Microalgae are primary producers in marine and freshwater ecosystems and serve as direct food sources for many aquatic organisms, especially filter feeders and larval organisms. Therefore, microalgae play an important role in fish and molluscan shellfish aquaculture. Depending on cell size, structure, nutrient value, digestibility, and ease of culture, over a dozen microalgal species have been selected for molluscan bivalve aquaculture (Davis and Guillard 1958; Guillard 1975; Creswell 2010). The culture of microalgae is costly and can account for about 40% of hatchery expenses for the production of bivalve seed (Helm and Bourne 2004). To ensure the effective use of microalgae without overfeeding bivalves in hatcheries, measurement of microalgae concentration is required.
In this publication, a method for the determination of microalgal concentration was developed using turbidity measurements. This method can be used by commercial shellfish hatcheries when calculating the volume of microalgae to use for the proper feeding of larvae and broodstock. Standard equations were generated based on linear relationships between turbidity measurement and cell concentration. Cell concentration was validated using hemocytometer counts of four commonly used microalgae in shellfish hatcheries. These standard equations will provide a quick, accurate, and easy-to-use method for the determination of microalgal concentration in hatcheries.
Table 1. The standard equations, linear correlations, and effective turbidity ranges between microalgal concentrations were estimated by use of hemocytometer and turbidity measurements for four microalgal species. The R2 values (close to 1) indicated the strong linear correlation between microalgal concentration and turbidity measures. X: turbidity measurement (NTU); Y: microalgal concentration (cells/ml).
How to Use These Standard Equations and Considerations
To minimize the effects of color on turbidity measurement, select a turbidity meter that complies with the ISO 0727 standard with a light source over an 800-nm wavelength. To obtain an estimated microalgal concentration, the steps are to measure the turbidity of the microalgal culture in NTU (nephelometric turbidity units) and then plug the turbidity value into the X variable for the equation provided for that species (Table 1). The resulting Y value will be the microalgal concentration in cells/ml. For example, if the turbidity of Tetraselmis suecica culture was measured as 391 NTU, the concentration would be calculated as 2.10 × 106 cells/ml, while the turbidity of Isochrysis galbana culture measured as 89 NTU would have a concentration calculated as 1.01 × 107 cells/ml. In practice, a spreadsheet can be generated to list the turbidity readings and their matching algal concentrations for convenient use of equations.
When using the standard equations for the estimation of microalgal concentrations, users must consider several factors:
- Effective turbidity range. The equations are effective only within the effective ranges of turbidity measurements (Table 1). In general, microalgal culture stocks in most hatcheries rarely reach a density beyond the effective ranges.
- Instrument calibration and maintenance. Before the measurement of samples, the turbidity meter must be calibrated following the manufacturer’s manual with the provided standard samples. Sample bottles or cuvettes need to be cleaned using distilled water before loading samples, and the outside surface of sample bottles or cuvettes needs to be wiped dry and clean. When measuring the turbidity, microalgal samples need to be mixed well but without the creation of any bubbles, which may affect the turbidity reading.
- Purity of microalgal culture. The use of uncontaminated microalgal cultures is important because different sizes and morphologies of contaminating cells can influence turbidity readings. Therefore, if microalgal cultures are heavily contaminated and mixed with other species, concentration estimation by turbidity measurement may not be reliable.
- Application of these equations to other microalgal species. Because turbidity readings of suspended microalgae will be affected by individual species’ cell sizes, shapes, colors, and reflectivity, a new set of standard equations must be generated for each species of microalgae. However, for species with comparable similarity of cell sizes and morphology, such as Isochrysis galbana (clone T, T-Iso) and I. galbana (clone C, C-ISO), the same equation can likely be applied.
How did we generate the standard equations?
The process used to generate the standard equations included the following steps:
- Preparation of serial dilution samples. Four commonly used microalgal species in shellfish hatcheries, Tetraselmis suecica, Isochrysis galbana (T-ISO), Chaetoceros calcitrans, and Chaetoceros gracilis (diatoms), were chosen for this study. First, each microalgae species was concentrated by centrifugation at 3,000 rpm for 5 minutes at room temperature (T = 22°C), and concentrated microalgae were serially diluted by a factor of 2× with artificial seawater (25 ppt, same as that for algal culture). Thus, a series of microalgal suspensions at different concentrations was generated (Figure 1) for measurements of their turbidity and actual cell concentration. For each microalgal species, three sets of serial samples were tested as replicates.
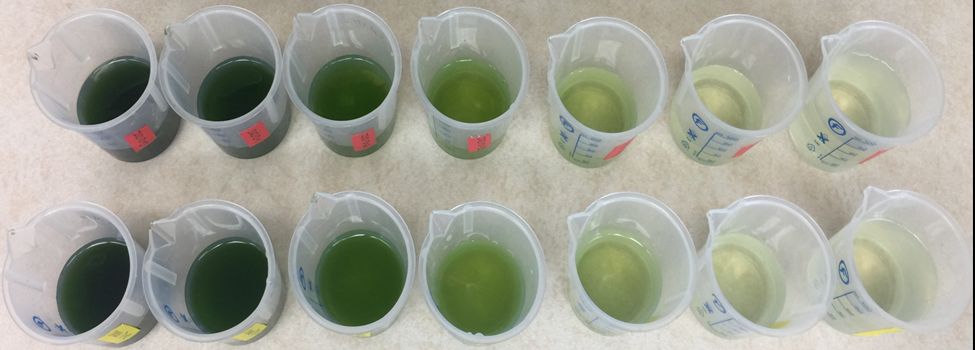
Credit: Huiping Yang, UF/IFAS
2. Measurement of turbidity. A turbidity meter (Type 860040, Sper Scientific, ~$400) was used to measure the turbidity of microalgal samples. This turbidity meter follows the ISO 7027 nephelometric standard with an 850-nm LED as a light source, and the effective range is 0 to 1000 NTU (nephelometric turbidity unit) with an accuracy of 0.5 NTU (within the ranges of 0.00-49.99 NTU) or 5 NTU (within the ranges of 50– 1000 NTU). For each sample, turbidity measurement was performed three times with a 10-ml sample to minimize instrumental errors, and the mean values were recorded as the turbidity for each sample.
3. Measurement of microalgal concentration by use of hemocytometer count. Actual cell concentrations of each sample were counted by use of a hemocytometer (Hausser Bright-Line, Hausser Scientific). One ml of microalgal suspension from each of the serial samples was removed and fixed by adding 10 µl of Lugol’s fixative (1%, 20 g potassium iodide, 10 g iodine crystals, 200 mL of distilled water containing 20 mL glacial acetic acid). The standard protocol for hemocytometer cell counting recognized by the World Health Organization was followed. A 10-µl fixed sample was loaded onto the hemocytometer and counted at 200× magnification using a microscope (Olympus BX43, Olympus). Each sample was counted in duplicates, and the mean value was used as the cell concentration of each sample.
4. Linear correlation between turbidity readings and actual concentrations. Significant linear correlations between turbidity measurements and cell concentrations were obtained for all four algae species (P < 0.001) (Figure 2). The standard equations for each microalgal species were generated and the coefficient of determination ranged from 0.930 to 0.988, indicating the reliability of the standard equations (Table 1).
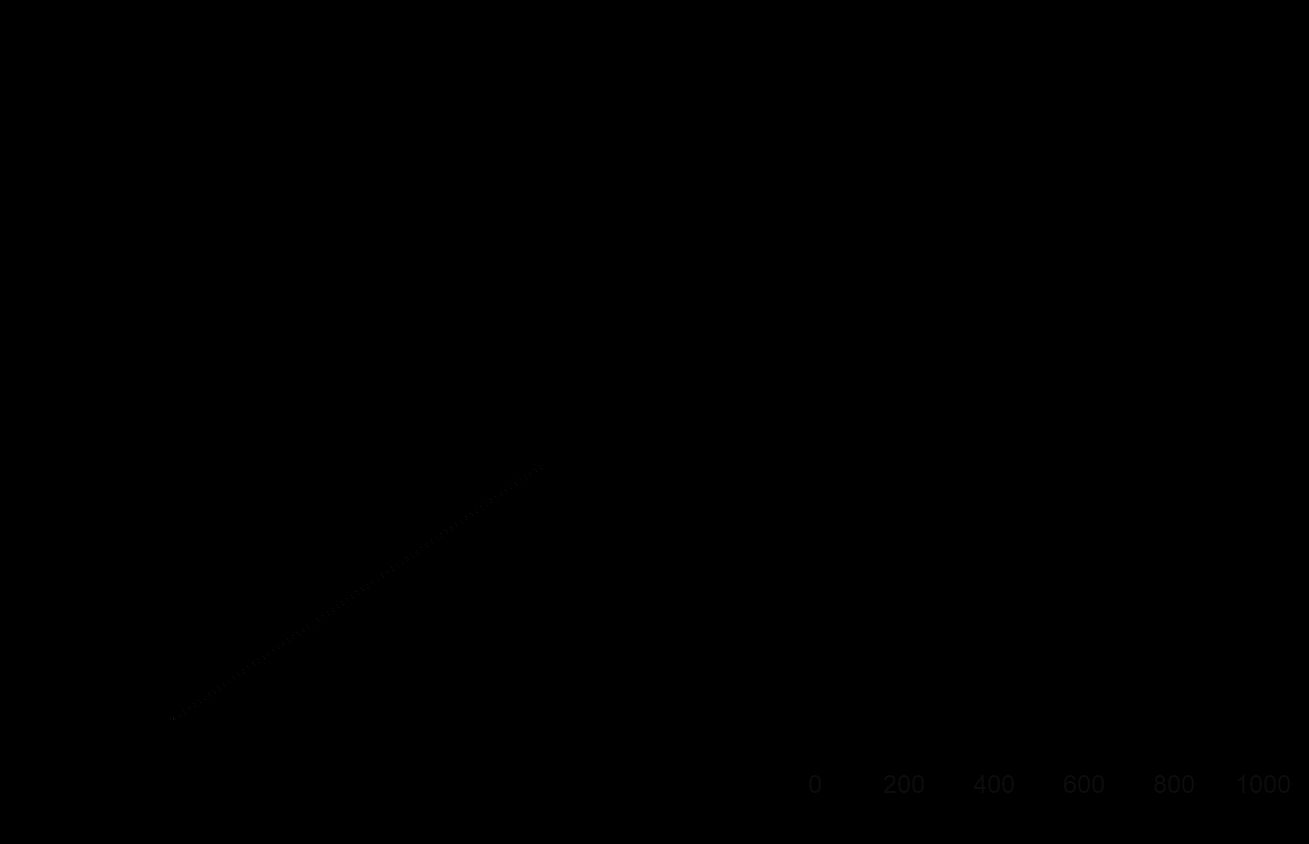
Credit: Huiping Yang and Jayme Yee, UF/IFAS
5. Validation of the standard equations. To validate the standard equations, microalgal cultures from an industry hatchery were sampled and quantified by these equations using the turbidity meter and compared to actual hemocytometer counts (data not shown). No differences were found in each of the four microalgal species (P ≥ 0.174), indicating the accuracy of the standard equations for cell concentration evaluation by measuring turbidity.
What is turbidity and how can it be used to determine microalgal concentration?
Turbidity means, essentially, “murkiness.” It describes the reduced transparency of water due to the presence of suspended particulates and dissolved organic materials. Turbidity can be recorded in two ways: 1) Nephelometry: A measurement of the incident light scattered at an angle of 90 degrees from the sample and presented as nephelometric turbidity units (NTU), and 2) Turbidimetry: A measurement of the decrease in transmitted light through the sample at an angle of 180 degrees and presented as Formazin Attenuation Units (FAU). Generally, the nephelometry method is more accurate and suitable for lower turbidity solutions.
A turbidity reading is highly correlated to the presence of suspended particles and dissolved light-absorbing substances. Factors that influence turbidity include:
- Particle concentration. The higher the particle concentration, the higher the turbidity measurement. This is the mechanism used to establish standard equations for the estimation of cell concentrations through turbidity measurements.
- Particle or suspension color. The color of the suspended particles and solution can affect the turbidity reading. However, this effect can be diminished when the wavelength of a light source is greater than 800 nm. The turbidity meter used in this study equips an 850-nm LED as its light source, therefore, the color of algal cultures did not affect the turbidity measurement.
- Particle size and morphology. The size and morphology of suspended particles can contribute differently to turbidity readings, especially for particles larger than 5 μm. The four microalgal species studied in this study have different cell sizes and morphologies: Tetraselmis suecica: 300 µm3, Isochrysis galbana: 40–50 µm3, Chaetoceros calcitrans: 35 µm3, Chaetoceros calcitrans: 80 µm3 (Helm and Bourne 2004). Therefore, at the same concentration, the four microalgal species showed different turbidity, and at the same turbidity, the four microalgal species showed different cell concentrations.
What are the general methods for measurement of microalgal concentration?
Generally, determination of microalgae concentration and other single-cell suspensions, such as blood, sperm, and bacteria, can be achieved through the following approaches.
- Direct count. Direct count is usually conducted by use of counting chambers, such as a hemocytometer, Makler counting chamber, or Sedgewick-rafter counting chamber (for larger-sized samples). Samples need to be distributed homogeneously throughout the grids for accurate cell counts within a certain known volume. Direct counting is an accurate approach with affordable devices (~$200–500 per hemocytometer). This method is time-consuming (about 20–30 min per sample) and requires a microscope.
- Measurement of optical properties of single-cell suspensions. Suspended cells can absorb or scatter light passing through a suspension. Thus, the measurement of optical properties, such as absorbance or transmission, could be used for the estimation of cell concentrations. Instruments, such as a spectrophotometer, turbidity meter, and microplate reader, have been used to estimate the concentration of sperm, bacteria, and phytoplankton. For cells with auto-fluorescent chlorophylls, fluorometry can be used to evaluate cell concentration through the measurement of fluorescence intensity. For these methods, linear correlations between cell concentration and optical properties need to be established and validated. The cost for these instruments varies from several hundred dollars (e.g., the turbidity meter in this study) to thousands of dollars.
- Use of single-cell fluidics system. Single-cell fluidic systems, such as the coulter counter, flow cytometer, or microfluidics, have the basic function to count the cell number passing through a detection apparatus. Single-cell fluidics system is accurate and fast (several seconds per sample), but the instruments themselves are usually expensive (more than $20,000).
Different methods, including direct count, use of optical properties, and chemical estimation of carbon capacity and chlorophyll α, have been compared for quantification of Asterionella formosa, and precision, limit of detection, and sensitivity were determined at cell concentration (Butterwick et al. 1982). In bivalve hatcheries, direct count by use of a hemocytometer is generally recommended for microalgal concentration measurement (Helm and Bourne 2004), but turbidity meters are not commonly used yet. We developed linear standard equations for microalgae concentration counted using a hemocytometer and turbidity values recorded by a turbidity meter. These equations could be applied by commercial hatcheries for quick estimation of microalgal concentrations by measuring turbidity.
Acknowledgements
This study was supported by funds from the Gulf States Marine Fisheries Commission, a National Sea Grant special workshop project (NA17OAR4170227), and a National Sea Grant Aquaculture Initiative Award (NA18OAR4170344).
References
Butterwick, C., S. I. Heaney, and J. F. Talling. 1982. "A Comparison of Eight Methods for Estimating the Biomass and Growth of Planktonic Algae." British Phycological Journal. 17:69–79. https://doi.org/10.1080/00071618200650091
Creswell, L., 2010. "Phytoplankton Culture for Aquaculture Feed." Southern Regional Aquaculture Center, SRAC Publication No. 5004.
Davis, H. C., R. R. L. Guillard. 1958. "Relative Value of Ten Genera of Micro-organisms as Food for Oyster and Clam Larvae." Fishery Bulletin 136, Fishery Bulletin of the Fish and Wildlife Service. 58:293–304.
Guillard, R. 1975. "Culture of Phytoplankton for Feeding Marine Invertebrates" in Culture of Marine Invertebrate Animals: Proceedings — 1st Conference on Culture of Marine Invertebrate Animals Greenport, edited by W. L. Smith and M. H. Chanley. Springer US, Boston, MA, pp. 29–60. https://doi.org/10.1007/978-1-4615-8714-9_3
Helm, M. M., and N. Bourne. 2004. "Hatchery Culture of Bivalves: A Practical Manual" inFAO Fisheries Technical Paper. No. 471, edited by A. Lovatelli. Rome, FAO, pp. 177.