit to the proposed site) at which the suitability of the area for commercial operations will be determined. With resource assessment approval, an agency review package of the proposed site is sent to DEP, FWC, Florida Department of State, and the local Board of County Commissioners. In addition to this review, FDACS will alert property owners within 500 feet of the proposed lease site to generate any public comment including approval or opposition. Once approved by the state of Florida Board of Trustees, a professional site survey must be completed by a licensed surveyor to ensure no impediments or historical landmarks exist within the site. Only when the FDACS Division of Aquaculture approves the submitted site survey may the leaseholder apply for the aforementioned Aquaculture Certificate of Registration (FDACS 2020b). More information on lease acquisition can be found on the UF/IFAS Florida Shellfish Aquaculture online resource guide (UF/IFAS 2023).
Note that lease applications for operations in federal waters require consultation from the U.S. Army Corps of Engineers for programmatic general permits, the U.S. Environmental Protection Agency for wastewater discharges, and the U.S. Coast Guard for Private Aids to Navigation (PATON) requirements.
Summary
Declining wild oyster fisheries in Florida can be alleviated by commercial aquaculture to produce consistent product to market. Because wild fisheries relied on seafloor (on-bottom) oyster habitats, more advanced technology may be a solution for increased oyster production. The recent approval of water column use for oyster farming lends itself to deployment of floating bags and cages. Water column production yields high-quality oysters faster due to greater nutrient access. This method of production also increases the survivability of oysters by limiting predation.
Despite its potential benefits, floating oyster aquaculture must contend with increased biofouling. Biofouling organisms can overweight gear, decrease water flow, and compete with oysters for food sources, and, therefore, must be addressed (typically by flipping cages and bags). This additional husbandry creates a heavier workload for aquaculturists compared to the commonly used on-bottom culture, wherein oysters require significantly less maintenance. This industry necessity creates an opportunity for innovative technologies to be developed and used to automate production processes. Advanced technologies reduce manual labor inputs while maximizing the quality and quantity of oysters produced in the United States.
Oyster Aquaculture Glossary
Anoxic: completely without oxygen.
Biofouling: the accumulation of biological organisms (e.g., algae, tunicates, sponges, barnacles) on a cage contributing to reduced water exchange.
Broodstock: the reproductively mature adults held in a hatchery that are spawned to produce more individuals.
Cilia: short, numerous filaments that are present on many animal tissues and can be used for locomotion in small organisms.
Condition Index: relative fatness of an organism representing energy reserves.
Condition index = (dry meat weight in g)(100) / (internal cavity volume in cm³)
Cultch: material (such as oyster shell, stone, cement, etc.) functioning as substrate for oyster attachment.
Microcultch: Finely ground cultch (300 microns).
Density stocking: the number of oysters placed in a cage or bag.
Desiccation: the process of exposing an oyster cage to the air as a mechanism for drying it out to mitigate biofouling accumulation.
Diploid: an oyster with two sets of chromosomes that is able to reproduce sexually.
Flipping: the process of turning over an oyster cage or bag to its opposite side as a biofouling mitigation measure.
Gametes: Reproductive cells including female egg cells and male sperm.
Hand tonging: using long tongs to pluck wild oysters from the seafloor.
Husbandry: the continued care and cultivation of oysters.
Pediveliger: final stage oyster larvae that resembles the adult stage and eventually settles onto a substrate.
Seed: small oyster spat.
Triploid: a genetically altered oyster having a third set of chromosomes (instead of only two sets in diploid). Triploid oysters are sterile but grow faster than diploid oysters.
Trochophore: first stage oyster larvae that are free-swimming and have a rounded shape.
Tumbling: the process of shaking oysters within their cages.
Veliger: second stage oyster larvae that have large, ciliated lobes.
References
Botta, R., E. Camp, C. Court, C. Stair, and C. Adams. 2020. “Potential Economic Impacts of Restoring Commercial Oyster Harvest Levels in Apalachicola Bay, Florida FE1085, 10/2020” EDIS 2020 (6):5. https://doi.org/10.32473/edis-fe1085-2020
Brown, H. O., S. K. Jacobson, and A. E. Adams. 2021. “Defining Success for a Declining Resource: Mental Models of Oyster Management in Apalachicola, Florida.” Environmental Management 68:377–392. https://doi.org/10.1007/s00267-021-01495-6
CRC (Chesapeake Research Consortium). 2008. A framework for native oyster aquaculture development in Maryland. CRC, Report 08-166. Edgewater, MD.
FDACS (Florida Department of Agriculture and Consumer Services). 2020. Florida’s shellfish aquaculture. FDACS, Technical Bulletin P-02023.
FDACS (Florida Department of Agriculture and Consumer Services). 2020. Shellfish aquaculture leasing process. FDACS, Technical Bulletin P-01758-06.
FDACS (Florida Department of Agriculture and Consumer Services). 2021. Shellfish aquaculture lease management. FDACS, Technical Bulletin P-02154-05.
FFWCC (Florida Fish and Wildlife Conservation Commission). 2013. 2012–2013 Florida Gulf Coast Oyster Disaster Report.
Fish Farmer Magazine. 2022. “Grainocean present a comprehensive method of high-end production for oyster farms.” www.fishfarmermagazine.com
FlipFarm Systems Ltd. 2022. www.flipfarm.co.nz/
Havens, K., M. Allen, E. Camp, T. Irani, A. M. Lindsey, J. Glenn, A. Kane, D. Kimbro, S. Otwell, B. Pine, and C. Walters. 2013. Apalachicola Bay Oyster Situation Report. Publication FLSGP-T-13-001. Florida Sea Grant Program. https://repository.library.noaa.gov/view/noaa/39884
FOS (Florida Oceanographic Society). 2021. Oyster Gardening. https://www.floridaocean.org/oyster-gardening
Love, G., S. Baker, and E. V. Camp. 2021. “Oyster-Predator Dynamics and Climate Change: FA228, 09/2020.” EDIS 2021 (1):5. https://doi.org/10.32473/edis-fa228-2020
NCCOS (National Centers for Coastal Ocean Science). 2017. Modeling Approach to Predicting Nitrogen Removal by the Eastern Oyster Aquaculture Industry. https://coastalscience.noaa.gov/project/predicting-nitrogen-removal-by-the-eastern-oyster-aquaculture-industry/
ORP (Oyster Recovery Partnership). 2021. Oyster facts. https://www.oysterrecovery.org/news/oyster-facts
Petes, L. E., A. J. Brown, and C. R. Knight. 2012. “Impacts of Upstream Drought and Water Withdrawals on the Health and Survival of Downstream Estuarine Oyster Populations.” Ecology and Evolution 2 (7): 1712–1724. https://doi.org/10.1002/ece3.291
Pine III, W. E., C. J. Walters, E. V. Camp, R. Bouchillon, R. Ahrens, L. Sturmer, and M. E. Berrigan. 2015. “The Curious Case of Eastern Oyster Crassostrea virginica Stock Status in Apalachicola Bay, Florida”. Ecology and Society 20 (3): 46. https://doi.org/10.5751/ES-07827-200346
Solar Oysters LLC. 2023. www.solaroysters.com
UF/IFAS (University of Florida Institute of Food and Agricultural Sciences). 2023. Acquiring a Lease. https://shellfish.ifas.ufl.edu/getting-started/acquiring-a-lease/
UF/IFAS (University of Florida Institute of Food and Agricultural Sciences). 2019. Floating Gear Comparison for Off-Bottom Oyster Culture. www.shellfish.ifas.ufl.edu
Wallace, R. K. 2001. “Cultivating the Eastern Oyster, Crassostrea virginica.” SRAC (Southern Regional Aquaculture Center), Publication 432.
Wallace, R. K., P. Waters, and F. S. Rikard. 2008. “Oyster Hatchery Techniques.” SRAC (Southern Regional Aquaculture Center), Publication 4302.
Walters, L. J., P. E. Sacks, and D. E. Campbell. 2021. “Boating Impacts and Boat-Wake Resilient Restoration of the Eastern Oyster Crassostrea virginica in Mosquito Lagoon, Florida, USA.” Florida Scientist 84:173–199.
Walton, W. C., and L. Swann. 2021. “Role of Sea Grant in Establishing Commercial Oyster Aquaculture through Applied Research and Extension.” Journal of Contemporary Water Research & Education 174 (1): 171–179. https://doi.org/10.1111/j.1936-704X.2021.3367.x
Webster, D., and D. Meritt. 2004. “Stabilizing Oyster Ground.” Maryland Sea Grant Extension Factsheet, Publication UM-SG-MAP-88-04.