Abstract
The redbay ambrosia beetle, harmless in its native Asia, has become a formidable pest since its introduction to the United States in 2002. The beetle spreads the fungus Harringtonia lauricola, a lethal pathogen of North American Lauraceae trees, including avocados. The fungus infection triggers a rapid onset of laurel wilt disease, which has nearly eliminated laurel trees across the southeastern United States within a few years. The beetle has spread across nearly 300 counties in the Southeast, facilitated by trade in wood products. Effective control in natural environments remains an unresolved challenge; in avocado groves, infected trees must be removed and destroyed. Introduction of X. glabratus into Mexico, California, Central America, or South America, regions with substantial avocado industries and diverse native Lauraceae species, would be disastrous. International cooperation is necessary to address this threat.
Introduction
Approximately 3,000 species of ambrosia beetles have been identified. Most of these are inconspicuous forest inhabitants with minimal impact on human activities. However, the redbay ambrosia beetle, Xyleborus glabratus Eichhoff (Coleoptera: Curculionidae), stands as a notable exception due to its role as a vector of a pathogenic fungus. This beetle primarily inhabits the xylem of trees in the laurel family (Lauraceae). The beetle and its progeny feed on its symbiotic fungus, Harringtonia lauricola (previously known as Raffaelea lauricola, Ophiostomatales: Ophiostomataceae). The beetle-fungus pair is inconspicuous and non-threatening in their native Asian forest region, where the beetle colonizes only stressed or dying Lauraceae, and the fungus does not cause a systemic response in the native host species (Hulcr et al. 2017). However, the fungus is pathogenic to American laurels, and its introduction to the United States triggered the fastest recorded epidemic of tree deaths (Fraedrich et al. 2015). After landscape-wide establishment, H. lauricola was found to also occur with other ambrosia beetles, especially in the congeneric Xyleborus ferrugineus (Fabricius), Xyleborus bispinatus Eichhoff, and Xyleborus affinis Eichhoff (Carrillo et al. 2014, Ploetz et al. 2017a). The expansion of X. glabratus and H. lauricola in the United States has led to over 300 million native Lauracae trees being infected and dying since its introduction (Hughes et al. 2017a, Joseph et al. 2023). This raises additional concern about potential spread of the disease to Central and South America and its impact on the avocado industry and laurels in the natural vegetation.
Recent History
X. glabratus was first intercepted in United States at a port in Georgia in 2002 (Haack 2003). Initial signs of significant mortality among redbay trees (Persea borbonia) were noted a year later, though the destructive potential was not immediately apparent (Douce and Johnson 2005, Mayfield and Thomas 2006). By 2005, a clear correlation between X. glabratus and redbay tree mortality was observed (Mayfield and Thomas 2006) and the beetle was given the common name “redbay ambrosia beetle.” In 2008, it was confirmed that the mortality of redbay and other Lauraceae species was due to the laurel wilt pathogen, then named Raffaelea lauricola, carried in the mycangia [a specialized morphological structure of ambrosia beetles used to transport fungal symbionts (Francke-Grosmann 1967)] of X. glabratus (Fraedrich et al. 2008, Harrington et al. 2008). A recent taxonomic study reclassified Raffaelea lauricola as Harringtonia lauricola (De Beer et al. 2022).
Biology and Life Cycle
X. glabratus belongs to the weevil subfamily of bark and ambrosia beetles (Curculionidae: Scolytinae), tribe Xyleborini. As in all members of the tribe, its biology is intertwined with a symbiotic wood-colonizing fungus. The beetle life cycle comprises four stages: egg, larva, pupa, and adult. Larvae feed exclusively on the specific symbiotic fungus. Females initiate egg-laying approximately a week after colonizing sapwood, with eggs taking two to three weeks to hatch into larvae. Larvae can develop into pupae after one week, and newly emerged adults can emerge from pupae in approximately one week (Brar 2012; Brar et al. 2013). While X. glabratus can complete its entire life cycle in one month in optimal lab conditions, development usually takes longer in natural settings (Brar et al. 2013; Brar et al. 2015). X. glabratus could not be reared for more than one generation on avocado logs, and its reproduction was significantly reduced compared with the multigenerational colonies obtained on swampbay logs (Brar et al. 2013).
Most ambrosia fungi are not wood decay fungi. They are unable to digest the structural components of wood, such as cellulose or lignin, and H. lauricola is not an exception. Instead, ambrosia fungi require inoculation into fresh wood, where they grow rapidly through the vascular tissue and sequester easily digestible nutrients (Huang et al. 2019; Skelton et al. 2019).
Like all Xyleborini, X. glabratus exhibits a distinctive reproductive strategy: haplodiploidy combined with inbreeding. Females typically mate with a male from their natal gallery (typically their brother). They then disperse and establish their own galleries, in which they lay fertilized diploid eggs, resulting in female offspring. The female also lays one or a few unfertilized eggs, from which haploid males hatch. This haplo-diploid sibling mating capacity increases the chance for these bark and ambrosia beetles to establish populations in new environments (Jordal et al. 2001).
Redbay ambrosia beetle X. glabratus appears to be a weak flyer, at least in laboratory experiments. They disperse by short flights of 1 to 5 m usually adding up to approximately 20 m distance in 24h (only a few fly more than 50 m, and none more than 200 m) with the highest activity between 3:00 to 9:00 PM (Seo et al. 2017).
Description
Adult X. glabratus females are 2.2–2.5 mm long, only about half the length of a grain of rice (Cognato et al. 2019), and smaller than most American Xyleborus species. Mature females are dark brown to black (newly emerged immature females are lighter), notably shiny, and nearly devoid of hair or setae. The lack of hair inspired their taxonomic name “glabratus,” which is derived from “glabrous,” meaning hairless.
The elytral ends meet at a point, rather than being rounded as in most other Xyleborus species; and the elytral slope bears several tiny, tooth-like structures (denticles) (Cognato et al. 2019).
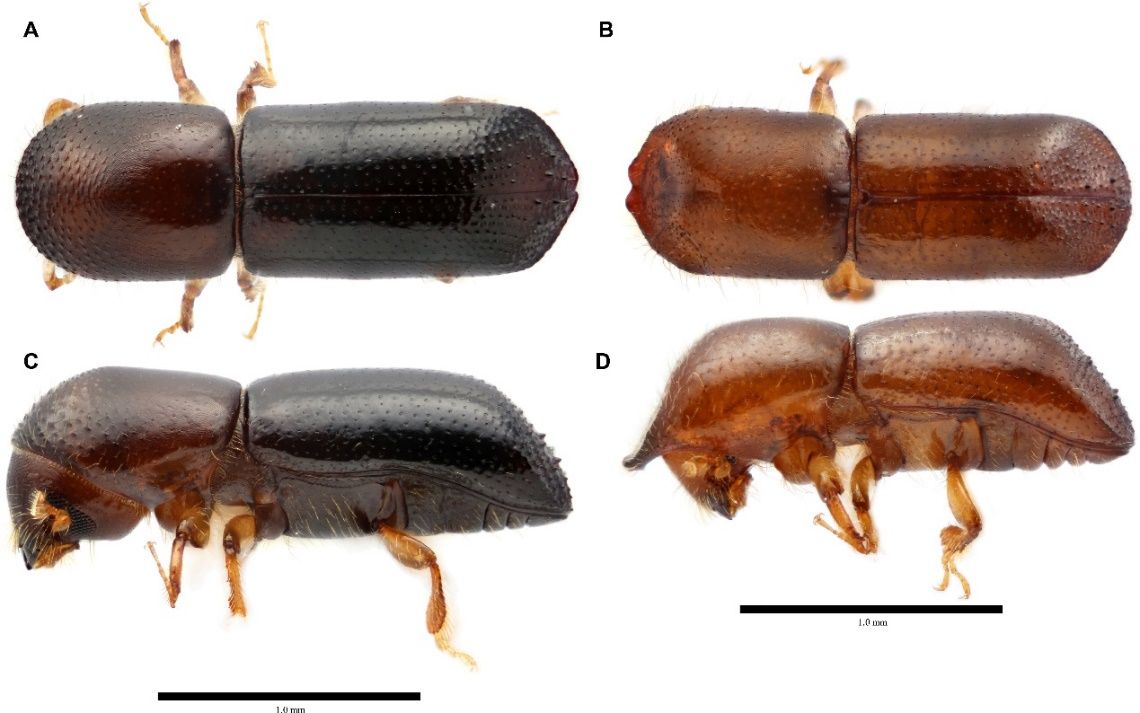
Credit: Andrew J. Johnson, UF/IFAS
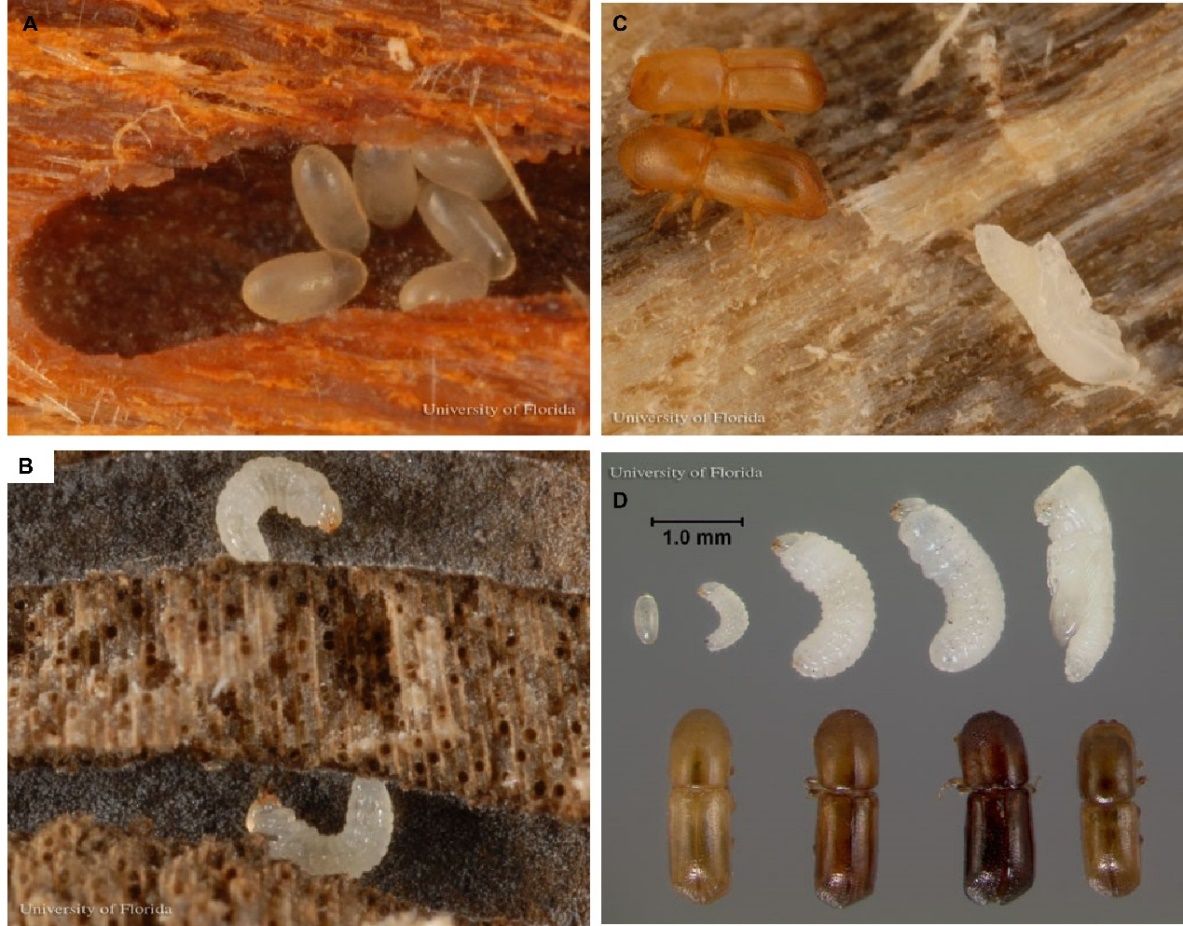
Credit: Lyle J. Buss, UF/IFAS
Tree Host Colonization
X. glabratus appears to colonize living American Lauraceae due to a semiochemical mismatch. The pioneer female is attracted to healthy leaf volatiles from bay trees but cannot establish galleries in them, typically dying instead. However, the fungus inoculum persists. As it grows within the tree’s vascular tissue, it causes excessive formation of defensive tyloses. The tree's rapid wilt and decline produces stress-related chemicals (such as methyl salicylate), initially repelling X. glabratus (Martini et al. 2017). After the tree’s desiccation and death, however, the wood releases sesquiterpenes, which attracts many more X. glabratus (Martini et al. 2017; Conover et al. 2024).
Host Plants
X. glabratus primarily targets plants in Lauraceae family, including redbay (Persea borbonia), swampbay (Persea palustris), and sassafras (Sassafras albidium), particularly in the United States (Hanula et al. 2008; Kendra et al. 2014; Crane et al. 2015). The rarity of this beetle in the avocado growing region contrasts with its abundance in natural areas, suggesting that avocado is a suboptimal host for X. glabratus, alhough several cases of X. glabratus colonization and reproduction on avocados have been reported (Mayfield et al. 2008a; Brar et al. 2013; Carrillo et al. 2012). In its native regions in Asia, the beetle also primarily colonizes Lauraceae (Hulcr and Lou 2013), and although several aberrant records from different families have been published, they may not be reproductive hosts (Cognato et al. 2019).
Damage and Symptoms
The impact of X. glabratus and its associated pathogen fungus, H. lauricola, on trees is characterized by distinct signs and symptoms, described together as laurel wilt. The most obvious symptoms are wilting and discoloration of foliage, which over the course of a few weeks gradually advance to affect twigs, branches, and ultimately the entire tree (Fraedrich et al. 2015). The initial invasion by X. glabratus can be challenging to see because the initial circular entrance holes on the tree bark are very small, and very few. After additional females successfully enter the xylem, they extrude frass stacks often called sawdust noodles. Sawdust noodles are a common sign of ambrosia beetle activity. They are not exclusive to X. glabratus and not reliable for diagnosis. A more definitive symptom of X. glabratus infestation and H. lauricola infection is the appearance of dark staining in the sapwood (Figure 3) (Fraedrich et al. 2015). This staining directly results from the fungal infection and serves as a key diagnostic feature in identifying affected trees.
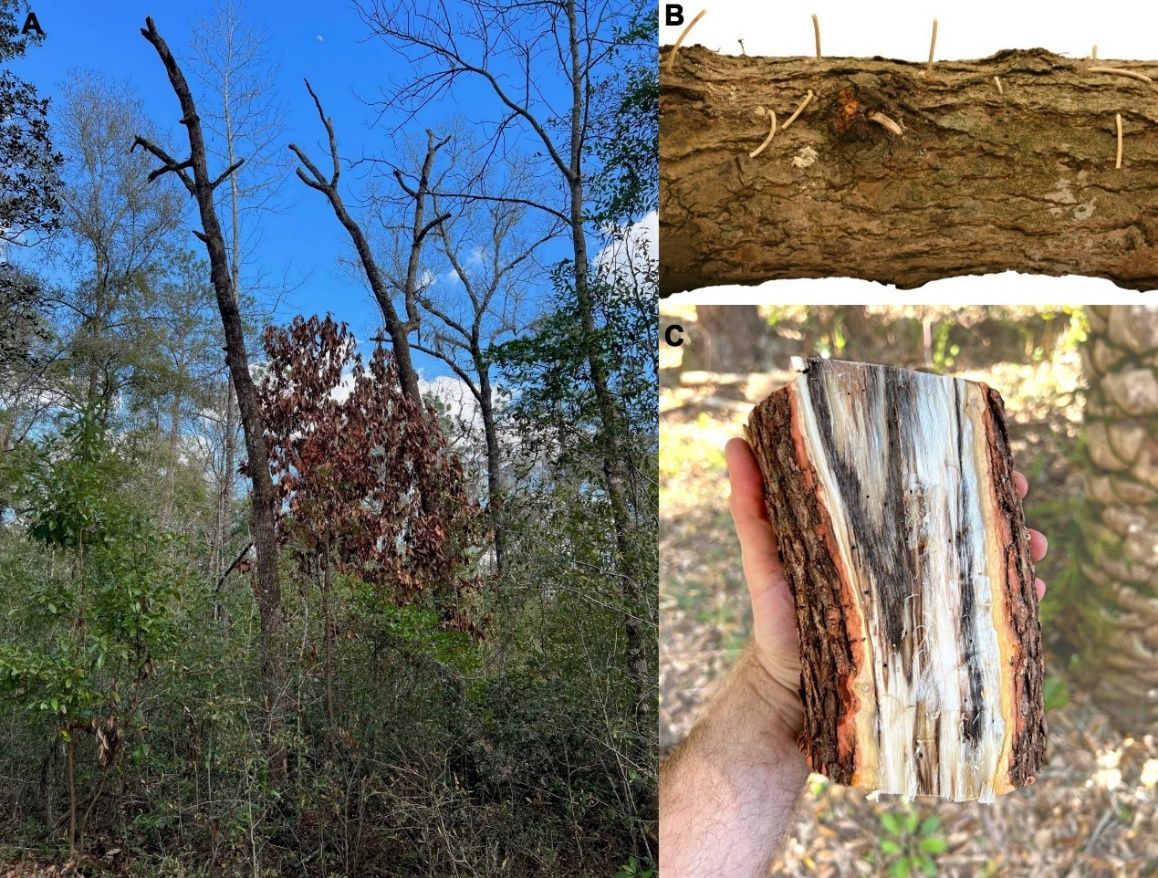
Credit: Jiri Hulcr, UF/IFAS
The progression of laurel wilt following H. lauricola infection involves a series of physiological processes. When the fungus infects the tree, it triggers the formation of tyloses: outgrowths in the tree's vessel elements of xylem (vascular system). These tyloses effectively block water transport within the tree, leading to the rapid cessation of water flow (Inch and Ploetz 2012; Inch et al. 2012; Fraedrich et al. 2015; Martini et al. 2020). As a result, the affected tree wilts and succumbs to the disease in a few weeks.
Several other damaging agents may be confused with laurel wilt but are not contagious to other trees.
First, the black twig borer Xylosandrus compactus (Eichhoff) (Coleoptera: Curculionidae) colonizes small twigs and stems of many tree species, including wild laurels and avocados (Dixon et al. 2003). The beetle boring kills the twig, and the leaf browning and wilting may resemble laurel wilt (Hughes et al. 2015). The black twig borer only colonizes individual twigs, however, and therefore the leaf flagging it creates is scattered. It does not colonize the trunk and does not kill larger trees. Further, the vascular discoloration of twigs colonized by X. compactus is localized around the entrance hole and the gallery (Dixon et al. 2003; Hughes et al. 2015).
Phytophthora root rot may result in the death of a whole avocado tree, and external symptoms may resemble those of laurel wilt (Crane et al. 2020; Navia-Urrutia et al. 2022). Phytophthora is a soil-borne, fungus-like organism. The disease progression of phytophthora root rot is generally slower than that of laurel wilt. Leaf discoloration progresses from green to yellow, and the trees typically defoliate (Coffey 1987; Salgadoe et al. 2018). Avocado death may be quicker if phytophthora infection is combined with flooding (Ploetz and Schaffer 1987; Schaffer et al. 1992). It does not cause the staining in the wood that is characteristic of laurel wilt. Examining the roots can aid in diagnosing phytophthora root rot.
Avocado at the northern edge of its cold tolerance (for example, in north Florida) often suffers from frost damage, which may look superficially similar to laurel wilt. In addition, frost damage in the spring often attracts ambrosia beetles that create sawdust noodles, further adding to potential confusion. The easiest way to distinguish laurel wilt from frost damage is to identify the ambrosia beetles involved and to observe weather patterns in the period immediately preceding the damage, given that frost damage only occurs after an acute frost episode.
In summary, while the initial signs of X. glabratus activity, such as entrance holes and sawdust noodles, may resemble those of other ambrosia beetles, infestation by X. glabratus and H. lauricola can be diagnosed by 1) morphological characteristics of the beetle, 2) dark sapwood staining, 3) the rapid progression of wilt, with leaves staying attached, and 4) the host identity as Lauraceae.
Geographic Distribution
Originally native to China and Japan, X. glabratus has been detected in various southeastern US regions since its first appearance in Georgia in 2002 (Haack 2003). Its presence has been confirmed in nearly 300 counties across the United States, ranging from western Texas to eastern North Carolina, south to Miami-Dade in Florida, and north to Kentucky (Laurel Wilt Public Dashboard 2023). The rapid and patchy expansion was clearly facilitated by movement of infested wood products across the region (Haack and Rabaglia 2013; Crane et al. 2020).
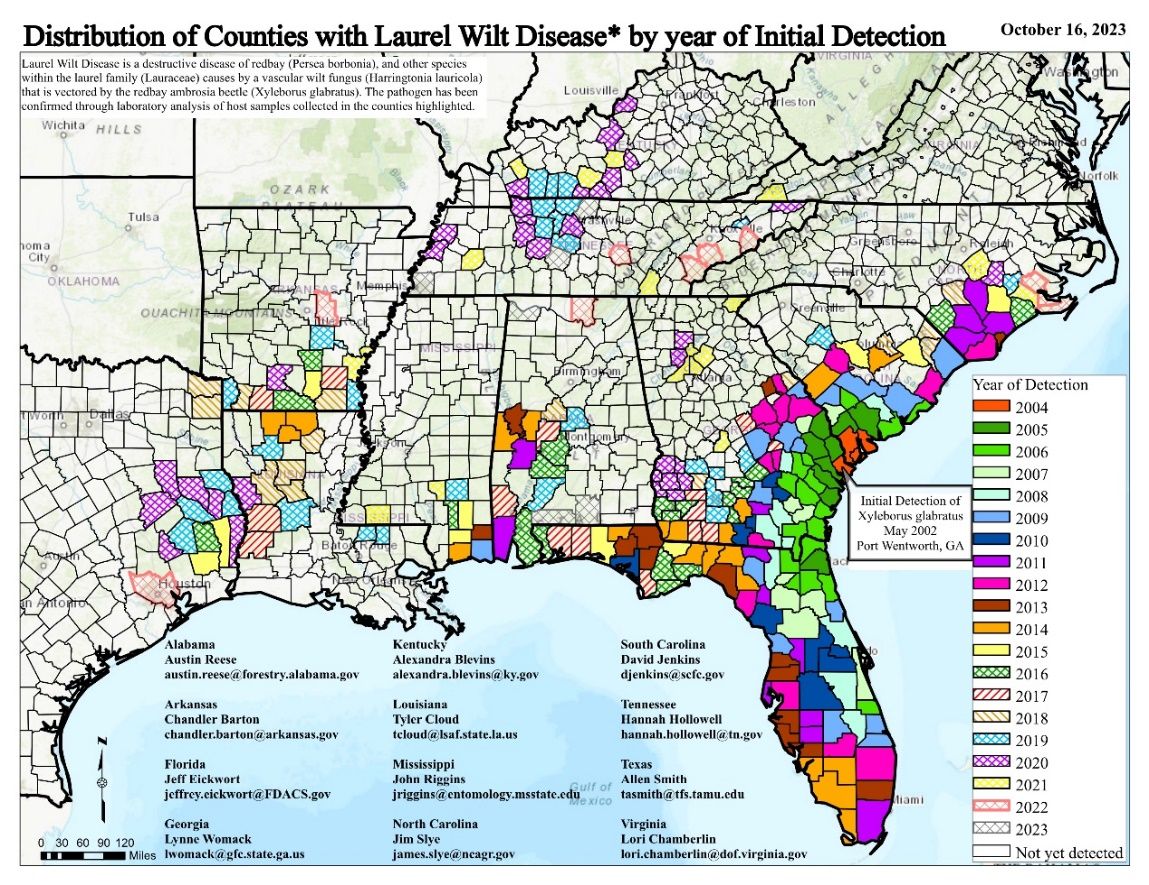
Credit: USDA Forest Service
Chemical Ecology
The set of chemicals that the redbay ambrosia beetle uses to find and evaluate its host is highly specific to its lauraceous hosts. Such specificity is relatively unusual among ambrosia beetles. X. glabratus is most attracted to sesquiterpenes (alpha-copaene, beta-caryophyllene, and alpha-humulene) commonly found in lauraceous wood, and to monoterpenes emitted by healthy trees (Brar et al. 2015; Martini et al. 2015). The beetle is repelled by plant hormones such as methyl salicylate that indicate a recent infection by the fungus (when the tree is actively defending itself) but attracted to the subsequent stages of the infection (when the tree is still fresh, but safely dead) (Martini et al. 2017).
Many ambrosia beetle species are known to be attracted to ethanol (Ranger et al. 2018). X. glabratus appears to be indifferent to ethanol, or even mildly repelled by it (Hanula et al. 2011; Kendra et al. 2014).
Monitoring
The redbay ambrosia beetle can be captured on sticky traps and in funnel traps or simple bottle traps baited with a lure containing alpha-copaene. The first attractive lure used was manuka oil, an essential oil that is rich in alpha-copaene (Hanula 2013). More recently, lures with a concentrated amount of alpha copaene have been developed and are used for ambrosia beetle monitoring (Kendra et al. 2016).
Note that the beetle can easily be present in the landscape below the detection limit of traps. Therefore, the most effective approach for detecting the presence of laurel wilt is to monitor areas for bay trees with laurel wilt symptoms.
Management in Natural Areas
Effective management of X. glabratus differs depending on the environment, with different strategies for natural areas versus avocado groves.
In natural environments, practical active management methods remain elusive. Some redbay tree individuals show innate tolerance (Hughes et al. 2015). Some have been successfully propagated and the progeny remains tolerant, but they are not commercially available. Preventative treatment of high-value redbay trees is possible (Mayfield et al. 2008b). A redbay on the University of Florida’s Gainesville campus that was experimentally injected with propiconazole every two years has survived until today, while all the other redbays in its vicinity have long since died.
Repellants may also be an option. Verbenone, a non-host volatile originally from conifers, is already used in the management of some bark beetles. In a pouch directly stapled to the trees, or in a paste directly applied to the trunk (e.g., SPLAT Verb®), it provides protection against the redbay ambrosia beetle and increases survivorship of redbay in areas where laurel wilt is prevalent (Hughes et al. 2017; Martini et al. 2020a; Martini et al. 2020b). Given the price of this method, it is likely to be used only on high-value individual trees.
Management in Avocado Groves
A comprehensive summary of laurel wilt management in commercial avocado is available here: https://edis.ifas.ufl.edu/publication/HS1360. Here we provide a brief summary.
X. glabratus does not typically attack avocado as its primary host, and reports of its presence in South Florida avocado groves are rare. However, avocado trees are susceptible to the fungus, which can be vectored by other ambrosia beetles. Therefore, management of the disease in groves does not target X. glabratus but rather other ambrosia beetles frequently carrying H. lauricola. Laurel wilt in south Florida avocado groves initially spread from foci with heavy infestations, typically abandoned groves. Now the pathogen is endemic, with new infestations occurring randomly. However, due to root grafting among mature avocado trees, once a new infestation occurs it rapidly spreads to adjacent trees (Ploetz et al. 2017b; Ploetz et al. 2017c).
The most practical active management strategy to reduce ambrosia beetle density and activity in groves is to prune mature trees to increase light exposure and air movement within the tree and the grove (Menocal et al. 2022). An additional strategy is sanitation, in which groves are monitored for wilting trees, and affected trees are rapidly removed. Once a tree is infested by the fungus, complete removal, including as much root system as possible, is the only recourse, as there is no cure. Shredding of the complete tree including the stump, or its removal from the grove, is essential to prevent future beetle breeding.
Various chemical, physical, and biological strategies have been attempted but have demonstrated limited practical utility. Certain chemical agents can inhibit the growth of the pathogenic symbiotic fungus H. lauricola (Mayfield et al. 2008b; Peña et al. 2011; Hughes et al. 2017b; Martini et al. 2017; Navia-Urrutia et al. 2023), but industrial-scale prevention remains lacking. Insecticides, including zeta-cypermethrin, bifenthrin, lambda-cyhalothrin, thiamethoxam, and fenpropathrin, have limited effectiveness. Aerial sprays are ineffective once ambrosia beetles are established inside trees. Combining multiple pesticides, for instance malathion, zeta-cypermethrin, and bifenthrin, has shown higher efficacy in protecting trees against X. glabratus attacks, but this practice has not been adopted because of the rarity of X. glabratus in avocado orchards.
In avocado groves, a mixture of methyl salicylate and verbenone has been shown to repel various ambrosia beetles from individual avocado trees, including beetle species that may vector H. lauricola (Martini, unpublished data; Byers et al. 2021), but this chemistry is not yet available as a commercial product.
Future Outlook
The most significant threat posed by X. glabratus is the potential that it may be introduced to California, Mexico, Central America, and South America, a scenario that could be disastrous. Meanwhile, the beetle and its associated fungus, H. lauricola, continue to expand their range in North America, exterminating populations of native Lauraceae. The spread is further facilitated by trade and the movement of infested wood products. The cryptic nature of the beetle, the fungus’s moderate promiscuity, and the potential for lateral transfer of the pathogen to other ambrosia beetle species all allow this destructive fungus to spread readily in (Carrillo et al. 2014; Haack and Rabaglia 2013; Crane et al. 2020). To mitigate this risk, wood products from regions with X. glabratus and other ambrosia beetles contaminated with the laurel wilt pathogen should undergo chipping, heat treatment, or chemical treatments before transport. Untreated wood products from regions where X. glabratus has been found should not be transported. Most crucially, they must never be shipped across the country or internationally (Mayfield and Thomas 2006; IPSM15 2019).
References
Brar, G. S. 2012. Ecology and biology of redbay ambrosia beetle (Xyleborus glabratus Eichhoff). PhD dissertation, University of Florida. https://ufdc.ufl.edu/ufe0044906/00001
Brar, G. S., Capinera, J. L., Kendra, P. E., McLean, S., and Peña, J. E. 2013. Life cycle, development, and culture of Xyleborus glabratus (Coleoptera: Curculionidae: Scolytinae). Florida Entomologist 96:1158–1167. https://doi.org/10.1653/024.096.0357
Brar, G. S., Capinera, J. L., Kendra, P. E., Smith, J. A., and Peña, J. E. 2015. Temperature-dependent development of Xyleborus glabratus (Coleoptera: Curculionidae: Scolytinae). Florida Entomologist:856–864. https://doi.org/10.1653/024.098.0307
Carrillo, D., Duncan, R. E., Ploetz, J. N., Campbell, A. F., Ploetz, R. C., and Peña, J. E. 2014. Lateral transfer of a phytopathogeneic symbiont among native and exotic ambrosia beetles. Plant Pathology 63:54–62. https://doi.org/10.1111/ppa.12073
Coffey, M. D. 1987. Phytophthora root rot of avocado: an integrated approach to control in California. Plant Disease 71:1046–1053. http://avocadosource.com/CAS_Yearbooks/CAS_71_1987/CAS_1987_PG_121-137.pdf
Cognato, A. I., Smith, S. M., Li, Y., Pham, T. H., and Hulcr, J. 2019. Genetic variability among Xyleborus glabratus populations native to Southeast Asia (Coleoptera: Curculionidae: Scolytinae: Xyleborini) and the description of two related species. Journal of Economic Entomology 112:1274–1284. https://doi.org/10.1093/jee/toz026
Conover, D., Paris, T., and Martini, X. 2024. Ecological dynamics of ambrosia beetle species in laurel wilt infected trees. Biological Invasions 26: 583–590. https://doi.org/10.1007/s10530-023-03194-1
Crane, J. H., Evans, E., Carrillo, D., Ploetz, R., and Palmateer, A. 2015. The potential for laurel wilt to threaten avocado production is real. Pages 9–16 in ACTAS: Proc. VIII Congreso Mundial de la Palta, Lima, Perú. Avocadosource. com, Hofshi Foundation, Fallbrook, CA. https://www.avocadosource.com/WAC8/Section_01/CraneJonathan2015b.pdf
Crane, J. H., Gazis, R., Wasielewski, J., Carrillo, D., Schaffer, B., Ballen, F., and Evans, E. A. 2020. Sampling guidelines and recommendations for submitting samples for diagnosing laurel wilt in avocado trees (Persea americana L.). EDIS, 2020. https://doi.org/10.32473/edis-hs1394-2020
Crane, J. H., Wasielewski, J., Carrillo, D., Gazis, R., Schaffer, B., Ballen, F., and Evans, E. 2020. Recommendations for the Detection and Mitigation of Laurel Wilt Disease in Avocado and Related Tree Species in the Home Landscape1. Horticultural Sciences Department, Universitty of Florida, IFAS Extension. https://doi.org/10.32473/edis-hs1358-2020
De Beer, Z. W., Procter, M., Wingfield, M. J., Marincowitz, S., and Duong, T. A. 2022. Generic boundaries in the Ophiostomatales reconsidered and revised. Studies in Mycology 101:57–120. https://doi.org/10.3114/sim.2022.101.02
Dixon, W., Woodruff, R., and Foltz, J. 2003. Black twig borer, Xylosandrus compactus (Eichhoff)(Insecta: Coleoptera: Curculionidae: Scolytinae). https://edis.ifas.ufl.edu/in577
Douce, G., and Johnson, J. 2005. Xyleborus glabratus in Georgia’s costal forests. Georgia Forestry Commission Pest Alert, October 31:2005.
Fraedrich, S., Harrington, T., and Best, G. 2015. Xyleborus glabratus attacks and systemic colonization by Raffaelea lauricola associated with dieback of Cinnamomum camphora in the southeastern United States. Forest pathology 45:60–70. https://doi.org/10.1111/efp.12124
Fraedrich, S., Harrington, T., Rabaglia, R., Ulyshen, M., Mayfield Iii, A., Hanula, J., Eickwort, J., and Miller, D. 2008. A fungal symbiont of the redbay ambrosia beetle causes a lethal wilt in redbay and other Lauraceae in the southeastern United States. Plant Disease 92:215–224. https://doi.org/10.1094/PDIS-92-2-0215
Francke-Grosmann, H. 1967. Ectosymbiosis in wood-inhabiting insects. Symbiosis:141–205. https://doi.org/10.1016/B978-1-4832-2758-0.50010-2
Haack, R. A. 2003. Intercepted Scolytidae (Coleoptera) at US ports of entry: 1985–2000. Integrated Pest Management Reviews 6:253–282. https://doi.org/10.1023/A:1025715200538
Haack, R. A., and Rabaglia, R. J. 2013. Exotic bark and ambrosia beetles in the USA: potential and current invaders. Pages 48–74 Potential invasive pests of agricultural crops. CABI Wallingford UK. https://doi.org/10.1079/9781845938291.0048
Hanula, J. L., Mayfield III, A. E., Fraedrich, S. W., and Rabaglia, R. J. 2008. Biology and host associations of redbay ambrosia beetle (Coleoptera: Curculionidae: Scolytinae), exotic vector of laurel wilt killing redbay trees in the southeastern United States. Journal of Economic Entomology 101:1276–1286. https://doi.org/10.1093/jee/101.4.1276
Hanula, J. L., Ulyshen, M. D., and Horn, S. 2011. Effect of trap type, trap position, time of year, and beetle density on captures of the redbay ambrosia beetle (Coleoptera: Curculionidae: Scolytinae). Journal of Economic Entomology 104:501–508. https://doi.org/10.1603/EC10263
Harrington, T., Fraedrich, S., and Aghayeva, D. 2008. Raffaelea lauricola, a new ambrosia beetle symbiont and pathogen on the Lauraceae. Mycotaxon 104:399–404.
Huang, Y. T., Skelton, J., and Hulcr, J. 2019. Multiple evolutionary origins lead to diversity in the metabolic profiles of ambrosia fungi. Fungal Ecology 38:80–88. https://doi.org/10.1016/j.funeco.2018.03.006
Hughes, M., Riggins, J., Koch, F., Cognato, A., Anderson, C., Formby, J., Dreaden, T., Ploetz, R., and Smith, J. 2017a. No rest for the laurels: symbiotic invaders cause unprecedented damage to southern USA forests. Biological Invasions 19:2143–2157. https://doi.org/10.1007/s10530-017-1427-z
Hughes, M., Smith, J., Ploetz, R., Kendra, P., Mayfield, A., Hanula, J., Hulcr, J., Stelinski, L., Cameron, S., and Riggins, J. 2015. Recovery plan for laurel wilt on redbay and other forest species caused by Raffaelea lauricola and disseminated by Xyleborus glabratus. Plant Health Progress 16:173–210. https://doi.org/10.1094/PHP-RP-15-0017
Hughes, M. A., Martini, X., Kuhns, E., Colee, J., Mafra‐Neto, A., Stelinski, L., and Smith, J. 2017b. Evaluation of repellents for the redbay ambrosia beetle, Xyleborus glabratus, vector of the laurel wilt pathogen. Journal of Applied Entomology 141:653–664. https://doi.org/10.1111/jen.12387
Hulcr, J., Black, A., Prior, K., Chen, C.-Y., and Li, H.-F. 2017. Studies of Ambrosia Beetles (Coleoptera: Curculionidae) in Their Native Ranges Help Predict Invasion Impact. Florida Entomologist 100:257–261, 255. https://doi.org/10.1653/024.100.0219
Hulcr, J., and Lou, Q. Z. 2013. The redbay ambrosia beetle (Coleoptera: Curculionidae) prefers Lauraceae in its native range: records from the Chinese National Insect Collection. Florida Entomologist 96:1595–1596. https://doi.org/10.1653/024.096.0444
Inch, S., and Ploetz, R. 2012. Impact of laurel wilt, caused by Raffaelea lauricola, on xylem function in avocado, Persea americana. Forest pathology 42:239–245. https://doi.org/10.1111/j.1439-0329.2011.00749.x
Inch, S., Ploetz, R., Held, B., and Blanchette, R. 2012. Histological and anatomical responses in avocado, Persea americana, induced by the vascular wilt pathogen, Raffaelea lauricola. Botany 90:627–635. https://doi.org/10.1139/b2012-015
IPSM15. 2019. International Standards for Phytosanitary Measures No.15: Regulation of wood packaging material in international trade.
Jordal, B. H., Beaver, R. A., and Kirkendall, L. R. 2001. Breaking taboos in the tropics: incest promotes colonization by wood‐boring beetles. Global Ecology and Biogeography 10:345–357. https://doi.org/10.1046/j.1466-822X.2001.00242.x
Joseph, R., Bansal, K., and Keyhani, N. O. 2023. Host switching by an ambrosia beetle fungal mutualist: Mycangial colonization of indigenous beetles by the invasive laurel wilt fungal pathogen. Environmental Microbiology. https://doi.org/10.1111/1462-2920.16401
Kendra, P. E., Montgomery, W. S., Niogret, J., Pruett, G. E., Mayfield III, A. E., MacKenzie, M., Deyrup, M. A., Bauchan, G. R., Ploetz, R. C., and Epsky, N. D. 2014. North American Lauraceae: Terpenoid emissions, relative attraction and boring preferences of redbay ambrosia beetle, Xyleborus glabratus (Coleoptera: Curculionidae: Scolytinae). PLoS ONE 9:e102086. https://doi.org/10.1371/journal.pone.0102086
Laurel Wilt Public Dashboard at ArcGIS Online, accessed on November 2, 2023: https://www.arcgis.com/apps/dashboards/d43391c8fdb741b597e6ccf1236d2a02
Martini, X., Hughes, M. A., Killiny, N., George, J., Lapointe, S. L., Smith, J. A., and Stelinski, L. L. 2017. The fungus Raffaelea lauricola modifies behavior of its symbiont and vector, the redbay ambrosia beetle (Xyleborus glabratus), by altering host plant volatile production. J Chem Ecol 43:519–531. https://doi.org/10.1007/s10886-017-0843-y
Martini, X., Hughes, M. A., Smith, J. A., and Stelinski, L. L. 2015. Attraction of redbay ambrosia beetle, Xyleborus glabratus, to leaf volatiles of its host plants in North America. J Chem Ecol 41:613–621. https://doi.org/10.1007/s10886-015-0595-5
Martini, X., Sobel, L., Conover, D., Mafra‐Neto, A., and Smith, J. 2020. Verbenone reduces landing of the redbay ambrosia beetle, vector of the laurel wilt pathogen, on live standing redbay trees. Agricultural and Forest Entomology 22:83–91. https://doi.org/10.1111/afe.12364
Mayfield III, A. E., Barnard, E. L., Smith, J. A., Bernick, S. C., Eickwort, J. M., and Dreaden, T. J. 2008b. Effect of propiconazole on laurel wilt disease development in redbay trees and on the pathogen in vitro. Arboric. Urban For 34:317–324. https://doi.org/10.48044/jauf.2008.043
Mayfield, A. E., Peña, J. E., Crane, J. H., Smith, J. A., Branch, C. L., Ottoson, E. D., and Hughes, M. 2008a. Ability of the redbay ambrosia beetle (Coleoptera: Curculionidae: Scolytinae) to bore into young avocado (Lauraceae) plants and transmit the laurel wilt pathogen (Raffaelea sp.). The Florida Entomologist 91:485–487. https://doi.org/10.1653/0015-4040(2008)91[485:AOTRAB]2.0.CO;2
Mayfield III, A. E., and Thomas, M. C. 2006. The redbay ambrosia beetle, Xyleborus glabratus Eichhoff (Scolytinae: Curculionidae). Pest Alert. Florida Department of Agriculture and Consumer Services.
Menocal, O., Kendra, P. E., Padilla, A., Chagas, P. C., Chagas, E. A., Crane, J. H., and Carrillo, D. 2022. Influence of canopy cover and meteorological factors on the abundance of bark and ambrosia beetles (Coleoptera: Curculionidae) in avocado orchards affected by laurel wilt. Agronomy 12, 547. https://doi.org/10.3390/agronomy12030547
Navia-Urrutia, M., Sánchez-Pinzón, L., Parra, P. P., and Gazis, R. 2022. A diagnostic guide for laurel wilt disease in avocado. Plant Health Progress 23:345–354. https://doi.org/10.1094/PHP-12-21-0149-DG
Navia-Urrutia, M., Sendoya-Corrales, C. A., Crane, J. H., and Gaizs, R. 2023. Reevaluation of the application method and efficacy of propiconazole in controlling laurel wilt in avocado orchards in south Florida. HortTechnology 33:425–436. https://doi.org/10.21273/HORTTECH05232-23
Peña, J. E., Crane, J. H., Capinera, J. L., Duncan, R. E., Kendra, P. E., Ploetz, R. C., McLean, S., Brar, G., Thomas, M. C., and Cave, R. D. 2011. Chemical control of the redbay ambrosia beetle, Xyleborus glabratus, and other Scolytinae (Coleoptera: Curculionidae). Florida Entomologist 94:882–896. https://doi.org/10.1653/024.094.0424
Ploetz, R. C., Konkol, J. L., Narvaez, T., Duncan, R. E., Saucedo, R. J., Campbell, A., Mantilla, J., Carrillo, D., and Kendra, P. E. 2017a. Presence and prevalence of Raffaelea lauricola, cause of laurel wilt, in different species of ambrosia beetle in Florida, USA. Journal of Economic Entomology 110:347–354. https://doi.org/10.1093/jee/tow292
Ploetz, R.C., Kendra, P. E., Choudhury, R. A., Rollins, J. A., Campbell, A., Garrett, K., Hughes, M., and Dreaden, T. 2017b. Laurel wilt in natural and agricultural ecosystems: understanding the drivers and scales of complex pathosystems. Forests 8:48. https://doi.org/10.3390/f8020048
Ploetz, R. C., Konkol, J. L., Pérez-Martínez, J. M., and Fernandez, R. 2017c. Management of laurel wilt of avocado, caused by Raffaelea lauricola. European Journal of Plant Pathology 149:133–143. https://doi.org/10.1007/s10658-017-1173-1
Ploetz, R. C., and Schaffer, B. 1987. Effects of flooding and Phytophthora root rot on photosynthetic characteristics of avocado. In: Proceedings of the Florida State Horticultural Society 100:290–294.
Ranger, C. M., Biedermann, P. H., Phuntumart, V., Beligala, G. U., Ghosh, S., Palmquist, D. E., Mueller, R., Barnett, J., Schultz, P. B., and Reding, M. E. 2018. Symbiont selection via alcohol benefits fungus farming by ambrosia beetles. Proceedings of the National Academy of Sciences 115:4447–4452. https://doi.org/10.1073/pnas.1716852115
Salgadoe, A. S. A., Robson, A. J., Lamb, D. W., Dann, E. K., and Searle, C. 2018. Quantifying the severity of phytophthora root rot disease in avocado trees using image analysis. Remote Sensing 10(2):226. https://doi.org/10.3390/rs10020226
Schaffer, B., Andersen, P. C., and Ploetz, R. C. 1992. “Responses of fruit crops to flooding” in Horticultural Reviews. John Wiley and Sons, New York. (p.257–314) https://doi.org/10.1002/9780470650509.ch7
Seo, M., Martini, X., Rivera, M.J. and Stelinski, L.L., 2017. Flight capacities and diurnal flight patterns of the ambrosia beetles, Xyleborus glabratus and Monarthrum mali (Coleoptera: Curculionidae). Environmental Entomology, 46(3): 729–734. https://doi.org/10.1093/ee/nvx085
Skelton, J., Jusino, M. A., Carlson, P. S., Smith, K., Banik, M. T., Lindner, D. L., Palmer, J. M., and Hulcr, J. 2019. Relationships among wood‐boring beetles, fungi, and the decomposition of forest biomass. Molecular Ecology 28:4971–4986. https://doi.org/10.1111/mec.15263