This document explains the details of equipment cleaning and sanitizing procedures in food-processing and/or food-handling operations.
Background
Cleaning and Sanitizing Program
Since cleaning and sanitizing may be the most important aspects of a sanitation program, sufficient time should be given to outline proper procedures and parameters. Detailed procedures must be developed for all food-product contact surfaces (equipment, utensils, etc.) as well as for non-product surfaces such as non-product portions of equipment, overhead structures, shields, walls, ceilings, lighting devices, refrigeration units and heating, ventilation and air conditioning (HVAC) systems, and anything else which could impact food safety.
Cleaning frequency must be clearly defined for each process line (i.e., daily, after production runs, or more often if necessary). The type of cleaning required must also be identified.
The objective of cleaning and sanitizing food contact surfaces is to remove food (nutrients) that bacteria need to grow, and to kill those bacteria that are present. It is important that the clean, sanitized equipment and surfaces drain dry and are stored dry so as to prevent bacteria growth. Necessary equipment (brushes, etc.) must also be clean and stored in a clean, sanitary manner.
Cleaning/sanitizing procedures must be evaluated for adequacy through evaluation and inspection procedures. Adherence to prescribed written procedures (inspection, swab testing, direct observation of personnel) should be continuously monitored, and records maintained to evaluate long-term compliance.
The correct order of events for cleaning/sanitizing of food product contact surfaces is as follows:
- Rinse
- Clean
- Rinse
- Sanitize.
Definitions
Cleaning
Cleaning is the complete removal of food soil using appropriate detergent chemicals under recommended conditions. It is important that personnel involved have a working understanding of the nature of the different types of food soil and the chemistry of its removal.
Cleaning Methods
Equipment can be categorized with regard to cleaning method as follows:
- Mechanical Cleaning. Often referred to as clean-in-place (CIP). Requires no disassembly or partial disassembly.
- Clean-out-of-Place (COP). Can be partially disassembled and cleaned in specialized COP pressure tanks.
- Manual Cleaning. Requires total disassembly for cleaning and inspection.
Sanitization
It is important to differentiate and define certain terminology:
- Sterilize refers to the statistical destruction and removal of all living organisms.
- Disinfect refers to inanimate objects and the destruction of all vegetative cells (not spores).
- Sanitize refers to the reduction of microorganisms to levels considered safe from a public health viewpoint.
Appropriate and approved sanitization procedures are processes, and, thus, the duration or time as well as the chemical conditions must be described. The official definition (Association of Official Analytical Chemists) of sanitizing for food product contact surfaces is a process which reduces the contamination level by 99.999% (5 logs) in 30 sec.
The official definition for non-product contact surfaces requires a contamination reduction of 99.9% (3 logs). The standard test organisms used are Staphylococcus aureus and Escherichia coli.
General types of sanitization include the following:
- Thermal Sanitization involves the use of hot water or steam for a specified temperature and contact time.
- Chemical Sanitization involves the use of an approved chemical sanitizer at a specified concentration and contact time.
Water Chemistry and Quality
Water comprises approximately 95–99% of cleaning and sanitizing solutions. Water functions to do the following:
- carry the detergent or the sanitizer to the surface
- carry soils or contamination from the surface.
The impurities in water can drastically alter the effectiveness of a detergent or a sanitizer. Water hardness is the most important chemical property with a direct effect on cleaning and sanitizing efficiency. (Other impurities can affect the food contact surface or may affect the soil deposit properties or film formation.)
Water pH ranges generally from pH 5 to 8.5. This range is of no serious consequence to most detergents and sanitizers. However, highly alkaline or highly acidic water may require additional buffering agents.
Water can also contain significant numbers of microorganisms. Water used for cleaning and sanitizing must be potable and pathogen-free. Treatments and sanitization of water may be required prior to use in cleaning regimes. Water impurities that affect cleaning functions are presented in Table 1.
Cleaning
Properties of Food Soils
Food soil is generally defined as unwanted matter on food-contact surfaces. Soil is visible or invisible. The primary source of soil is from the food product being handled. However, minerals from water residue and residues from cleaning compounds contribute to films left on surfaces. Microbiological biofilms also contribute to the soil buildup on surfaces.
Since soils vary widely in composition, no one detergent is capable of removing all types. Many complex films contain combinations of food components, surface oil or dust, insoluble cleaner components, and insoluble hard-water salts. These films vary in their solubility properties depending upon such factors as heat effect, age, dryness, time, etc.
It is essential that personnel involved have an understanding of the nature of the soil to be removed before selecting a detergent or cleaning regime. The rule of thumb is that acid cleaners dissolve alkaline soils (minerals) and alkaline cleaners dissolve acid soils and food wastes. Improper use of detergents can actually "set" soils, making them more difficult to remove (e.g., acid cleaners can precipitate protein). Many films and biofilms require more sophisticated cleaners that are amended with oxidizing agents (such as chlorinated detergents) for removal.
Soils may be classified as the following:
- soluble in water (sugars, some starches, most salts);
- soluble in acid (limestone and most mineral deposits);
- soluble in alkali (protein, fat emulsions);
- soluble in water, alkali, or acid.
The physical condition of the soil deposits also affects its solubility. Freshly precipitated soil in a cool or cold solution is usually more easily dissolved than an old, dried, or baked-on deposit, or a complex film. Food soils are complex in that they contain mixtures of several components. A general soil classification and removal characteristics are presented in Table 2 .
Fat-Based Soils
Fat usually is present as an emulsion and can generally be rinsed away with hot water above the melting point. More difficult fat and oil residues can be removed with alkaline detergents, which have good emulsifying or saponifying ingredients.
Protein-Based Soils
In the food industry, proteins are by far the most difficult soils to remove. In fact, casein (a major milk protein) is used for its adhesive properties in many glues and paints. Food proteins range from more simple proteins, which are easy to remove, to more complex proteins, which are very difficult to remove. Heat-denatured proteins can be extremely difficult.
Generally, a highly alkaline detergent with peptizing or dissolving properties is required to remove protein soils. Wetting agents can also be used to increase the wettability and suspendability of proteins. Protein films require alkaline cleaners that have hypochlorite in addition to wetting agents.
Carbohydrate-Based Soils
Simple sugars are readily soluble in warm water and are quite easily removed. Starch residues, individually, are also easily removed with mild detergents. Starches associated with proteins or fat scan usually be easily removed by highly alkaline detergents.
Mineral Salt-Based Soils
Mineral salts can be either relatively easy to remove or be highly troublesome deposits or films. Calcium and magnesium are involved in some of the most difficult mineral films. Under conditions involving heat and alkaline pH, calcium and magnesium can combine with bicarbonates to form highly insoluble complexes. Other difficult deposits contain iron or manganese. Salt films can also cause corrosion of some surfaces. Difficult salt films require an acid cleaner (especially organic acids that form complexes with these salts) for removal. Sequestering agents such as phosphates or chelating agents are often used in detergents for salt film removal.
Microbiological Films
Under certain conditions, microorgranisms (bacteria, yeasts, and molds) can form invisible films (biofilms) on surfaces. Biofilms can be difficult to remove and usually require cleaners as well as sanitizers with strong oxidizing properties.
Lubricating Greases and Oils
These deposits (insoluble in water, alkali, or acid) can often be melted with hot water or steam, but often leave a residue. Surfactants can be used to emulsify the residue to make it suspendable in water and flushable.
Other Insoluble Soils
Inert soils such as sand, clay, or fine metal can be removed by surfactant-based detergents. Charred or carbonized material may require organic solvents.
Quantity of Soil
It is important to rinse food-contact surfaces prior to cleaning to remove most of the soluble soil. Heavy deposits require more detergent to remove. Improper cleaning can actually contribute to build-up of soil.
The Surface Characteristics
The cleanability of the surface is a primary consideration in evaluating cleaning effectiveness. Included in surface characteristics are the following:
Surface Composition
Stainless steel is the preferred surface for food equipment and is specified in many industry and regulatory design and construction standards. For example, 3-A Sanitary Standards (equipment standards used for milk and milk products applications) specify 300 series stainless steel or equivalent. Other grades of stainless steel may be appropriate for specific applications (i.e., 400 series) such as handling of high fat products, meats, etc. For highly acidic, high salt, or other highly corrosive products, more corrosion resistant materials (i.e., titanium) is often recommended.
Other "soft" metals (aluminum, brass, copper, or mild steel), or nonmetallic surfaces (plastics or rubber) are also used on food contact surfaces. Surfaces of soft metals and nonmetallic materials are generally less corrosion-resistant and care should be exercised in their cleaning.
Aluminum is readily attacked by acids as well as highly alkaline cleaners, which can render the surface non-cleanable. Plastics are subject to stress cracking and clouding from prolonged exposure to corrosive food materials or cleaning agents.
Hard wood (maple or equivalent) or sealed wood surfaces should be used only in limited applications such as cutting boards or cutting tables, provided the surface is maintained in good repair. Avoid using porous wood surfaces.
Surface Finish
Equipment design and construction standards also specify finish and smoothness requirements. 3-A standards specify a finish at least as smooth as a No. 4 ground finish for most applications. With high-fat products, a less smooth surface is used to allow product release from the surface.
Surface Condition
Misuse or mishandling can result in pitted, cracked, corroded, or roughened surfaces. Such surfaces are more difficult to clean or sanitize, and may no longer be cleanable. Thus, care should be exercised in using corrosive chemicals or corrosive food products.
Environmental Considerations
Detergents can be significant contributors to the waste discharge (effluent). Of primary concern is pH. Many publicly owned treatment works limit effluent pH to the range of 5 to 8.5. So it is recommended that in applications where highly alkaline cleaners are used, that the effluent be mixed with rinse water (or some other method be used) to reduce the pH. Recycling of caustic soda cleaners is also becoming a common practice in larger operations. Other concerns are phosphates, which are not tolerated in some regions of the U.S., and the overall soil load in the waste stream that contributes to the chemical oxygen demand (COD) and biological oxygen demand (BOD).
Chemistry of Detergents
Detergents and cleaning compounds are usually composed of mixtures of ingredients that interact with soils in several ways:
- Physically active ingredients alter physical characteristics such as solubility or colloidal stability.
- Chemically active ingredients modify soil components to make them more soluble and, thus, easier to remove.
In some detergents, specific enzymes are added to catalytically react with and degrade specific food soil components.
Physically Active Ingredients
The primary physically-active ingredients are the surface active compounds termed surfactants. These organic molecules have general structural characteristic where a portion of the structure is hydrophilic (water-loving) and a portion is hydrophobic (not reactive with water). Such molecules function in detergents by promoting the physical cleaning actions through emulsification, penetration, spreading, foaming, and wetting.
The classes of surfactants are as follows:
- Ionic surfactants that are negatively charged in water solution are termed anionic surfactants. Conversely, positively charged ionic surfactants are termed cationic surfactants. If the charge of the water soluble portion depends upon the pH of the solution, it is termed an amphoteric surfactant. These surfactants behave as cationic surfactants under acid conditions, and as anionic surfactants under alkaline conditions. Ionic surfactants are generally characterized by their high foaming ability.
- Nonionic surfactants, which do not dissociate when dissolved in water, have the broadest range of properties depending upon the ratio of hydrophilic/hydrophobic balance. This balance are also affected by temperature. For example, the foaming properties of nonionic detergents is affected by temperature of solution. As temperature increases, the hydrophobic character and solubility decrease. At the cloud point (minimum solubility), these surfactants generally act as defoamers, while below the cloud point they are varied in their foaming properties.
It is a common practice to blend surfactant ingredients to optimize their properties. However, because of precipitation problems, cationic and anionic surfactants cannot be blended.
Chemically Active Ingredients
Alkaline Builders
Highly Alkaline Detergents (or heavy-duty detergents) use caustic soda (sodium hydroxide) or caustic potash (potassium hydroxide). An important property of these highly alkaline detergents is that they saponify fats: forming soap. These cleaners are used in many CIP systems or bottle-washing applications.
Moderately Alkaline Detergents include sodium, potassium, or ammonium salts of phosphates, silicates, or carbonates. Tri-sodium phosphate (TSP) is one of the oldest and most effective. Silicates are most often used as a corrosion inhibitor. Because of interaction with calcium and magnesium and film formation, carbonate-based detergents are of only limited use in food processing cleaning regimes.
Acid Builders
Acid Detergents include organic and inorganic acids. The most common inorganic acids used include phosphoric, nitric, sulfamic, sodium acid sulfate, and hydrochloric. Organic acids, such as hydroxyacetic, citric, and gluconic, are also in use. Acid detergents are often used in a two-step sequential cleaning regime with alkaline detergents. Acid detergents are also used for the prevention or removal of stone films (mineral stone, beer stone, or milk stone).
Water Conditioners
Water conditioners are used to prevent the build-up of various mineral deposits (water hardness, etc.). These chemicals are usually sequestering agents or chelating agents. Sequestering agents form soluble complexes with calcium and magnesium. Examples are sodium tripolyphosphate, tetra-potassium pyrophosphate, organo-phosphates, and polyelectrolytes. Chelating agents include sodium gluconate and ethylene diamine tetracetic acid (EDTA).
Oxidizing Agents
Oxidizing agents used in detergent application are hypochlorite (also a sanitizer) and—to a lesser extent—perborate. Chlorinated detergents are most often used to clean protein residues.
Enzyme Ingredients
Enzyme-based detergents, which are amended with enzymes such as amylases and other carbohydrate-degrading enzymes, proteases, and lipases, are finding acceptance in specialized food industry applications.
The primary advantages of enzyme detergents are that they are more environmentally friendly and often require less energy input (less hot water in cleaning). Uses of most enzyme cleaners are usually limited to unheated surfaces (e.g., cold-milk surfaces). However, new generation enzyme cleaners (currently under evaluation) are expected to have broader application.
Fillers
Fillers add bulk or mass, or dilute dangerous detergent formulations that are difficult to handle. Strong alkalis are often diluted with fillers for ease and safety of handling. Water is used in liquid formulations as a filler. Sodium chloride or sodium sulfate are often fillers in powdered detergent formuations.
Miscellaneous Ingredients
Additional ingredients added to detergents may include corrosion inhibitors, glycol ethers, and butylcellosolve (improve oil, grease, and carbon removal).
Sanitizing
Thermal Sanitizing
As with any heat treatment, the effectiveness of thermal sanitizing is dependant upon a number of factors including initial contamination load, humidity, pH, temperature, and time.
Steam
The use of steam as a sanitizing process has limited application. It is generally expensive compared to alternatives, and it is difficult to regulate and monitor contact temperature and time. Further, the byproducts of steam condensation can complicate cleaning operations.
Hot Water
Hot-water sanitizing—through immersion (small parts, knives, etc.), spray (dishwashers), or circulating systems—is commonly used. The time required is determined by the temperature of the water. Typical regulatory requirements (Food Code 1995) for use of hot water in dishwashing and utensil sanitizing applications specify immersion for at least 30 sec. at 77°C (170°F) for manual operations; and a final rinse temperature of 74°C (165°F) in single tank, single temperature machines and 82°C (180°F) for other machines.
Many state regulations require a utensil surface temperature of 71°C (160°F), as measured by an irreversibly registering temperature indicator in warewashing machines. Recommendations and requirements for hot-water sanitizing in food processing may vary. The Grade A Pasteurized Milk Ordinance specifies a minimum of 77°C (170°F) for 5 min. Other recommendations for processing operations are 85°C (185°F) for 15 min., or 80°C (176°F) for 20 min.
The primary advantages of hot-water sanitization are relatively inexpensive, easy to apply, and readily available, generally effective over a broad range of microorganisms, relatively non-corrosive, and penetrates into cracks and crevices. Hot-water sanitization is a slow process that requires come-up and cool-down time; can have high energy costs; and has certain safety concerns for employees. The process also has the disadvantages of forming or contributing to film formations and shortening the life of certain equipment or parts thereof (gaskets, etc.).
Chemical Sanitizing
The ideal chemical sanitizer should:
- be approved for food contact surface application.
- have a wide range or scope of activity.
- destroy microorganisms rapidly.
- be stable under all types of conditions.
- be tolerant of a broad range of environmental conditions.
- be readily solubilized and possess some detergency.
- be low in toxicity and corrosivity.
- be inexpensive.
No available sanitizer meets all of the above criteria. Therefore, it is important to evaluate the properties, advantages, and disadvantages of available sanitizer for each specific application.
Regulatory Considerations
The regulatory concerns involved with chemical sanitizers are antimicrobial activity or efficacy, safety of residues on food contact surfaces, and environmental safety. It is important to follow regulations that apply for each chemical usage situation. The registration of chemical sanitizers and antimicrobial agents for use on food and food product contact surfaces and on nonproduct contact surfaces is through the U.S. Environmental Protection Agency (EPA). (Prior to approval and registration, the EPA reviews efficacy and safety data, and product labeling information.)
The U.S. Food and Drug Administration (FDA) is primarily involved in evaluating residues form sanitizer use that may enter the food supply. Thus, any antimicrobial agent and its maximum usage level for direct use on food or on food product contact surfaces must be approved by the FDA. Approved no-rinse food contact sanitizers and nonproduct contact sanitzers, their formulations and usage levels are listed in the Code of Federal Regulations (21 CFR 178.1010). The U.S. Department of Agriculture (USDA) also maintains lists of antimicrobial compounds (i.e., USDA List of Proprietary Substances and Non Food Product Contact Compounds), which are primarily used in the regulation of meats, poultry, and related products by USDA's Food Safety and Inspection Service (FSIS).
Factors Affecting Sanitizer Effectiveness
Physical Factors
Surface Characteristics. Prior to the sanitization process, all surfaces must be clean and thoroughly rinsed to remove any detergent residue. An unclean surface cannot be sanitized. Since the effectiveness of sanitization requires direct contact with the microorganisms, the surface should be free of cracks, pits, or crevices which can harbor microorganisms. Surfaces which contain biofilms cannot be effectively sanitized.
Exposure Time. Generally, the longer time a sanitizer chemical is in contact with the equipment surface, the more effective the sanitization effect; intimate contact is as important as prolonged contact.
Temperature. Temperature is also positively related to microbial kill by a chemical sanitizer. Avoid high temperatures (above 55°C [131°F]) because of the corrosive nature of most chemical sanitizers.
Concentration. Generally, the activity of a sanitizer increases with increased concentration. However, a leveling off occurs at high concentrations. A common misconception regarding chemicals is that "if a little is good, more is better". Using sanitizer concentrations above recommendations does not sanitizer better and, in fact, can be corrosive to equipment and in the long run lead to less cleanability. Follow manufacturer's label instructions.
Soil. The presence of organic matter dramatically reduces the activity of sanitizers and may, in fact, totally inactivate them. The adage is "you cannot sanitize an unclean surface".
Chemical Factors
pH. Sanitizers are dramatically affected by the pH of the solution. Many chlorine sanitizers, for example, are almost ineffective at pH values above 7.5.
Water properties. Certain sanitizers are markedly affected by impurities in the water.
Inactivators. Organic and/or inorganic inactivators may react chemically with sanitizers giving rise to non-germicidal products. Some of these inactivators are present in detergent residue. Thus, it is important that surfaces be rinsed prior to sanitization.
Biological Factors
The microbiological load can affect sanitizer activity. Also, the type of microorganism present is important. Spores are more resistant than vegetative cells. Certain sanitizers are more active against gram positive than gram negative microorganisms, and vice versa. Sanitizers also vary in their effectiveness against yeasts, molds, fungi, and viruses.
Specific Types of Chemical Sanitizers
The chemicals described here are those approved by FDA for use as no-rinse, food-contact surface sanitizers. In food-handling operations, these are used as rinses, sprayed onto surfaces, or circulated through equipment in CIP operations. In certain applications the chemicals are foamed on a surface or fogged into the air to reduce airborne contamination.
Chlorine-Based Sanitizers
Chlorine Compounds. Chlorine, in its various forms, is the most commonly used sanitizer in food processing and handling applications. Commonly used chlorine compounds include liquid chlorine, hypochlorites, inorganic chloramines, and organic chloramines. Chlorine-based sanitizers form hypochlorous acid (HOCl, the most active form) in solution. Available chlorine (the amount of HOCl present) is a function of pH. At pH 5, nearly all is in the form of HOCl. At pH 7.0, approximately 75% is HOCl. The maximum allowable level for no-rinse applications is 200ppm available chlorine, but recommended usage levels vary. For hypochlorites, an exposure time of 1 min at a minimum concentration of 50ppm and a temperature of 24°C (75°F) is recommended. For each 10°C (18°F) drop in temperature, a doubling of exposure time is recommended. For chloramines, 200ppm for 1 min is recommended.
Chlorine compounds are broad spectrum germicides that act on microbial membranes, inhibit cellular enzymes involved in glucose metabolism, have a lethal effect on DNA, and oxidize cellular protein. Chlorine has activity at low temperature, is relatively cheap, and leaves minimal residue or film on surfaces.
The activity of chlorine is dramatically affected by such factors as pH, temperature, and organic load. However, chlorine is less affected by water hardness when compared to other sanitizers (especially the quaternary ammonium compounds).
The major disadvantage to chlorine compound is corrosiveness to many metal surfaces (especially at higher temperatures). Health and safety concerns can occur because of skin irritation and mucous membrane damage in confined areas. At low pH (below 4.0), deadly Cl2 (mustard gas) can form. In recent years, concerns have also been raised about the use of chlorine as a drinking water disinfectant and as an antimicrobial with direct food contact (meat, poultry and shellfish). This concern is based upon the involvement of chlorine in the formation of potentially carcinogenic trihalomethanes (THMs) under appropriate conditions. While chlorine's benefits as a sanitizer far outweigh these risks, it is under scrutiny.
Chlorine dioxide. Chlorine dioxide (ClO2) is currently being considered as a replacement for chlorine, since it appears to be more environmentally friendly. Stabilized ClO2 has FDA approval for most applications in sanitizing equipment or for use as a foam for environmental and non-food contact surfaces. Approval has also been granted for use in flume waters in fruits and vegetable operations and in poultry process waters. ClO2 has 2.5 times the oxidizing power of chlorine and, thus, less chemical is required. Typical use concentrations range from 1 to 10ppm.
CLO2's primary disadvantages are worker safety and toxicity. Its highly concentrated gases can be explosive and exposure risks to workers are higher than that for chlorine. Its rapid decomposition in the presence of light or at temperatures greater than 50°C (122°F) makes on-site generation a recommended practice.
Iodine
Use of iodine as an antimicrobial agents dates back to the 1800s. This sanitizer exists in many forms and usually exists with a surfactant as a carrier. These mixtures are termed iodophors. The most active agent is the dissociated free iodine (also less stable). This form is most prevalent at low pH. The amount of dissociation from the surfactant is dependent upon the type of surfactant. Iodine solubility is very limited in water. Generally recommended usage for iodophors is 12.5 to 25ppm for 1 min.
It is generally thought that the bactericidal activity of iodine is through direct halogenation of proteins. More recent theories have centered upon cell wall damage and destruction of microbial enzyme activity.
Iodophors, like chlorine compounds, have a very broad spectrum: being active against bacteria, viruses, yeasts, molds, fungi, and protozoans. Iodine is highly temperature-dependent and vaporizes at 120°F. Thus, it is limited to lower temperature applications. The degree to which iodophors are affected by environmental factors is highly dependant upon properties of the surfactant used in the formulation. Iodophors are generally less affected by organic matter and water hardness than chlorine. However, loss of activity is pronounced at high pH.
Iodine has a long history of use in wound treatment. However, ingestion of iodine gas does pose a toxicity risk in closed environments. The primary disadvantage is that iodine can cause staining on some surfaces (especially plastics).
Quaternary Ammonium Compounds (QACs)
Quaternary ammonium compounds (QACs) are a class of compounds that have the general structure as follows (Figure 1):
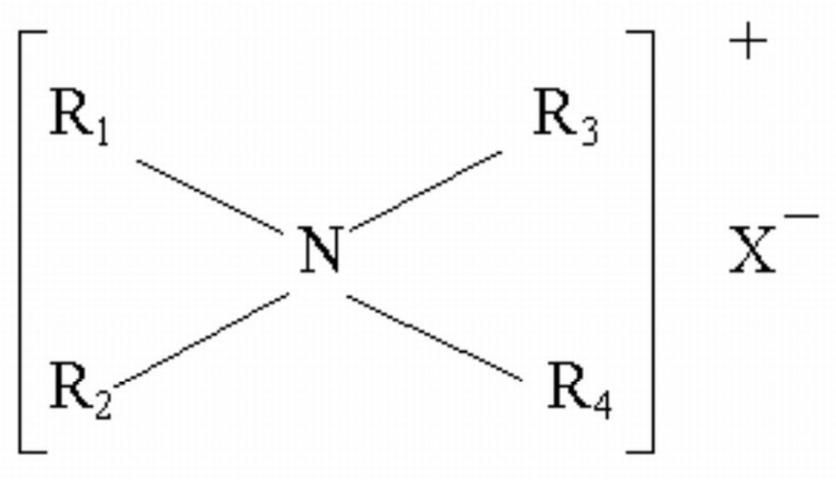
The properties of these compounds depend upon the covalently bound alkyl groups (R groups), which can be highly diverse. Since QACs are positively charged cations, their mode of action is related to their attraction to negatively charged materials such as bacterial proteins. It is generally accepted that the mode of action is at the membrane function. The carbon length of R-group side chain is, generally, directly related with sanitizer activity in QACs. However, because of the lower solubility in QACs composed of large carbon chains, these sanitizers may have lower activity than short chain structures.
QACs are active and stable over a broad temperature range. Because they are surfactants, they possess some detergency. Thus, they are less affected by light soil than are other sanitizers. However, heavy soil dramatically decreases activity. QACs generally have higher activity at alkaline pH. While lack of tolerance to hard water is often listed as a major disadvantage of QACs when compared to chlorine, some QACs are fairly tolerant of hard water. Activity can be improved by the use of EDTA as a chelator. QACs are effective against bacteria, yeasts, mold, and viruses.
An advantage of QACs in some applications is that they leave a residual antimicrobial film. However, this would be a disadvantage in operations such as cultured dairy products, cheese, beer, etc., where microbial starter cultures are used.
QACs are generally more active against gram positive than gram negative bacteria. They are not highly effective against bacteriophages. Their incompatibility with certain detergents makes thorough rinsing following cleaning operations imperative. Further, many QAC formulations can cause foaming problems in CIP applications.
Under recommended usage and precautions, QACs pose little toxicity or safety risks. Thus, they are in common use as environmental fogs and as room deodorizers. However, care should be exercised in handling concentrated solutions or use as environmental fogging agents.
Acid-Anionic Sanitizers
Like QACs, acid-anionic sanitizers are surface-active sanitizers. These formulations include an inorganic acid plus a surfactant and are often used for the dual function of acid rinse and sanitization.
Whereas QACs are positively charged, these sanitizers are negatively charged. Their activity is moderately affected by water hardness. Their low use pH, detergency, stability, low odor potential, and non-corrosiveness make them highly desirable in some applications.
Disadvantages include relatively high cost, a closely defined pH range of activity (pH 2 to 3), low activity on molds and yeasts, excessive foaming in CIP systems, and incompatibility with cationic surfactant detergents.
Fatty Acid Sanitizers
Fatty acid or carboxylic acid sanitizers were developed in the 1980s. Typical formulations include fatty acids plus other acids (phosphoric acids, organic acids). These agents also have the dual function of acid rinse and sanitization. The major advantage over acid anionics is lower foaming potential. These sanitizers have a broad range of activity, are highly stable in dilute form, are stable to organic matter, and are stable to high temperature applications.
These sanitizers have low activity above pH 3.5–4.0, are not very effective against yeasts and molds, and some formulations lose activity at temperatures below 10°C (50°F). They also can be corrosive to soft metals and can degrade certain plastics and rubber.
Peroxides
Peroxides or peroxy compounds contain at least one pair of covalently bonded oxygen atoms (-O-O-) and are divided into two groups: the inorganic group, containing hydrogen peroxide (HP) and related compounds; and the organic group, containing peroxyacetic acid (PAA) and related compounds.
Hydrogen peroxide (HP), while widely used in the medical field, has found only limited application in the food industry. FDA approval has been granted for HP use for sterilizing equipment and packages in aseptic operations.
The primary mode of action for HP is through creating an oxidizing environment and generation of singlet or superoxide oxygen (SO). HP is fairly broad spectrum with slightly higher activity against gram-negative than gram-positive organisms.
High concentrations of HP (5% and above) can be an eye and skin irritant. Thus, high concentrations should be handled with care.
Peroxyacetic Acid (PAA) has been known for its germicidal properties for a long time. However, it has only found food-industry application in recent years and is being promoted as a potential chlorine replacement. PAA is relatively stable at use strengths of 100 to 200ppm. Other desirable properties include absence of foam and phosphates, low corrosiveness, tolerance to hard water, and favorable biodegradability. PAA solutions have been shown to be useful in removing biofilms.
While precise mode of action mechanisms have not been determined, it is generally theorized that the PAA reaction with microorganisms is similar to that of HP. PAA, however, is highly active against both gram-positive and gram-negative microorganisms. The germicidal activity of PAA is dramatically affected by pH. Any pH increase above 7–8 drastically reduces the activity.
PAA has a pungent odor and the concentrated product (40%) is a highly toxic, potent irritant, and powerful oxidizer. Thus, care must be used in its use.
A general comparison of the chemical and physical properties of commonly used sanitizers is presented in Table 3.
References Used
Bakka, R.L. 1995. Making the Right Choice - Cleaners. Ecolab, Inc./Food & Beverage Div., St. Paul, MN.
Barnard, S. Extension. Handout. Penn. State Univ.
Boufford, T. 1996. Making the Right Choice - Sanitizers. Ecolab, Inc./Food & Beverage Div., St. Paul, MN.
Cords, B.R. and G.R. Dychdala. 1993. Sanitizers: Halogens, Surface-Active Agents, and Peroxides. Pp. 36-52. In: P M. Davidson and A. L. Branen, (eds.). Antimicrobials in Foods. Marcel Dekker, Inc., New York, NY.
Food Code 1995. U.S. Public Health Service, Food and Drug Admin., Washington, DC.
Grade A Pasteurized Milk Ordinance, 1995. Revision. U.S. Public Health Service, FDA, Washington, DC.
Marriott, N.G. 1994. Cleaning compounds for Effective Sanitation. Pp. 85-113. Sanitatizers for Effective Sanitation. Pp. 114-166. Principles of Food Sanitation. Chapman & Hall, New York, NY.