Summary
Container mosquitoes inhabit small natural and artificial water-filled vessels during their immature stages. This publication lists and illustrates fifteen species of mosquitoes, eleven native and four exotic, that preferentially occupy containers in Florida. Two of the exotics are the invasive yellow fever mosquito (Aedes aegypti) and Asian tiger mosquito (Aedes albopictus), the most important vector species of dengue, chikungunya, yellow fever, and Zika viruses. True container mosquitoes are differentiated from other common species that may occasionally be found in containers. Many methods, including some novel techniques still under development, are available for controlling container mosquitoes. Novel control methods include autocidal gravid ovitraps (AGO traps), autodissemination of insect growth regulators by adult mosquitoes, and releases of altered mosquitoes, which generally require scientific oversight and the support of communities and governmental or non-governmental organizations. Among control methods that can be easily applied by homeowners, the most efficacious is reducing container mosquito numbers via source reduction, i.e., eliminating the aquatic habitat favorable for mosquito growth. Alternatively, biorational products containing the active ingredients Bacillus thuringiensis subspecies israelensis (BTI) or insect growth regulators may be added to container habitats to kill developing immature mosquitoes with minimal or no non-target effects.
Introduction
Adult female container mosquitoes lay their eggs in small vessels that hold water. The immatures that hatch spend their aquatic stages in these containers until they emerge as terrestrial adults. Container mosquitoes represent approximately twenty percent of the ninety mosquito species currently known to occur in Florida. This publication describes types of container habitats used by Florida mosquitoes and which species are "true" container inhabitants compared to those which occasionally occupy containers. A brief description of each container species is provided, and methods for controlling pest or disease-vectoring container mosquitoes are discussed.
The intended audience of this publication is anyone with the curiosity or professional needs to read it. For either of these audiences, ample references are provided for deeper inquiries into selected aspects, such as vector control, mosquito ecology, and public health.
What is a container habitat?
Container mosquitoes are called such because of the typically small aquatic habitats that they occupy during their immature stages. Previous efforts to classify the larval habitats of these mosquitoes distinguished between natural and artificial containers (Bates 1949; Laird 1988). The natural container habitats used by mosquitoes are usually phytotelmata, the small water bodies held by terrestrial plants, such as bromeliad tanks and axils, tree holes, flower bracts of tropical plants, and pitcher plants. Plant parts that collect rainwater after falling to the ground, such as fruit husks, fungus caps, and palm spathes are additional examples of phytotelmata used as habitats by immature mosquitoes (Figure 1; Kitching 2000). Rock holes (Figure 2A) that collect rainwater or splash from nearby streams, lakes, or oceans represent natural but non-living containers used for development by a few species of mosquitoes. Empty snail shells (Figure 2B) that collect water account for another natural, but non-living, container suitable for the growth and development of immature stages of some mosquitoes (e.g., Burkett-Cadena and Unnasch 2014).
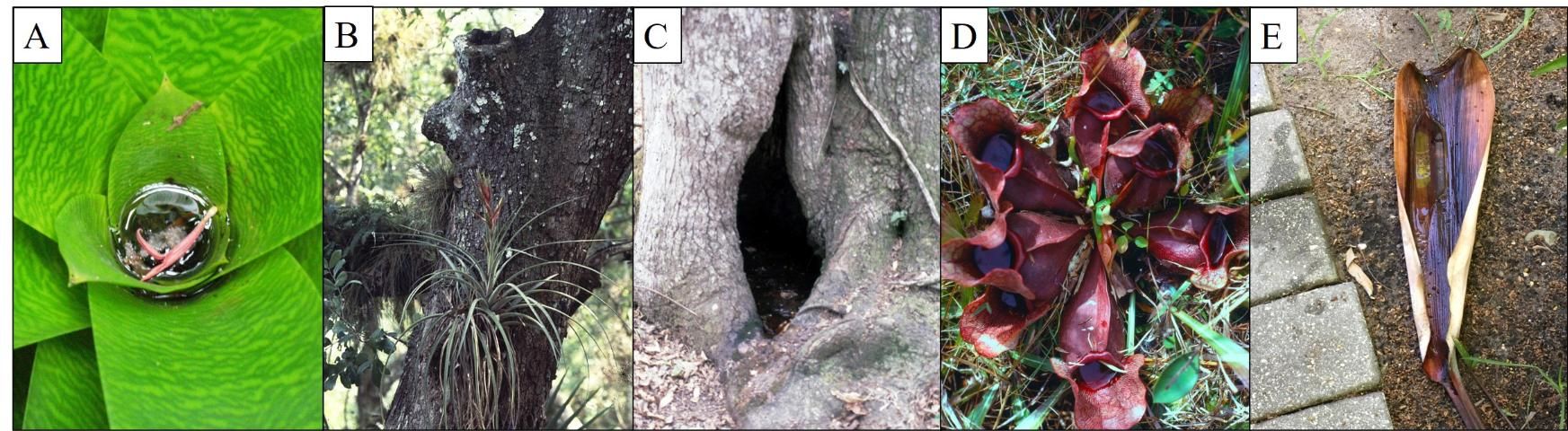
Credit: A–D: L. P. Lounibos, UF/IFAS. E: E. A. Buckner, UF/IFAS
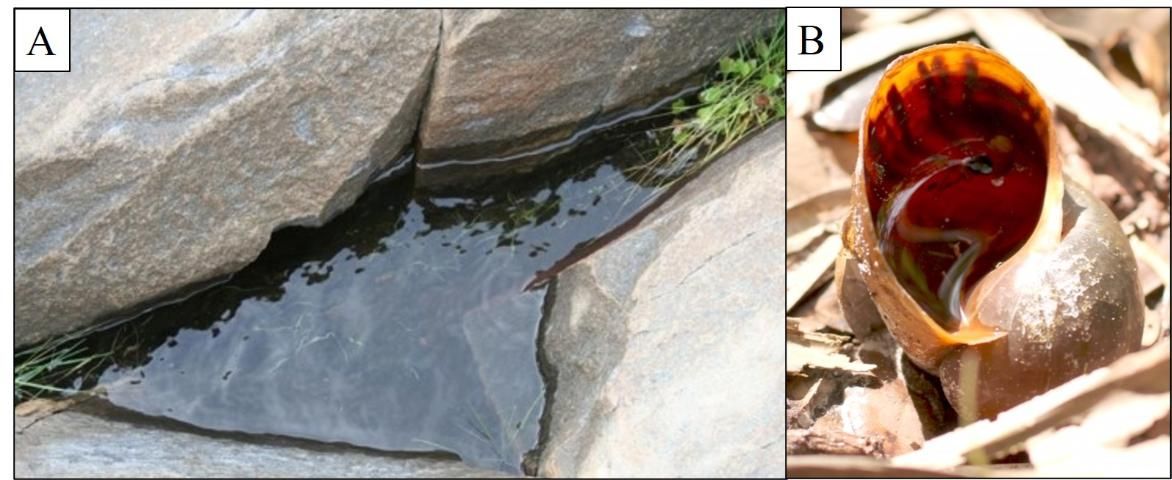
Credit: A: G. F. O'Meara, UF/IFAS B: N. D. Burkett-Cadena, UF/IFAS
As some mosquito species that originally occupied phytotelmata or rock holes became associated with humans, artificial containers offered alternative and additional aquatic microhabitats. Jars and barrels for domestic water storage, plant pots and saucers and other containers that collect rainwater, discarded automobile tires, or neglected bird baths and fountains in suburban yards all provide mosquitoes with viable habitat (Figure 3). Ranking the relative importance of artificial containers for the productivity of local container-inhabiting disease vectors is a common research objective to prioritize mosquito control efforts (e.g., Hribar and Whiteside 2010).
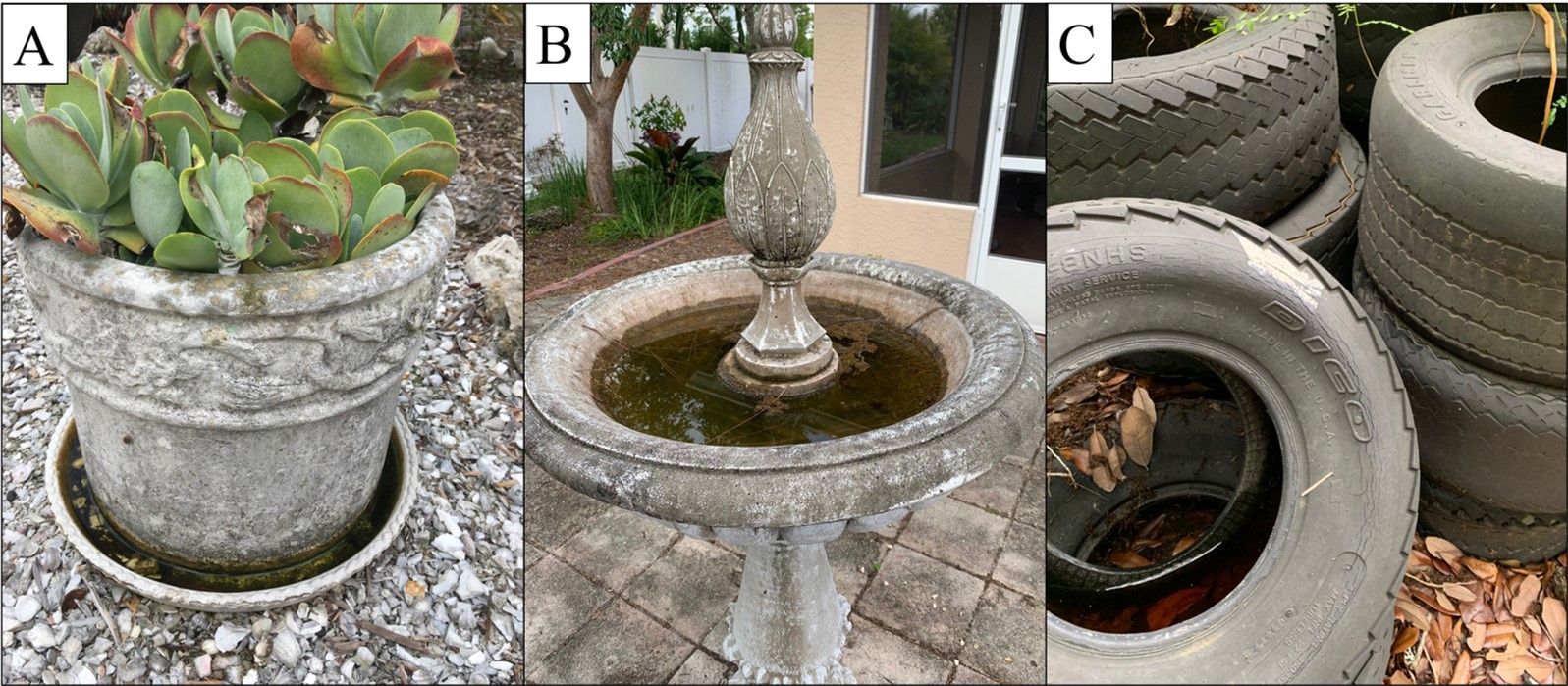
Mosquito Nomenclature
This publication uses the most accepted genus and species names for container mosquitoes, retaining the generic status of Aedes to preserve stability (Wilkerson et al. 2015). Although common names are provided when available (e.g., Burkett-Cadena 2016), these do not have the widespread acceptance or stability of the scientific names.
Invasive Mosquito Container Species
Some of Florida's container mosquitoes are invasive species, i.e., non-native to the United States, introduced inadvertently by humans, and having a known or potential negative impact on human or ecosystem health (Juliano and Lounibos 2005). The transport of containers occupied by immature stages of these species has facilitated their international and local spread (Juliano and Lounibos 2005). Both the yellow fever mosquito, Aedes aegypti, introduced by the slave trade from Africa during the 15th–17th centuries (Lounibos 2002), and the Asian tiger mosquito, Aedes albopictus, spread from Asia by the international trade of used tires in the late 20th century (Lounibos 2002), are currently widespread in Florida (Parker et al. 2019). Because these two container mosquito species are broadly distributed in Florida and the most important vectors of the arboviruses (arthropod-borne viruses) that cause dengue, chikungunya, and Zika diseases, their surveillance and control are a priority of many of Florida's mosquito control programs.
Distinguishing between (a) Exclusive or Routine and (b) Occasional Container Use by Mosquito Species
Early research on treehole and pitcher plant communities distinguished between aquatic fauna, mainly insects, that occupied these habitats (a) exclusively, (b) routinely but not exclusively, or (c) occasionally (Röhnert 1950; Thienemann 1932; Kitching 2000). Recognizing that similar distinctions apply to mosquitoes in all container habitats, this overview will focus on Florida species that occupy containers exclusively or routinely, with only minor mention of those species found only occasionally in container habitats.
Exclusive or Routine "True" Container Mosquitoes
This cosmopolitan species, native to Africa, has spread throughout most of the Western Hemisphere since its first introductions to the United States and the New World tropics during the slave trade (Lounibos 2002). Currently found in all of Florida except the Panhandle (Parker et al. 2019), this species is highly adapted to urban and suburban environments, where it develops in containers that hold stored water or rainwater (Braks et al. 2003). Aedes aegypti (Figure 4) is currently regarded as the most important vector species of dengue, chikungunya, and Zika viruses, both in Florida and worldwide.
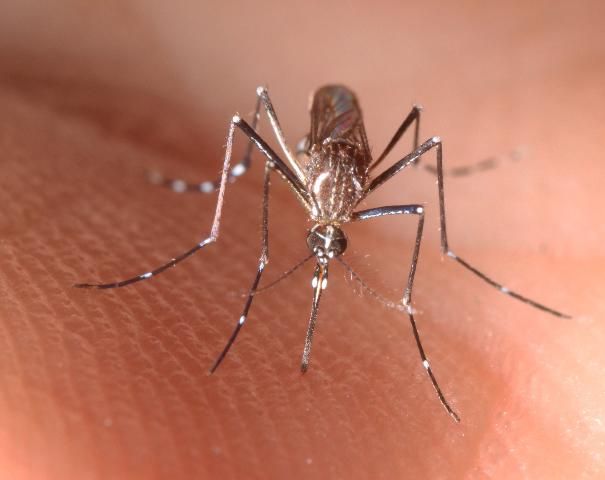
Credit: N. D. Burkett-Cadena, UF/IFAS
2. Aedes albopictus Skuse (Asian tiger mosquito)
This invasive species became established in Texas in the mid-1980s before entering Florida from the north and sweeping southward through the peninsula (Lounibos 2002). During its spread from Texas into the southeastern United States, A. albopictus (Figure 5) competitively displaced A. aegypti from parts of its former range (Lounibos 2002; Lounibos and Juliano 2018). Although A. albopictus is currently distributed throughout Florida, where it often co-occurs with A. aegypti, the Asian tiger mosquito is preferentially found in containers in vegetated zones, such as suburban yards (Braks et al. 2003).
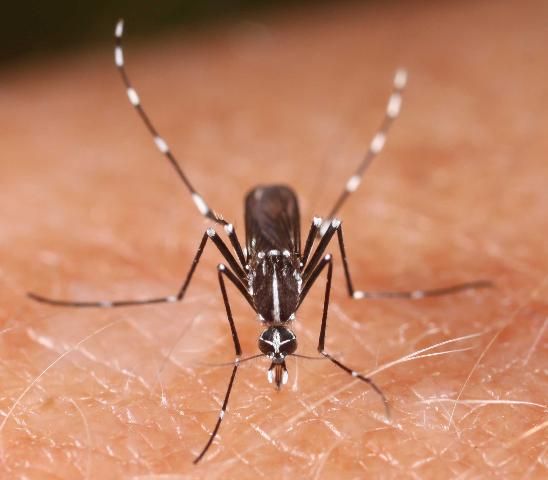
Credit: N. D. Burkett-Cadena, UF/IFAS
3. Aedes bahamensis Berlin (Bahamian container mosquito)
This non-native species was first discovered in Florida during surveillance for the Asian tiger mosquito (Pafume et al. 1988). Because it is not known to have spread outside of Broward and Miami-Dade Counties and does not require blood to develop an egg batch (O'Meara et al. 1993), A. bahamensis (Figure 6) is not regarded as a public health threat comparable to the two previous, invasive species.
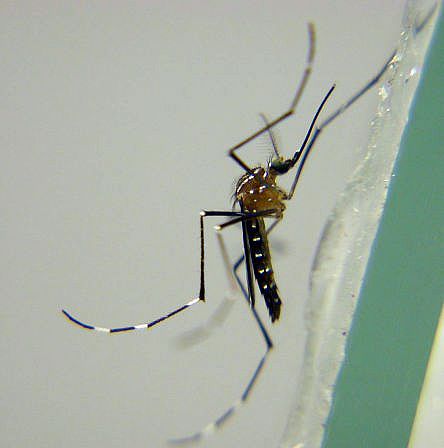
Credit: M. Cutwa, UF/IFAS
4. Aedes hendersoni Cockerell (Eastern tree hole mosquito sibling species)
To date, this species has been identified in Florida from only the more northern, temperate area of the state (Zavortink and Belkin 1979) where it co-occurs with its sister species, Aedes triseriatus (see below). The two vertically segregate in hardwood forests of the United States, with A. hendersoni (Figure 7) preferring higher treeholes and A. triseriatus lower (Scholl and DeFoliart 1977).
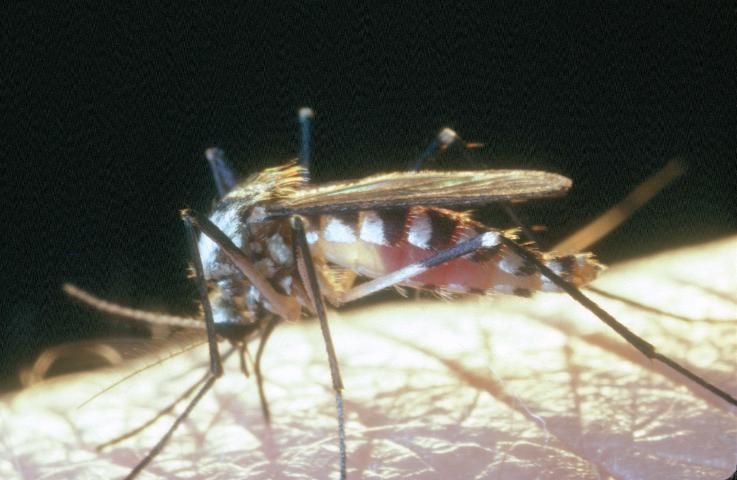
Credit: L. E. Munstermann, Yale University
5. Aedes japonicus (Theobald) (Asian rock hole mosquito)
This invasive temperate species, originating from Japan and Korea (Fonseca et al. 2010), was first detected in 1999 in the continental United States in Connecticut (Andreadis et al. 2001). Multiple independent introductions of this species are responsible for populations on the eastern Atlantic seaboard and in the Pacific Northwest and Hawaii (Fonseca et al. 2010). Aedes japonicus (Figure 8) is currently only found in Florida's Panhandle counties (Riles et al. 2017; Smith et al. 2020). Artificial containers, such as rainwater barrels and discarded tires, are commonly occupied by this species where rock holes containing water are not available.
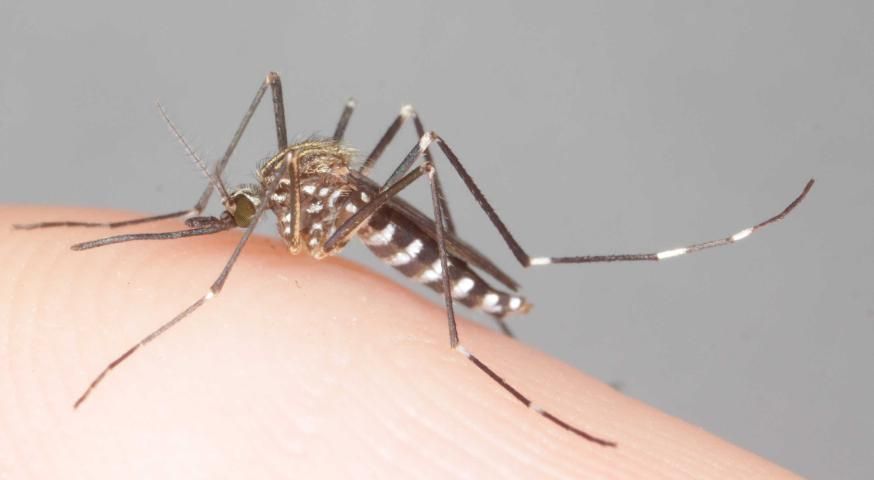
Credit: N. D. Burkett-Cadena, UF/IFAS
6. Aedes triseriatus Say (Eastern tree hole mosquito)
In the Midwest and mid-Atlantic United States, A. triseriatus (Figure 9) is the traditional vector of La Crosse encephalitis virus (LACV), which may cause life-threatening neurological damage in humans. While the majority of LACV disease cases occur in the upper Midwestern, mid-Atlantic, and southeastern states, two LACV neuroinvasive disease cases were reported in Florida from 2010 to 2019. Ae. triseriatus was the most abundant Aedes found in Florida treeholes until A. albopictus arrived and spread in the late 1980s and early 1990s. It is also common throughout the state in discarded tires, especially in wooded areas.
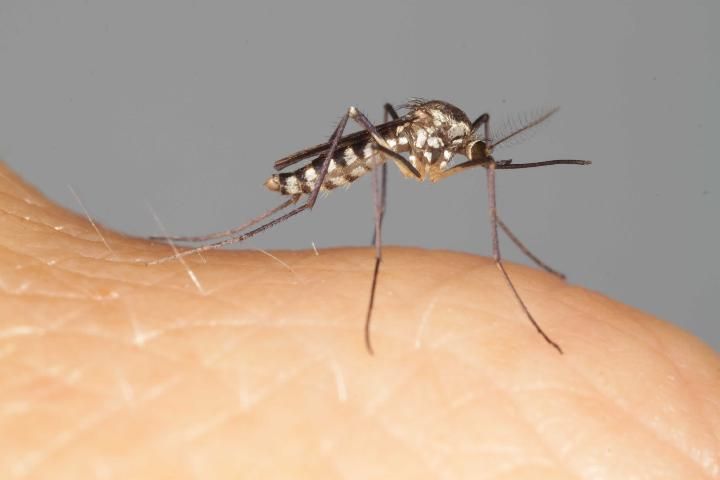
Credit: N. D. Burkett-Cadena, UF/IFAS
7. Anopheles barberi Coquillett (Barber's tree hole Anopheles)
One of only a few species of this genus known to develop in treeholes in hardwood forests of northern Florida, A. barberi (Figure 10) occupies a distinctive subset of treeholes that are large, drought resistant, and found lower down in larger trees, and that contain dark water with high conductivity and pH levels (Bradshaw and Holzapfel 1983, 1988). Like several other treehole mosquitoes native to the United States, A. barberi overwinters in its larval stages (Bradshaw and Holzapfel 1984) and is known to consume other small aquatic animals, including small mosquito larvae (Nannini and Juliano 1998).
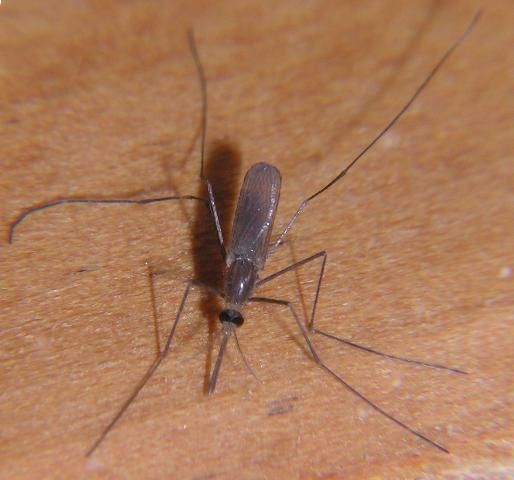
Credit: N. D. Burkett-Cadena, UF/IFAS
8. Culex biscaynensis Zavortink & O'Meara (South Florida bromeliad Culex)
Until recently, this species was unknown to science. After its discovery by O'Meara and Evans (1997) in bromeliad axils in southern Miami-Dade County, C. biscayensis (Figure 11) was described as a new species (Zavortink and O'Meara 1999). To date it has been collected only from Miami-Dade County, and very little is known about its biology and epidemiological importance.
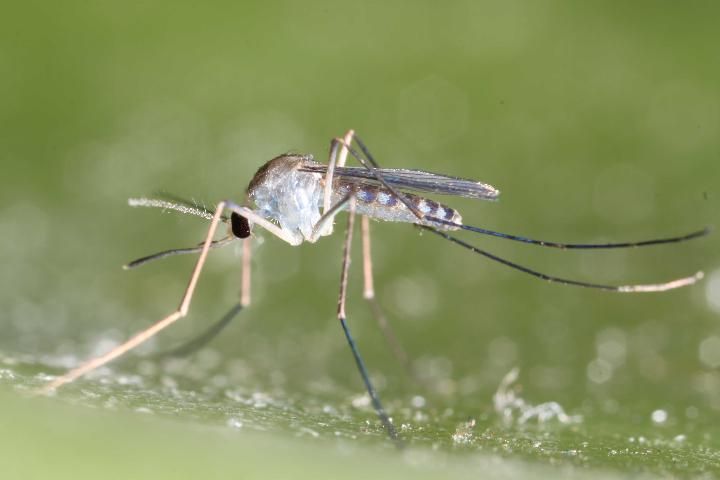
Credit: N. D. Burkett-Cadena, UF/IFAS
9. Orthopodomyia signifera (Coq.) and Orthopodomyia alba (ornate treehole mosquitoes)
These two sibling species may co-occur, even in the same treehole, in the midwestern United States (Copeland and Craig 1990). In northern Florida, O. signifera (Figure 12) commonly occurs in the same treehole with the larval predator Toxorhynchites rutilus (see below) but escapes predation by quiet filter-feeding that does not arouse prey-detection cues used by this predator (Bradshaw and Holzapfel 1983). Although O. alba is included in keys to the mosquitoes of Florida (Darsie and Morris 2000) and was recorded as having been collected in Bay County, Florida, by Jenkins and Carpenter (1946), the adult female of this species is indistinguishable from that of O. signifera, and the claim that O. alba may be present in Florida warrants confirmation.
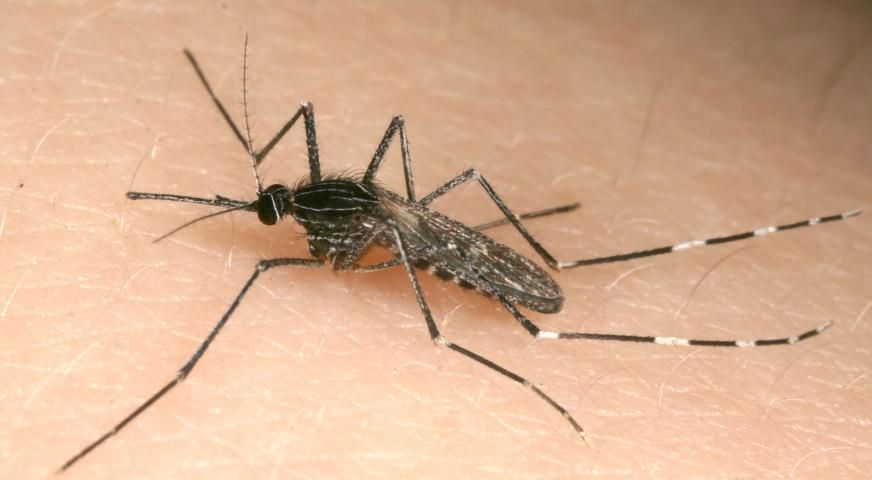
Credit: N. D. Burkett-Cadena, UF/IFAS
10. Toxorhynchites rutilus (Coq.) (Non-biting cannibal or elephant mosquito)
As very large carnivorous predators in Florida treeholes, larvae of T. rutilus (Figure 13) regulate the structure of the aquatic mosquito community (Bradshaw and Holzapfel 1983) and significantly reduce the abundance of some other mosquito prey species, such as A. triseriatus (Lounibos et al. 1997; Bradshaw and Holzapfel 1983). While T. rutilus may suffer local extinctions during droughts (Lounibos et al. 1997; Bradshaw and Holzapfel 1988), adults do not take blood, and larvae of this genus have been deployed as biological control agents, e.g., in New Orleans, to reduce abundances of A. aegypti (Focks 2007).
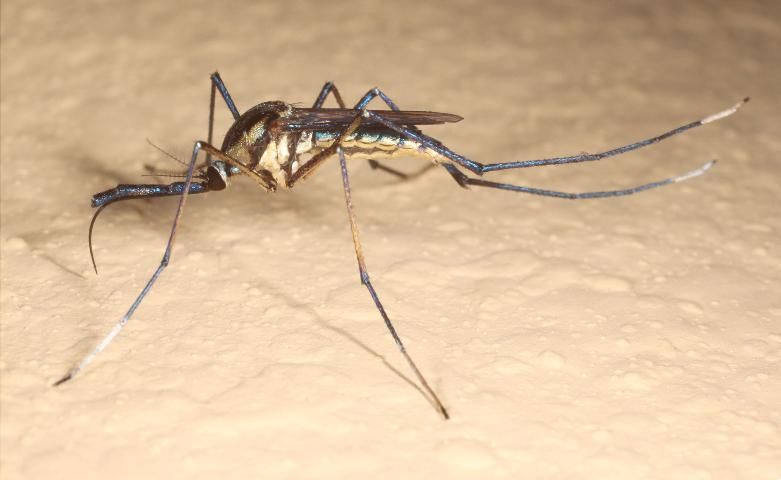
Credit: N. D. Burkett-Cadena, UF/IFAS
11. Wyeomyia mitchellii (Theobald) (Florida bromeliad Wyeomyia)
This is one of two sister species native to Florida that occupy bromeliad phytotelmata, such as the native air plants (Figure 1B), as well as the many exotic species of bromeliads (Figure 1A) planted as ornamentals in gardens and parks. Florida Wyeomyia spp. are not known to vector any pathogens, but the two sister species can be daytime biting pests where exotic bromeliads proliferate in suburban gardens (Frank 1985). In addition to Florida, W. mitchellii (Figure 14) is now thriving in Hawaii, having hitchhiked across the Pacific and established in bromeliads planted in gardens (Shroyer 1981).
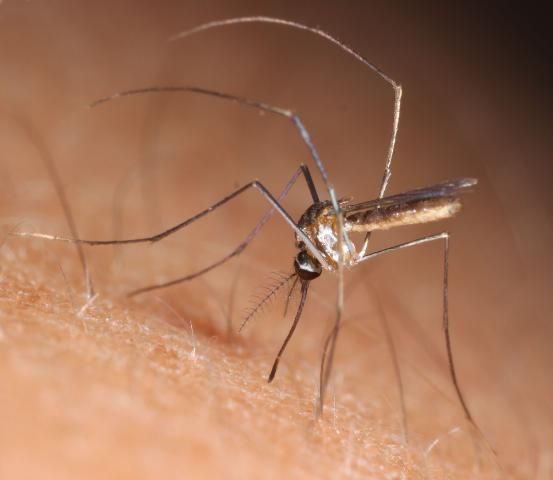
Credit: N. D. Burkett-Cadena, UF/IFAS
12. Wyeomyia smithii (Coq.) (North American pitcher plant mosquito)
This species is only found within the range of its obligate host, the purple pitcher plant, Sarracenia purpurea, from northern Florida northward to Newfoundland and Labrador, and westward to Alberta. On the Gulf Coast where multiple Sarracenia spp. co-occur, the immature stages of W. smithii (Figure 15) may be found in hybrids between S. purpurea and Sarracenia flava or Sarracenia leucophylla. In the northern United States, this species does not take blood, but populations from Florida do (O'Meara et al. 1981).
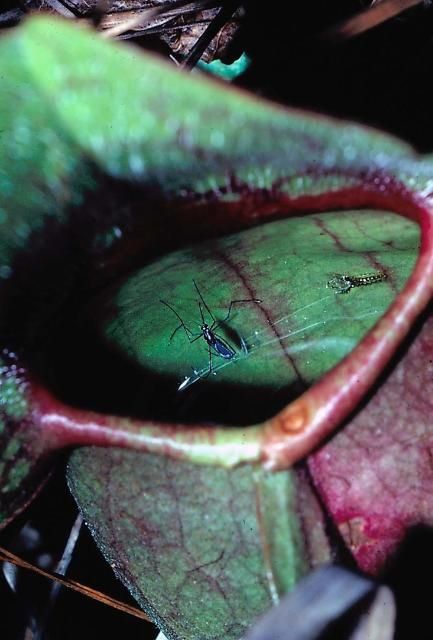
Credit: L. P. Lounibos, UF/IFAS
13. Wyeomyia vanduzeei Dyar & Knab (Florida bromeliad Wyeomyia sister species)
Like its sister species W. mitchellii, immature W. vanduzeei can be found in bromeliads native to Florida as well as in the water-filled tanks and leaf axils of exotic ornamental bromeliads that have been imported into Florida. The range of this species on the Florida peninsula is similar to that of its sister species W. mitchellii, but the preferred habitats of the two species differ in sun/shade exposure, with W. vanduzeei (Figure 16) being more prevalent in bromeliads exposed to more sun compared to W. mitchellii (Frank and O'Meara 1985).
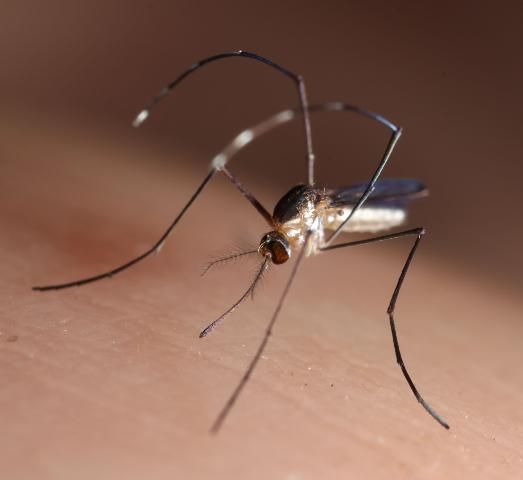
Credit: N. D. Burkett-Cadena, UF/IFAS
Occasional Mosquito Occupants of Containers
Anopheles crucians (a complex of 7 or more closely related species), Culex coronator, Culex nigripalpus, Culex restuans, Culex quinquefasciatus (southern house mosquito), and Deinocerites cancer (North American crabhole mosquito) are examples of mosquito species that may be found, on occasion, in containers or treeholes, but each of these species is found in greater relative abundance in its preferred habitat, e.g., D. cancer in crab holes or C. quinquefasciatus in eutrophic wastewaters. Notably, the mosquito species listed above that occasionally invade container habitats include one species known to vector the pathogen that causes malaria in humans (A. crucians) and others that transmit multiple arboviruses capable of causing human disease (C. coronator, C. nigripalpus, and C. quinquefasciatus).
Control
In this section, we discuss potential container mosquito and disease control methods. We distinguish between control methods that can be used by individuals, e.g., homeowners, versus large-scale control methods that can be used by governmental or non-governmental organizations potentially requiring scientific oversight and community participation.
Source Reduction
This term is used in mosquito control to refer to restricting the number or quality of habitats suitable for growth of immature mosquito life stages, i.e., eggs, larvae, and pupae. Source reduction and other control methods that target aquatic, immature mosquito life stages should be the first considered, because immature mosquito habitats are easier to access and treat compared to flying adult mosquitoes. When applied to container mosquitoes, source reduction advocates eliminating larval habitats by overturning water-holding containers or preventing their filling with rain or irrigation waters that produce suitable immature mosquito habitats. Although phytotelmata are generally less amenable to source reduction than artificial containers, purging standing water from bromeliad tanks with a hose jet has been suggested for larval mosquito control (Frank 1985). While source reduction may be challenging to sustain (Faraji and Unlu 2016), especially in the tropics, where homeowners might rely on the same stored water in containers for cooking, drinking, washing, etc., it is the most important technique that homeowners can employ to reduce mosquitoes. Homeowners who conduct source reduction can potentially decrease pesticide use and reduce the risk of contracting mosquito-borne diseases. More information about source reduction can be found here: Mosquitoes and their Control: Integrated Pest Management for Mosquito Reduction around Homes and Neighborhoods.
Biological and Biorational Control
Although many container habitats that harbor mosquitoes, especially those created by humans, contain simplified aquatic food webs in which predators are uncommon, artificial augmentation of predators by classical biological control methods can reduce abundances of container mosquito pests or disease vectors. For example, the rearing and release of non-biting adults of the larval predator Toxorhynchites spp. were used successfully to control A. aegypti in New Orleans in the 1980s (Focks 2007). The most successful use of predators for the biological control of container mosquitoes, as well as disease control, was achieved in rural villages in North Vietnam, where predatory copepods (small aquatic crustaceans) from local ponds were cultivated en masse, then released into the domestic water jars that produced the majority of A. aegypti in these villages (Kay et al. 2001). The reduction in vector numbers and elimination of dengue outbreaks were facilitated by community participation, both in rearing the copepods and accepting their maintenance in water storage vessels inside houses.
The term "biorational" was coined to describe natural products, or their analogs, that may function as chemical insecticides and that are thought to pose less risk to non-target organisms and food chains than synthesized chemical alternatives (Floore 2007). Examples of biorational products include derivatives of the insect juvenile hormone (JH) like methoprene and pyriproxyfen (PPF). These insect growth regulators interrupt mosquito larval development with minor non-target effects.
Gram-positive bacteria of the genus Bacillus were isolated and recognized for their toxicity to certain insects, such as Bacillus thuringiensis (BT) used to control caterpillars in agriculture. A strain of BT isolated from soil in Israel and subsequently designated Bacillus thuringiensis subspecies israelensis (BTI) was found to be specifically toxic to aquatic Diptera, such as mosquito and blackfly larvae (Margalit and Dean 1985). Currently in broad use as a biorational larvicide, the proteinaceous toxins of BTI are extracted and crystallized for delivery into the aquatic environment. Other related bacteria, notably Bacillus sphaericus, are cultured and delivered alive for control in larval mosquito habitats, especially in economically developing regions (Lacey 2007). Methoprene and BTI have in the United States largely supplanted the previously favored synthetic chemical larvicide Temephos (Abate) that was formerly certified as safe for use in domestic water containers (WHO 2009) but that is no longer approved by the EPA for sale in the United States. Biorational products containing the active ingredients BTI or methoprene can be added around homes to container mosquito habitats that cannot be eliminated using source reduction to kill developing mosquitoes.
While the biological and biorational control methods discussed above can reduce container mosquito populations, they require being able to detect and access container habitats for treatment. However, some immature container mosquitoes, particularly yellow fever and Asian tiger mosquitoes, can develop in small, hidden vessels, which makes detection and treatment of larval habitats difficult using conventional methods. The rest of this publication will discuss novel tools that may be used for controlling container mosquitoes. Owing to the scale and sophistication of these methods, their implementation potentially requires governmental or non-governmental organization(s) and community participation and scientific support.
Autocidal Gravid Trap
The US Centers for Disease Control and Prevention Dengue Branch (CDC-DB) recently developed the Autocidal Gravid Ovitrap (AGO trap), an inexpensive tool for reducing A. aegypti populations. An AGO trap is designed to attract gravid (pregnant) A. aegypti females looking for a site to lay their eggs. As females try to lay eggs inside the trap, they get stuck to sticky paper and die (Mackay et al. 2013). In communities in Puerto Rico where AGO traps were deployed, the density of female A. aegypti mosquitoes was reduced by >80% (Barrera et al. 2014). Also, the prevalence of chikungunya virus infection among residents in communities with AGO traps was lower compared to communities without AGO traps (Sharp et al. 2019).
Autodissemination
Yellow fever and Asian tiger female mosquitoes do not lay all of their eggs in one container and instead "skip oviposit," meaning that they visit and lay eggs in multiple containers (Colton et al. 2003). Autodissemination is a novel method of controlling skip-ovipositing mosquitoes that puts the mosquitoes themselves to work by capitalizing on the ability of females to find containers. Autodissemination traps or stations are designed to attract female skip-ovipositing mosquitoes that are ready to lay eggs and contaminate them with an insect growth regulator such as PPF. Then, as the skip-ovipositing females visit multiple containers, PPF is deposited in each one, preventing the emergence of adult mosquitoes from those containers (Caputo et al. 2012; Gaugler et al. 2012). Large-scale field trials have shown that autodissemination of PPF can reduce the density of skip-ovipositing adult mosquitoes (e.g., Abad-Franch et al. 2015; Buckner et al. 2021).
Releases of Altered Adult Mosquitoes
This subsection discusses a variety of techniques, some still in experimental phases of development, that release modified adult mosquitoes into environments where their mating with resident vector species, such as A. aegypti, may alter or suppress the targeted, resident species population (Ritchie 2014).
Mosquito Population Suppression Techniques
-
Sterilization by releasing irradiated males: This method relies on inundative releases of male mosquitoes that produce inviable sperm attributable to brief exposures to ionizing or chemical radiation. Although this technique has been used successfully to suppress various species of agricultural pests, it has never been satisfactorily deployed for mosquito control, owing to decreased fitness of released males and/or the rapid recoveries of temporarily sterilized wild populations in experimental trials.
-
Genetically modified mosquitoes (GMO) with fatal genetic flaws: Releases of A. aegypti genetically engineered to express a lethal mutation after mating with resident mosquitoes of the same species have been deployed in several tropical countries, especially Brazil, to suppress dengue and Zika virus vectors. Sustained releases of transgenic (containing genes from another species) A. aegypti reduced local A. aegypti by >90% in one Brazilian field study (Carvalho et al. 2015). The Florida Keys Mosquito Control District in collaboration with the British firm Oxitec are scheduled in 2021 to conduct the first field evaluation of the ability of transgenic A. aegypti to reduce local A. aegypti populations in the United States.
-
Mating incompatibilities through Wolbachia: A third method of suppression relies on introduction of Wolbachia spp., intracellular bacteria, in released mosquitoes. Wolbachia cause hybrid sterility in certain crosses with wild populations. This technique, which successfully suppressed the tropical vector C. quinquefasciatus (=fatigans) in Burma in the 1950s (Laven 1967), is now being promoted by the US firm MosquitoMate, Inc. and others against A. aegypti and A. albopictus. Releases of over 14 million Wolbachia-infected sterile A. aegypti males led to a 95.5% reduction in the number of female mosquitoes in release areas compared to non-release areas in a 2018 field trial in Fresno County, California (Crawford et al. 2020).
It should be noted that the aforementioned strategies are designed to eradicate local mosquito populations during their treatment (release) phases, but rebounds of these populations are to be expected over time, through immigration or unchecked growth of survivors that were not originally eradicated.
Mosquito Population Modification Techniques
An unexpected outcome of the transfer of Wolbachia from fruit flies to A. aegypti was the creation of a strain A. aegypti resistant to development and transmission of the common, disease-causing arboviruses, such as Zika, dengue, and yellow fever (Walker et al. 2011). This discovery led to the formation of the Eliminate Dengue Program (now known as the World Mosquito Program), which successfully introduced the Wolbachia-carrying A. aegypti to mate with resident mosquitoes of that same species in northern Australia, where dengue virus transmitted by that species had formerly been common (O'Neill et al. 2019). The introduction and spread of the virus-resistance into local A. aegypti has since been exported to multiple tropical countries, such as Colombia, Brazil, and Indonesia, by the World Mosquito Program (WMP).
While the WMP has already proven its capacity to modify resident populations of A. aegypti to reduce risks of arbovirus transmission, new gene drive tools, such as CRISPR-cas9, are currently being tested on caged populations of major vector mosquito species to rapidly drive modifications, such as resistance to carrying human parasites or pathogens, to genetic fixation (100% frequency of gene in population). However, owing to the novelty of these gene drive techniques and concerns about potential unexpected consequences, none have yet been approved for experimental trials in nature.
References
Abad-Franch, F., E. Zamora-Perea, G. Ferraz, S. D. Padilla-Torres, and S. L. B. Luz. 2015. "Mosquito-Disseminated Pyriproxyfen Yields High Breeding-Site Coverage and Boosts Juvenile Mosquito Mortality at the Neighborhood Scale." PLoS. Negl. Trop. Dis. 9:e0003702. https://doi.org/10.1371/journal.pntd.0003702
Andreadis, T. G., J. F. Anderson, L. E. Munstermann, R. J. Wolfe, and D. A. Florin. 2001. "Discovery, Distribution, and Abundance of the Newly Introduced Mosquito, Ochlerotatus japonicus (Diptera: Culicidae) in Connecticut, USA." J. Med. Entomol. 38:774–779. https://doi.org/10.1603/0022-2585-38.6.774
Barrera, R., M. Amador, V. Acevedo, R. R. Hemm, and G. Felix. 2014. "Sustained, Area-Wide Control of Aedes aegypti Using CDC Autocidal Gravid Ovitraps. Am. J. Trop. Med. Hyg. 91:1269–76. https://doi.org/10.4269/ajtmh.14-0426
Bates, M. 1949. The Natural History of Mosquitoes. NY: MacMillan Co. 378pp + xii.
Bradshaw, W. E., and C. M. Holzapfel. 1983. "Predator-Mediated, Non-Equilibrium Coexistence of Tree-Hole Mosquitoes in Southeastern North America." Oecologia 57:239–256. https://doi.org/10.1007/BF00379586
Bradshaw, W. E., and C. M. Holzapfel. 1984. "Seasonal Development of Tree-Hole Mosquitoes (Diptera: Culicidae) and Chaoborids in Relation to Weather and Predation." J. Med. Entomol. 21:366–378. https://doi.org/10.1093/jmedent/21.4.366
Bradshaw, W. E., and C. M. Holzapfel. 1988. "Drought and the Organization of Tree-Hole Mosquito Communities." Oecologia 74:507–514. https://doi.org/10.1007/BF00380047
Braks, M. A. H., N. A. Honorio, R. Lourenco-de-Oliveira, S. A. Juliano, and L. P. Lounibos. 2003. "Convergent Habitat Segregation of Aedes aegypti and Aedes albopictus (Diptera: Culicidae) in Southeastern Brazil and Florida." J. Med. Entomol. 40:785–794. https://doi.org/10.1603/0022-2585-40.6.785
Buckner, E. A., K. F. Williams, S. Ramirez, C. Darrisaw, J. Carrillo, M. D. Latham, and C. R. Lesser. 2021. "A Large-Scale Field Evaluation of the Effectiveness of In2Care® Mosquito Traps at Reducing Aedes aegypti (L.) Mosquitoes." PLoS. Negl. Trop. Dis. Manuscript in preparation.
Burkett-Cadena, N. D. 2013. Mosquitoes of the Southeastern United States. Tuscaloosa, AL: University of Alabama Press. 188 pp. + xiii
Burkett-Cadena, N., and T. R. Unnasch. 2014. "Conspiring Invasive Species? The Case of Tiger Mosquitoes and Apple Snails." Wing Beats 25:4–9.
Caputo, B., A. Ienco, D. Cianci, M. Pombi, V. Petrarca, A. Baseggio, G. J. Devine, and A. della Torre. 2012. "The 'auto-dissemination' approach: A novel concept to fight Aedes albopictus in urban areas." PLoS. Negl. Trop. Dis. 6:e1793. https://doi.org/10.1371/journal.pntd.0001793
Carvalho, D. O., A. R. McKemey, L. Garziera, R. Lacroix, C. A. Donnelly, L. Alphey, A. Malavasi, and M. L. Capurro. 2015. "Suppression of a Field Population of Aedes aegypti in Brazil by Sustained Release of Transgenic Male Mosquitoes." PLoS. Negl. Trop. Dis. 9 (7): e0003864. https://doi.org/10.1371/journal.pntd.0003864
Colton, Y. M., D. D. Chadee, and D. W. Severson. 2003. "Natural Skip Oviposition of the Mosquito Aedes aegypti Indicated by Codominant Genetic Markers." Med. Vet. Entomol. 17:195–204. https://doi.org/10.1046/j.1365-2915.2003.00424.x
Copeland, R. S., and G. B. Craig. 1990. "Habitat Segregation among Treehole Mosquitoes (Diptera: Culicidae) in the Great Lakes Region of the United States." Ann. Entomol. Soc. Am. 83:1063–1073. https://doi.org/10.1093/aesa/83.6.1063
Crawford, J. E., D. W. Clarke, V. Criswell et al. 2020. "Efficient Production of Male Wolbachia-Infected Aedes aegypti Mosquitoes Enables Large-Scale Suppression of Wild Populations." Nat. Biotechnol. 38:482–492. https://doi.org/10.1038/s41587-020-0471-x
Darsie, R. F., and C. D. Morris. 2000. "Keys to the Adult Females and Fourth Instar Larvae of the Mosquitoes of Florida (Diptera, Culicidae)." Tech. Bull. Fla. Mosq. Cont. Assoc., Vol. 1, 159pp (revised 2003).
Faraji, A., and I. Unlu. 2016. "The Eye of the Tiger, the Thrill of the Fight: Effective Larval and Adult Control Measures against the Asian Tiger Mosquito, Aedes albopictus (Diptera: Culicidae) in North America." J. Med. Entomol. 53:1029–1047. https://doi.org/10.1093/jme/tjw096
Floore, T. G. (ed.). 2007. "Biorational Control of Mosquitoes." Bull. No. 7, Am. Mosq. Cont. Assoc., published as Suppl. 2, J. Am. Mosq. Cont. Assoc. 23.
Focks, D. A. 2007. "Toxorhynchites as Biocontrol Agents." J. Am. Mosq. Cont. Assoc. (Suppl.) 23:118–127. https://doi.org/10.2987/8756-971X(2007)23[118:TABA]2.0.CO;2
Fonseca, D. M., A. K. Widdel, M. Hutchinson, S. E. Spichiger, and L. D. Kramer. 2010. "Fine-Scale Spatial and Temporal Population Genetics of Aedes japonicus, a New US Mosquito, Reveal Multiple Introductions." Molec. Ecol. 19:1559–1572. https://doi.org/10.1111/j.1365-294X.2010.04576.x
Frank, J. H. 1985. "Mosquito Production from Bromeliads in Florida." Florida Agric. Exp. Sta. Bull. No 877.
Frank, J. H., and G. F. O'Meara. 1985. "Influence of Micro- and Macrohabitat on Distribution of Some Bromeliad-Inhabiting Mosquitoes." Entomol. Exp. Appl. 37:169–174. https://doi.org/10.1111/j.1570-7458.1985.tb03469.x
Gaugler, R., D. Suman, and Y. Wang. 2012. "An Autodissemination Station for the Transfer of an Insect Growth Regulator to Mosquito Oviposition Sites." Med. Vet. Entomol. 26:37–45. https://doi.org/10.1111/j.1365-2915.2011.00970.x
Hribar, L. J., and M. E. Whiteside. 2010. "Seasonal Habitat Use by Immature Aedes aegypti (Linneaus) (Diptera: Culicidae) in the Florida Keys, USA." Stud. Dipt. 17:237–251.
Jenkins, D. W., and S. J. Carpenter. 1946. Ecology of the Treehole Breeding Mosquitoes of Nearctic North America." Ecol. Mgr. 16:31–47. https://doi.org/10.2307/1943573
Juliano, S. A., and L. P. Lounibos. 2005. "Ecology of Invasive Mosquitoes: Effects on Resident Species and on Human Health." Ecol. Lett. 8:558–574. 10.1111/j.1461-0248.2005.00755.x
Kay, B. H., V. S. Nam, T. V. Phong, T. Y. Nyugen, V. P. Tran, and T. B. D. Vu. 2001. "Control of Aedes Vectors of Dengue in Three Provinces of Vietnam by Use of Mesocyclops (Copepoda) and Community-Based Methods Validated by Entomologic, Clinical, and Serological Surveillance." 66:40–48. https://doi.org/10.4269/ajtmh.2002.66.40
Lacey, L. 2007. "Bacillus thuringiensis serovariety israelensis and Bacillus sphaericus for Mosquito Control." J. Am. Mosq. Cont. Assoc. (Suppl.2) 23:133–163. https://doi.org/10.4269/ajtmh.2002.66.40
Laird, M. 1988. The Natural History of Larval Mosquito Habitats. NY: Academic Press. 555pp. + xiv.
Laven, H. 1967. "Eradication of Culex pipiens fatigans through Cytoplasmic Incompatibility." Nature 216:383–384. https://doi.org/10.1038/216383a0
Lounibos, L. P. 2002. "Invasions by Insect Vectors of Human Disease." Ann. Rev. Entomol. 47:233–266. https://doi.org/10.1146/annurev.ento.47.091201.145206
Lounibos, L. P., R. L. Escher, N. Nishimura, and S. A. Juliano. 1997. "Long-Term Dynamics of a Predator Used for Biological Control and Decoupling from Mosquito Prey in a Subtropical Treehole Ecosystem." Oecologia 111:189–200. https://doi.org/10.1007/s004420050225
Lounibos, L. P., and S. A. Juliano. 2018. "Where Vectors Collide: The Importance of Mechanisms Shaping the Realized Niche for Modeling Ranges of Invasive Aedes Mosquitoes." Biol. Invas. 20:1913–1929. https://doi.org/10.1007/s10530-018-1674-7
Mackay, A. J., M. Amador, and R. Barrera. 2013. "An Improved Autocidal Gravid Ovitrap for the Control and Surveillance of Aedes aegypti." Parasit. Vectors 6:225. https://doi.org/10.1186/1756-3305-6-225
Margalit, J,, and D. Dean. 1985. "The Story of Bacillus thuringiensis var. israelensis (Bti)." J Am. Mosq. Cont. Assoc. 1:1–7.
Nannini, M. A., and S. A. Juliano. 1998. "Effects of the Facultative Predator Anopheles barberi on Population Performance of Aedes triseriatus." Ann. Entomol. Soc. Am. 91:33–42. https://doi.org/10.1093/aesa/91.1.33
O'Meara, G. F., and L. F. Evans. 1997. "Discovery of a Bromeliad-Inhabiting Culex (Micraedes) sp. in South Florida." J. Am. Mosq. Cont. Assoc. 13:208–210.
O'Meara, G. F., V. L. Larson, and D. H. Mook. 1993. "Blood Feeding and Autogeny in the Peridomestic Mosquito Aedes bahamensis (Diptera: Culicidae)." J. Med. Entomol. 30:378–383. https://doi.org/10.1093/jmedent/30.2.378
O'Meara, G. F., V. L. Larson, D. H. Mook, and M. D. Latham. 1989. "Aedes bahamensis: Its Invasion of South Florida and Association with Aedes aegypti." J. Am. Mosq. Cont. Assoc. 4:1–5.
O'Meara, G. F., L. P. Lounibos, and R. A. Brust. 1981. "Repeated Egg Clutches without Blood in the Pitcher-Plant Mosquito." Ann. Entomol. Soc. Am. 74:68–72. https://doi.org/10.1093/aesa/74.1.68
O'Neill, S. A., P. A. Ryan, A. P. Turley et al. 2019. "Scaled Deployment of Wolbachia to Protect the Community from Dengue and Other Aedes Transmitted Arboviruses." Gates Open Res. 2:36. https://doi.org/10.12688/gatesopenres.12844.3
Pafume, B. A., E. G. Campos, D. B. Francy, E. L. Peyton, A. N. Davis, and M. Nelms. 1988. "Discovery of Aedes (Howardina) bahamensis in the United States." J. Am. Mosq. Cont. Assoc. 4:380.
Parker, C. A., D. Ramirez, and C. R. Connelly. 2019. "State-Wide Survey of Aedes aegypti and Aedes albopictus (Diptera: Culicidae) in Florida." J. Vect. Ecol. 44:210–215. https://doi.org/10.1111/jvec.12351
Riles, M. T., J. P. Smith, N. Burkett-Cadena, C. R. Connelly, G. W. Morse, and B. D. Byrd. 2017. "First Record of Aedes japonicus in Florida." J. Am. Mosq. Cont. Assoc. 33:340–344. https://doi.org/10.2987/17-6696.1
Ritchie, S. 2014. "Rear and Release: A New Paradigm for Dengue Control." Austral. Entomol. 53:363–367. https://doi.org/10.1111/aen.12127
Röhnert, U. 1950 "Wassererfüllte Baumhöhlen und ihre Besiedlung. Ein Beitrag zur fauna dendrolimnetica." Archv. Hydrobiol. 44:475–516.
Scholl, P. J., and G. R. DeFoliart. 1977. "Aedes triseriatus and Aedes hendersoni: Vertical and Temporal Distribution as Measured by Oviposition." Envir. Entomol. 6:355–358. https://doi.org/10.1093/ee/6.3.355
Sharp, T. M., O. Lorenzi, B. Torres-Velásquez, V. Acevedo, J. Pérez-Padilla, A. Rivera, J. Muñoz-Jordán, H. S. Margolis, S. H. Waterman, B. J. Biggerstaff, G. Paz-Bailey, and R. Barrera. 2019. "Autocidal Gravid Ovitraps Protect Humans from Chikungunya Virus Infection by Reducing Aedes aegypti Mosquito Populations." PLoS Negl. Trop. Dis. 13:e0007538. https://doi.org/10.1371/journal.pntd.0007538
Shroyer, D. A. 1981. "Establishment of Wyeomyia mitchellii on the Island of Oahu, Hawaii." Mosq. News 41:805–806.
Smith, J. P., T. J. Taylor, I. Cami, C. I. Adams, R. A. Tennant, E. J. Cope, J. Walsh, B. Nomann, C. Horn, F. Johnson, G. Hilson, C. Golden, G. O'Neal, J. Kozak, K. Armstrong, and L. Conyers. 2020. "Updated County Mosquito Species Records for Northwest Florida." J. Fla. Mosq. Cont. Assoc. 67:1–9.
Thienemann, A. 1932. "Die Tierwelt der Nepenthes-Kannen." Archv. Hydrobiol. (Suppl.) 3:1–54.
Walker, T., P. H. Johnson, L. A. Moreira et al. 2011. "The wMel Wolbachia Strain Blocks Dengue and Invades Caged Aedes aegypti Populations." Nature 476:450–453. https://doi.org/10.1038/nature10355
Wilkerson, R. C., Y.-M. Linton, D. M. Fonseca, T. R. Schultz, D. C. Price, and D. A. Strickman. 2016. "Making Mosquito Taxonomy Useful: A Stable Classification of Tribe Aedini That Balances Utility with Current Knowledge of Evolutionary Relationships." Plos One10 (7): e0133602. https://doi.org/10.1371/journal.pone.0133602
World Health Organization (WHO). 2009. "Temephos in Drinking-Water: Use for Vector Control in Drinking-Water Sources and Containers." WHO/HSE/WSH/09.01/1.
Zavortink, T. J., and J. N. Belkin. 1979. "Occurrence of Aedes hendersoni in Florida (Diptera, Culicidae)." Mosq. News 39:673.
Zavortink, T. J., and G. F. O'Meara. 1999. "A New Species of Culex (Micraedes) from Florida (Diptera, Culicidae)." J. Am. Mosq. Cont. Assoc. 15:263–270.