Introduction
More than half of the global population lives in urban areas that predominately rely on external supplies of resources (e.g., food, water, and energy) and ecosystem services. Ecosystem services are defined as benefits people obtain from nature, such as flood abatement, climate regulation, and landscape recreation. The United Nations projects that 2.5 billion more people will live on the planet by the mid-21st century, with disproportionate growth in urban areas (Forman and Wu 2016). Urbanization and rising resource demands in cities are further exacerbated by environmental changes such as climate and land-use change, economic fluctuations, and disturbances (e.g., natural disasters such as floods and hurricanes). Hence, it is crucial to explore pathways to achieve sustainable development in urban systems.
Urban agriculture, broadly defined as growing, processing, and distributing food related to crops and raised livestock directly for the urban market both within and on the fringe of urban areas, has been proposed as a viable solution to sustainable and resilient urban environments (Lovell 2010; Ackerman et al. 2014). Urban agriculture has undergone a global political uptick in momentum in recent decades and garnered increasing public attractions (Palmer 2018). For example, in the United States, urban agriculture began as early as the 1890s with community gardens emerging on vacant lots and continued with “victory gardens” during the Great Depression in the 1940s as promoted by the federal government and other public agencies. Movement in the 1970s led to the development of community gardens to support renewal of urban areas and to vitalize urban communities. These movements further expanded to many other cities and grew from individual grassroots efforts to coordinated programs for addressing issues such as food security, especially in the “food desert” regions and marginal communities. The need to develop urban agriculture is heightened in a planetary crisis like the COVID-19 pandemic, where global supply chains are disrupted and urban residents rely on local food productions (Cappelli and Cini 2020; Hobbs 2020). Many US cities, like Miami, Florida, are now experiencing an accelerated pace of urban agriculture development, which is also boosted by national initiatives such as Farm Bureau Urban County Farm Coalition and US Department of Agriculture Office of Urban Agriculture and Innovative Production. Outside the United States, other cities provide additional examples. Paris, France, for instance, opened the world’s largest urban farm (~14,000 m2) to feed residents and foster urban resilience (Harrap 2019).
Besides improving food security and enhancing local residents’ equitable access to healthy, fresh, and affordable food, urban agriculture can also deliver an array of ecosystem services that are often non-tangible, less recognized, and under-appreciated. This publication will elaborate on multiple ecosystem services provided by urban agriculture both within and across urban boundaries. Information in this publication will enrich the general public understanding of urban agriculture, foster public engagement into urban agricultural practices, and assist planners and decision-makers devising policies for land use, food, health, environment and agriculture in urban settings. Here we acknowledge that urban agriculture is highly diverse (Figure 1) and encompasses different types from the non-profit community garden to the commercial peri-urban farm, and forward into the future, with inspiring prototypes of vertical farms (Goldstein et al. 2016). The specific type of urban agriculture to a large extent determines the kind and combination of ecosystem services that can be delivered.
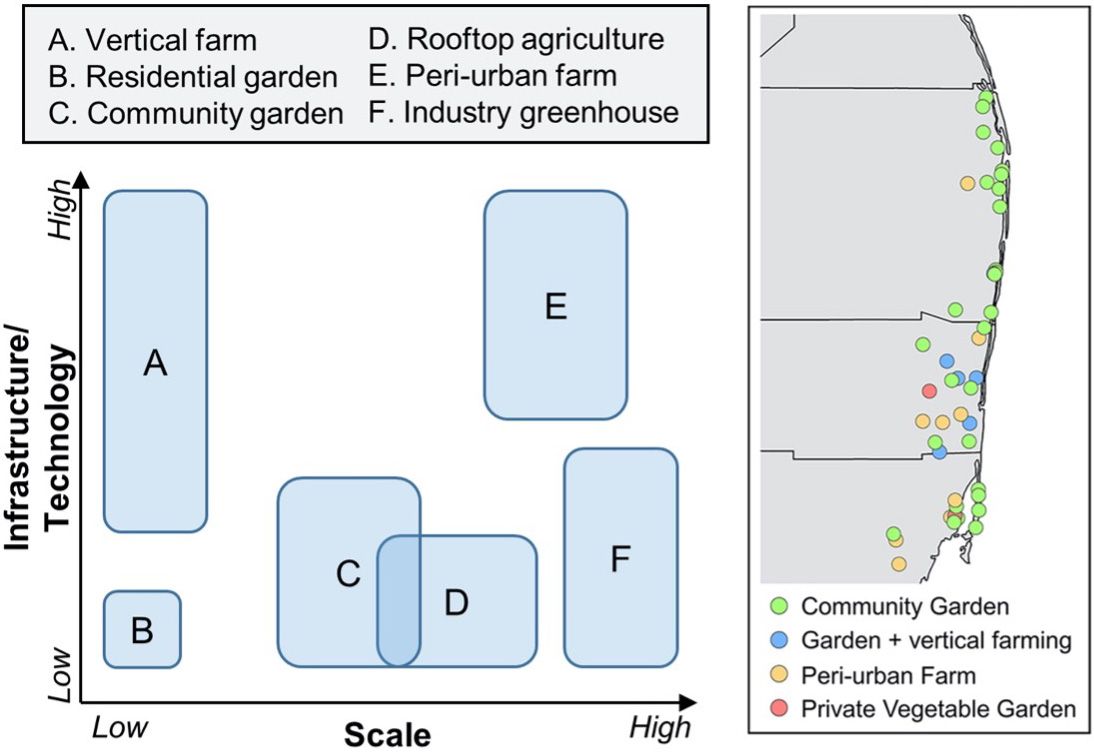
Credit: Jiangxiao Qiu, UF/IFAS
Ecosystem Services from Urban Agriculture Across Scales
In this section, we adopt the Millennium Ecosystem Assessment (MEA 2005) that categorizes ecosystem services into provisioning, regulating, and cultural services that directly benefit people. Provisioning services are the products directly derived from ecosystems such as food, fiber, and timber. Regulating services are the benefits obtained from regulation of ecological processes such as climate regulation, water flow regulation, and pest and disease regulation. Cultural services are the non-material benefits people obtain from ecosystems including aesthetic values, recreation and ecotourism, cultural heritage, educational access, etc.
Provisioning Services
Food production: One of the most obvious benefits of urban agriculture is with the production of food (e.g., fruits, vegetables, honey, poultry, milk, eggs, and other produce) in proximity to the consumers. In some cases, the food is directly consumed by the producers, improving food security for households. In other cases, much of the food is distributed through local markets, providing disposable income sources for individual residents and economic vitality for the community (e.g., commercial urban farms) (Lovell 2010). Such production potentials of urban agriculture should not be underestimated because (1) at present, ~15% of human-consumed food originates from within and in proximity to urban regions; and (2) a global analysis revealed that potentially 100–180 million metric tons of food can be produced with urban agriculture (Clinton et al. 2018). Recent advances in urban agriculture also include precision farming (i.e., farm management that uses information technology to ensure that crops and soils receive what they need for optimal health and productivity) and vertical farming (i.e., the practice of growing crops in vertically stacked layers). These practices incorporate techniques that can optimize resource use efficiency and substantially increase yields. They may even be expandable to include staple crops (e.g., wheat) that are currently not feasible in the urban setting (Asseng et al. 2020). Besides increasing food quantity, localizing food production can also contribute to addressing residents’ diet-related chronic diseases that result from limited access to healthy, nutritious, and fresh food (e.g., diabetes, obesity, heart disease) (Orsini et al. 2013).
Regulating Services
Microclimate and air quality regulation: Urban agriculture also has the potential to regulate the urban microclimate. For example, through increasing vegetated land cover in the urban centers, urban agriculture could mitigate the “urban heat island” effect, a phenomenon where air temperature in the urban centers is consistently higher than in the urban fringes and rural areas due to concentrations of pavement, buildings, and other surface structures that absorb and retain heat. A review of global cities revealed that urban temperature can be reduced by 0.5°C to 4.0°C through humidity control and evapotranspiration (i.e., processes by which water moves from the ground into the atmosphere) related to urban agriculture (Qiu et al. 2013). In addition, urban agriculture could deliver air purification by absorbing particulate matter, acidic gaseous chemicals, and other air pollutants suspended in the atmosphere. Previous studies have estimated that 711,000 metric tons of air pollutants are removed annually by urban trees (Yang, Yu, and Gong 2008). However, in densely populated urban centers, it can be impossible to plant enough trees to improve air quality, and under such situations, urban agriculture like green roofs and rooftop gardens can also be encouraged to provide air purification.
Stormwater retention and management: Through increased infiltration (i.e., the process by which water flows into the ground) and higher evapotranspiration, urban agriculture areas have the capacity to retain stormwater and reduce surface runoff and peak flows, as compared to built-up and impervious surface areas. Studies have shown that green roofs can retain between 52.3% and 100% of precipitation, thus reducing the amount of stormwater runoff (Berndtsson 2010). Such benefits can be directly applicable to urban agriculture through the form of rooftop agriculture that has garnered increasing interest in large metropolitan regions such as New York City, Portland, and Chicago. Other studies also revealed that, if vacant lots were converted to urban agriculture and community gardens, it could reduce stormwater runoff by 85% (Hankard et al. 2016). At the global scale, the benefits of potential stormwater mitigation from urban agriculture are equivalent to between 45 and 57 billion cubic meters each year (Clinton et al. 2018).
Waste management and nutrient recycling: Urban agriculture could contribute to urban waste management by providing composting as an alternative means of disposing organic waste (e.g., food or human wastes). For example, in New York City, in 2009 more than 130 community gardens had composting activities, which were integrated with the existing city sanitation policy and plans (Azunre et al. 2019). Studies in Glasgow, Scotland, and Lyon, France, have also revealed that an integrated urban agriculture system could assimilate between 16.9% and 51.7% of food waste (Weidner and Yang 2020). In addition, urban agriculture could facilitate the treatment and recycling of wastewater by using wastewater for irrigation to conserve water and recover nutrients (Miller-Robbie , Ramaswami, and Amerasinghe 2017). Lydecker and Drechsel (2010) have estimated that vegetable farms in Accra, Ghana, could treat the wastewater from ~225,000 households. However, studies have also raised potential concerns, including increased health risks and pathogen and heavy metal contamination associated with the uses of untreated wastewater in urban agriculture (Ensink, Blumenthal, and Brooker 2008). Practices such as regular soil and water testing, soil remediation (i.e., procedures to remove contaminants from soils), crop selection (e.g., choosing crops with low heavy metal accumulation), and proper sanitation measures in processing and postharvest handling of food are crucial to ensure food safety.
Biodiversity conservation: Urban agriculture can contribute to biodiversity conservation in urban landscapes that are typically highly simplified and intensively developed with low levels of native biodiversity (Lin and Fuller 2013). Specifically, by providing diverse and structurally complex green infrastructure, urban agriculture can serve as critical habitat for a range of flora and fauna like native plants, insects, birds, vertebrates, and soil invertebrates (Lin, Philpott, and Jha 2015). Across the entire urban landscape, urban agriculture could enhance biodiversity in adjacent locations due to the “spill over” of energy, resources, genetics and organisms across habitats, which are key for the persistence of diverse species in human-dominated landscapes (Blitzer et al. 2012). All these positive effects on biodiversity from urban agriculture can further produce other ecosystem services, such as pollination from the diverse assemblage of bees and butterflies it enables and pest regulation from the persistence of diverse natural enemies.
Cultural Services
Compared to conventional and rural agriculture, the integration of urban agriculture into densely populated urban areas substantially extends the opportunities to combine food production with social-cultural functions and use of urban green space (Lovell 2010). These social-cultural services of urban agriculture can be challenging to quantify, but are vital to improved life quality of urban residents. Specifically, urban agriculture can provide landscape aesthetics, sense of place, and connections of residents with land and nature (Pearson, Pearson, and Pearson 2010). In addition, community-based forms of urban agriculture could improve social interactions, community building (Galluzzi, Eyzaguirre, and Negri2010) and civic engagement (Obach and Tobin 2014). Moreover, urban agriculture (e.g., community gardens, home gardens, and rooftop gardens) also offers a place for indoor and outdoor recreation, as well as for achieving personal happiness, wellbeing, and mental, psychological, and physical health (Brown and Jameton 2000; Douglas 2012). Last but not least, urban agriculture can also provide a medium for learning experiences, youth development, and educational programs. School and community gardens can be used to guide informed decisions about food systems, for instance (Azunre et al. 2019).
Simultaneous Effects of Urban Agriculture on Food, Water, and Energy Resources
Urban agriculture can simultaneously affect food, water, and energy resources, all of which are interlinked in their flows and consumptions, an interaction known as the food-water-energy nexus. The food-water-energy nexus refers to the intricate linkages among supply and consumption of food, water, and energy resources, meaning that actions in one sector could influence the other sectors. The impacts of urban agriculture on ecosystem services can extend beyond the local scale and indeed have important regional and global implications for climate mitigation, water and energy conservation, and improved environmental footprints through the food and resource supply chains (Chang et al. 2020b; 2020a) (Figure 2). Such cross-scale effects in the food-water-energy nexus are often inexplicit and seldom considered in the context of urban decision-making. Specifically, by localizing food production and supply, urban agriculture can reduce external food imports and thus mitigate energy consumption and greenhouse gas emissions associated with the packing, transporting, and storage of food along the supply chain, which accounts for up to 75% of total energy consumption in the food system (Pimentel et al. 2008; Mohareb et al. 2017). In addition, urban agriculture’s effects on the urban heat island and microclimate may lower urban energy demands and external energy supplies for cooling, thus reducing carbon emissions from energy transportation and generation. Energy benefits from urban agriculture could further cascade to water savings, given that water is often required for cooling power plants in generating energy supplies. Indeed, recent comprehensive research provided evidence that urban agriculture could be an effective strategy to combat climate change through increased carbon sequestration and reduced energy use and greenhouse emissions (Lwasa et al. 2015). However, these sustainability implications would be more meaningful if urban agriculture could be scaled up in amount, size, and area, instead of being limited to small-scale implementations (Daigger et al. 2015).
In addition, urban agriculture could reduce irrigation needs relative to the traditional large-scale farming. A vertical farm, for instance, uses up to 95% less water than a field farm (Daigger et al. 2015; Qiu, Bayabil, and Li 2020). Urban agriculture would also foster water savings if water-intensive urban lawns were converted to vegetables (Figure 2). For example, research shows that, in the state of Florida, >60% of domestic water irrigates lawns, and it goes up to 88% in the summer (Haley, Dukes, and Miller 2007). All these water benefits from urban agriculture are non-trivial, given the accelerated drawdown and depletion of groundwater across the globe (Aeschbach-Hertig and Gleeson 2012; Dalin et al. 2017). Water savings also mean that less energy is being consumed to pump and distribute water. Further, urban agriculture can also reduce water embedded (or “hidden”) in food products (especially water-intensive crops such as almond and avocado) and lower water footprints and impacts to other external food supply regions (Zimmer and Renault 2003; Dalin et al. 2017).
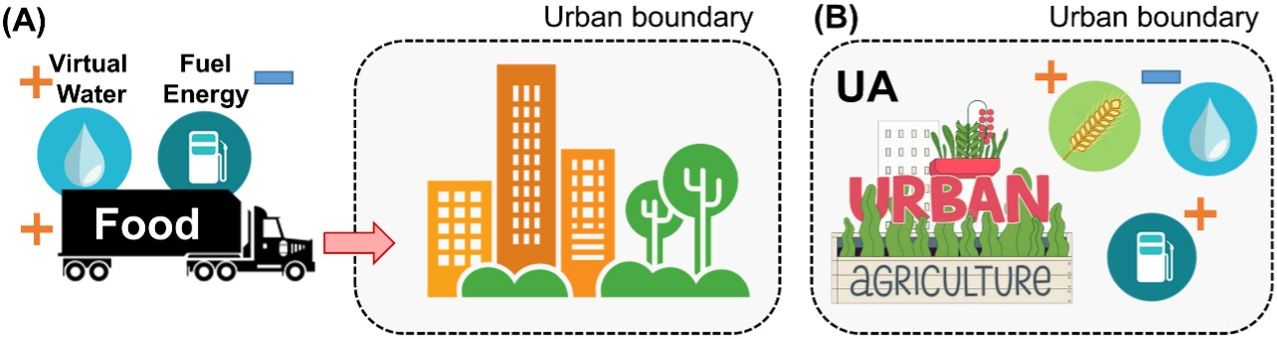
Credit: Jiangxiao Qiu, UF/IFAS
Conclusions
This publication adopts a holistic perspective to summarize the portfolio of provisioning, regulating, and cultural ecosystem services that can be delivered by urban agriculture and their effects across local, regional, and global scales through resource supply chains. While certain implications of urban agriculture on ecosystem services are obvious (e.g., food production, biodiversity), others can be intangible and obscure and sometimes dependent upon the scale of reference (e.g., local vs. regional and global) and the type of urban agriculture. It is important to note that (1) there are other vital economic benefits associated with urban agriculture (e.g., employment, disposable income, tax revenue, diversified industry base) that are not discussed at length here but are reviewed elsewhere (e.g., Azunre et al. 2019); (2) urban agriculture may have potential risks, tradeoffs, and disservices (e.g., pollution, pests, pathogens, diseases, health risks), which will require more thorough investigation on how and the extent to which these risks can be minimized; and (3) technology and its innovation and integration with urban agriculture (e.g., vertical farming, hydroponics) (Chang et al. 2020b; Qiu, Bayabil, and Li 2020), while beyond the scope of this publication, is a promising avenue for research and application and can be critical for ultimately scaling up urban agriculture, reducing tradeoffs, and archiving the full sustainability benefits from urban agriculture.
References
Ackerman, K., M. Conard, P. Culligan, R. Plunz, M.-P. Sutto, and L. Whittinghill. 2014. "Sustainable Food Systems for Future Cities: The Potential of Urban Agriculture." The Economic and Social Review 45:189–206.
Aeschbach-Hertig, W., and T. Gleeson. 2012. "Regional Strategies for the Accelerating Global Problem of Groundwater Depletion." Nature Geoscience 5:853–861. https://doi.org/10.1038/ngeo1617
Asseng, S., J. R. Guarin, M. Raman, O. Monje, G. Kiss, D. D. Despommier, F. M. Meggers, and P. P. G. Gauthier. 2020. Wheat Yield Potential in Controlled-Environment Vertical Farms." Proceedings of the National Academy of Sciences. https://doi.org/10.1073/pnas.2002655117
Azunre, G. A., O. Amponsah, C. Peprah, S. A. Takyi, and I. Braimah. 2019. A Review of the Role of Urban Agriculture in the Sustainable City Discourse." Cities 93:104–119. https://doi.org/10.1016/j.cities.2019.04.006
Berndtsson, J. C. 2010. Green Roof Performance towards Management of Runoff Water Quantity and Quality: A Review." Ecological Engineering 36:351–360. https://doi.org/10.1016/j.ecoleng.2009.12.014
Blitzer, E. J., C. F. Dormann, A. Holzschuh, A.-M. Klein, T. A. Rand, and T. Tscharntke. 2012. Spillover of Functionally Important Organisms between Managed and Natural Habitats." Agriculture, Ecosystems & Environment 146:34–43. https://doi.org/10.1016/j.agee.2011.09.005
Brown, K. H., and A. L. Jameton. 2000. "Public Health Implications of Urban Agriculture." Journal of Public Health Policy 21:20–39. https://doi.org/10.2307/3343472
Cappelli, A., and E. Cini. 2020. "Will the COVID-19 pandemic make us reconsider the relevance of short food supply chains and local productions?" Trends in Food Science & Technology 99:566. https://doi.org/10.1016/j.tifs.2020.03.041
Chang, N.-B., U. Hossain, A. Valencia, J. Qiu, and N. Kapucu. 2020a. "The Role of Food-Energy-Water Nexus Analyses in Urban Growth Models for Urban Sustainability: A Review of Synergistic Framework." Sustainable Cities and Society:102486. https://doi.org/10.1016/j.scs.2020.102486
Chang, N.-B., U. Hossain, A. Valencia, J. Qiu, Q. P. Zheng, L. Gu, M. Chen, J.-W. Lu, A. Pires, and C. Kaandorp. 2020b. "Integrative Technology Hubs for Urban Food-Energy-Water Nexuses and Cost-Benefit-Risk Tradeoffs (II): Design Strategies for Urban Sustainability." Critical Reviews in Environmental Science and Technology 51 (14): 1533–1583. https://doi.org/10.1080/10643389.2020.1761088
Clinton, N., M. Stuhlmacher, A. Miles, N. U. Aragon, M. Wagner, M. Georgescu, C. Herwig, and P. Gong. 2018. "A Global Geospatial Ecosystem Services Estimate of Urban Agriculture." Earth’s Future 6 (1): 40–60. https://doi.org/10.1002/2017EF000536
Daigger, G. T., J. P. Newell, N. G. Love, N. McClintock, M. Gardiner, E. Mohareb, M. Horst, J. Blesh, and A. Ramaswami. 2016. "Scaling Up Agriculture in City-Regions to Mitigate FEW System Impacts." Ann Arbor, MI: School of Natural Resources and Environment. Department of Civil and Environmental Engineering, University of Michigan. 68 pages. https://css.umich.edu/publications/research-publications/scaling-agriculture-city-regions-mitigate-few-system-impacts
Dalin, C., Y. Wada, T. Kastner, and M. J. Puma. 2017. "Groundwater Depletion Embedded in International Food Trade." Nature 543:700–704. https://doi.org/10.1038/nature21403
Douglas, I. 2012. "Urban Ecology and Urban Ecosystems: Understanding the Links to Human Health and Well-Being." Current Opinion in Environmental Sustainability 4 (4): 385–392. https://doi.org/10.1016/j.cosust.2012.07.005
Ensink, J. H., U. J. Blumenthal, and S. Brooker. 2008. "Wastewater Quality and the Risk of Intestinal Nematode Infection in Sewage Farming Families in Hyderabad, India." The American Journal of Tropical Medicine and Hygiene 79 (4): 561. https://doi.org/10.4269/ajtmh.2008.79.561
Forman, R. T. T., and J. Wu. 2016. "Where to Put the Next Billion People." Nature 537:608–611. https://doi.org/10.1038/537608a
Galluzzi, G., P. Eyzaguirre, and V. Negri. 2010. "Home Gardens: Neglected Hotspots of Agro-biodiversity and Cultural Diversity." Biodiversity and Conservation 19:3635–3654. https://doi.org/10.1007/s10531-010-9919-5
Goldstein, B., M. Hauschild, J. Fernández, and M. Birkved. 2016. "Urban Versus Conventional Agriculture, Taxonomy of Resource Profiles: A Review." Agronomy for Sustainable Development 36:9. https://doi.org/10.1007/s13593-015-0348-4
Harrap, C. 2019. "World's Largest Urban Farm to Open--on a Paris Rooftop." The Guardian https://www.theguardian.com/cities/2019/aug/13/worlds-largest-urban-farm-to-open-on-a-paris-rooftop
Haley, M. B., M. D. Dukes, and G. L. Miller. 2007. "Residential Irrigation Water Use in Central Florida." Journal of Irrigation and Drainage Engineering 133 (5): 427–434. https://doi.org/10.1061/(ASCE)0733-9437(2007)133:5(427)
Hankard, M., M. Reid, R. Schaefer, K. Vang, and C. Small. 2016. "Stormwater Runoff Benefits of Urban Agriculture." St. Paul, MN, USA: University of St. Thomas. Available online https://www. stthomas. edu/media/officeofsustainability/spring2016projectreports/UrbanAgStor mwaterReport. pdf.
Hobbs, J. E. 2020. "Food Supply Chains during the COVID‐19 Pandemic." Canadian Journal of Agricultural Economics/Revue Canadienne D’Agroeconomie. https://doi.org/10.1111/cjag.12237
Lin, B. B., and R. A. Fuller. 2013. "Sharing or sparing? How should we grow the world’s cities?" Journal of Applied Ecology 50 (5): 1161–1168. https://doi.org/10.1111/1365-2664.12118
Lin, B. B., S. M. Philpott, and S. Jha. 2015. "The Future of Urban Agriculture and Biodiversity—Ecosystem Services: Challenges and Next Steps." Basic and Applied Ecology 16 (3): 189–201. https://doi.org/10.1016/j.baae.2015.01.005
Lovell, S. T. 2010. "Multifunctional Urban Agriculture for Sustainable Land Use Planning in the United States." Sustainability 2 (8): 2499–2522. https://doi.org/10.3390/su2082499
Lwasa, S., F. Mugagga, B. Wahab, D. Simon, J. P. Connors, and C. Griffith. 2015. "A Meta-Analysis of Urban and Peri-Urban Agriculture and Forestry in Mediating Climate Change." Current Opinion in Environmental Sustainability 13:68–73. https://doi.org/10.1016/j.cosust.2015.02.003
Lydecker, M., and P. Drechsel. 2010. "Urban Agriculture and Sanitation Services in Accra, Ghana: The Overlooked Contribution." International Journal of Agricultural Sustainability 8 (1–2): 94–103. https://doi.org/10.3763/ijas.2009.0453
MEA. 2005. Ecosystems and Human Well-being: Synthesis. Island Press, Washington, DC.
Miller-Robbie, L., A. Ramaswami, and P. Amerasinghe. 2017. "Wastewater Treatment and Reuse in Urban Agriculture: Exploring the Food, Energy, Water, and Health Nexus in Hyderabad, India." Environmental Research Letters 12:075005. https://doi.org/10.1088/1748-9326/aa6bfe
Mohareb, E., M. Heller, P. Novak, B. Goldstein, X. Fonoll, and L. Raskin. 2017. "Considerations for Reducing Food System Energy Demand while Scaling up Urban Agriculture." Environmental Research Letters 12 (12): 125004. https://doi.org/10.1088/1748-9326/aa889b
Obach, B. K., and K. Tobin. 2014. "Civic Agriculture and Community Engagement." Agriculture and Human Values 31 (2): 307–322. https://doi.org/10.1007/s10460-013-9477-z
Palmer, L. 2018. "Urban Agriculture Growth in US Cities." Nature Sustainability 1:5–7. https://doi.org/10.1038/s41893-017-0014-8
Pearson, L. J., L. Pearson, and C. J. Pearson. 2010. "Sustainable Urban Agriculture: Stocktake and Opportunities." International Journal of Agricultural Sustainability 8 (1–2): 7–19. https://doi.org/10.3763/ijas.2009.0468
Pimentel, D., S. Williamson, C. E. Alexander, O. Gonzalez-Pagan, C. Kontak, and S. E. Mulkey. 2008. "Reducing Energy Inputs in the US Food System." Human Ecology 36:459–471. https://doi.org/10.1007/s10745-008-9184-3
Qiu, G., H. Li, Q. Zhang, W. Chen, X. Liang, and X. Li. 2013. "Effects of Evapotranspiration on Mitigation of Urban Temperature by Vegetation and Urban Agriculture." Journal of Integrative Agriculture 12 (8): 1307–1315. https://doi.org/10.1016/S2095-3119(13)60543-2
Qiu, J., H. K. Bayabil, and Y. Li. 2020. "Indoor Vertical Farming Systems for Food Security and Resource Sustainability." FOR360 EDIS 2020 (2): 5. https://doi.org/10.32473/edis-fr429-2020
Sonnino, R., C. L. Tegoni, and A. De Cunto. 2019. "The Challenge of Systemic Food Change: Insights from Cities." Cities 85:110–116. https://doi.org/10.1016/j.cities.2018.08.008
Weidner, T., and A. Yang. 2020. "The Potential of Urban Agriculture in Combination with Organic Waste Valorization: Assessment of Resource Flows and Emissions for Two European Cities." Journal of Cleaner Production 244:118490. https://doi.org/10.1016/j.jclepro.2019.118490
Yang, J., Q. Yu, and P. Gong. 2008. "Quantifying Air Pollution Removal by Green Roofs in Chicago." Atmospheric Environment 42 (31): 7266–7273. https://doi.org/10.1016/j.atmosenv.2008.07.003
Zimmer, D., and D. Renault. 2003. "Virtual Water in Food Production and Global Trade: Review of Methodological Issues and Preliminary Results." Pages 1–19 Virtual water trade: Proceedings of the International Expert Meeting on Virtual Water Trade, Value of Water Research Report Series.