This publication is part of a series titled Contaminants in the Urban Environment. This series is intended to give State and local government officials, soil scientists, consulting engineers, Extension agents, and citizens (1) a basic understanding of the occurrence, toxic effects, and source of various contaminants in the environment and (2) guidance on ways to protect human and environmental health.
Introduction and Purpose
Pharmaceuticals are products that we ingest or give to our pets and domesticated animals to improve health and to prevent or treat human and animal diseases. Pharmaceuticals include over-the-counter drugs, prescription medicines, nutritional supplements, and veterinary drugs.
Personal care products are products used to improve the quality of daily life by adorning and cleaning our bodies. Personal care products include lotions, fragrances, shampoos, antibacterial soaps, detergents, sunscreens, insect repellents, and cosmetics.
Together, these products are known as pharmaceuticals and personal care products, abbreviated as PPCPs (Figure 1).
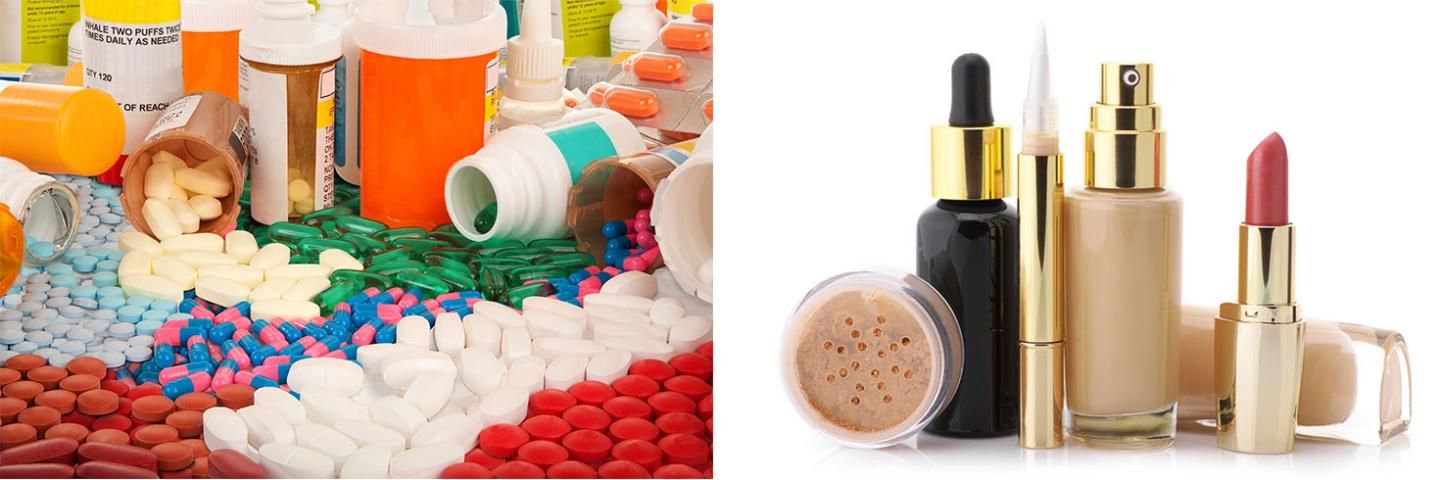
Credit: iStock/Thinkstock.com
Contaminants in the Urban Environment: Pharmaceuticals and Personal Care Products (PPCPs)—Part 1 provided an overview of PPCPs use and sale in the United States and the world (https://edis.ifas.ufl.edu/ss632). This publication, Contaminants in the Urban Environment: Pharmaceuticals and Personal Care Products (PPCPs)—Part 2, discusses the sources and impacts of PPCPs as well as the ways to protect our environment from PPCPs.
Sources of PPCPs in the Environment
PPCPs are released to the environment by multiple sources (Figure 2). Human contribution of PPCPs includes excretion in urine and feces and washing in baths and sinks inside the home. Other sources of PPCPs in the environment include residues from pharmaceutical manufacturing plants (which are well defined and controlled), residues from hospitals, illicit drug use, veterinary drug use (especially antibiotics and steroids), and agricultural activities.
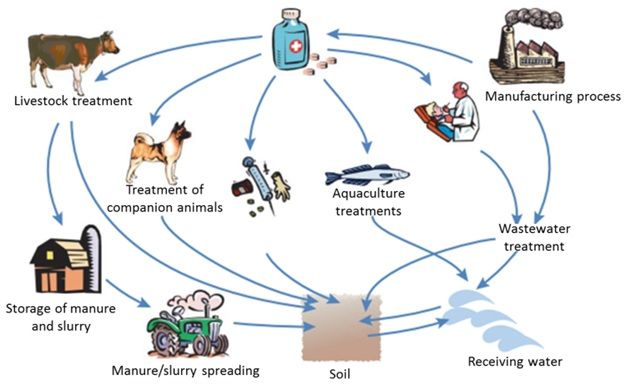
Credit: Boxall (2004)
Among all these sources, human contribution of PPCPs is the major source. Human PPCPs are generally absorbed by the body after intake and are then excreted and discharged into the sewage or septic systems (wastewater). When wastewater is either discharged directly into the environment (after undergoing proper treatment at a WWTP), used for irrigation (i.e., reclaimed water) or biosolids (i.e., properly treated sewage sludge) and then applied as a fertilizer to agricultural land, PPCPs can be released into the environment (Kinney et al. 2006).
Veterinary and agricultural pharmaceuticals can be released to the environment via animal excretion or during the land application of manure. The usage and subsequent release of pharmaceuticals into the environment from animal usage is particularly concerning as approximately 80% of all antiobiotic usage in the United States is for agriculture/aquaculture (Hollis and Ahmed 2013). Another potential but less recognized source of PPCPs in groundwater is septic or onsite wastewater treatment systems. Septic systems remain important sources of PPCP contamination of groundwater, particularly where groundwater tables are shallow, such as in Florida and in several coastal states. To learn more about chemical compounds in septic systems, consult the EDIS article, Onsite sewage treatment and disposal systems: Trace Organic Chemicals at: https://edis.ifas.ufl.edu/ss554.
Impact of PPCPs in the Environment
A number of studies have documented the presence of PPCPs such as antibiotic, anti-inflammatory, anti-epileptic, and recreational medicines in ground- and surface waters throughout the world (see summary of these studies in Tables 1 and 2). The six most commonly reported PPCPs globally in the groundwater (Table 1) are as follows (Lapworth et al. 2012):
- Caffeine (14 studies, average maximum concentration: 9,800 nanograms per liter [ng/L])
- Carbamazepine (23 studies, average maximum concentration: 5,000 ng/L)
- Bisphenol A (9 studies, average maximum concentration: 2,500 ng/L)
- Ibuprofen (14 studies, average maximum concentration: 1,500 ng/L)
- Sulfamethoxazole (15 studies, average maximum concentration: 252 ng/L)
- Diclofenac (11 studies, average maximum concentration: 121 ng/L)
These six compounds are also present in surface water, stream water, and finished drinking water (Table 2). Concentrations of PPCPs detected in surface waters near discharges of sewage effluents are as follows (Whitacre 2011):
- Caffeine (8 studies, minimum concentrations: 4,900 ng/L)
- Carbamazepine (9 studies, minimum–maximum concentrations: <1,000 to 7,100 ng/L)
- Ibuprofen (18 studies, minimum–maximum concentrations: non-detected (ND) to 1,200 ng/L)
- Sulfamethoxazole (10 studies, minimum–maximum concentrations: ND to 1,900 ng/L)
- Diclofenac (14 studies, minimum–maximum concentrations: ND to 5,040 ng/L)
Table 1 shows the frequency and summary statistics of PPCPs detected in regional and national scale groundwater reconnaissance studies. In these studies, the range of detection frequency was variable: sulfamethoxazole (10%–24%), ibuprofen (0%–7%), carbamazepine (1.2%–42%), bisphenol-A (8%–59%), and caffeine (13%–83%). The widespread occurrence of PPCPs in the environment could be due to their history of use and because they have had sufficient time to travel through the unsaturated soil zone (vadose zone) to the groundwater. In addition, properties of PPCPs can control the attenuation of PPCPs during wastewater treatment and the subsequent release of PPCPs into the environment. Even if PPCPs are partially or fully degraded in the environment or during treatment processes, the continual release of these contaminants into the environment has led to their being termed pseudopersistant (Ellis 2006).
A summary of PPCPs' occurrence and frequency in the United States waters is provided in Table 2. The main classes of PPCPs in US freshwaters were antibiotic, anti-inflammatory, anticonvulsant, sterol, flame retardant, plasticizer, and stimulant. Less information on PPCPs in the environment was found in the literature a few decades ago due to lack of knowledge and the analytical challenges of detecting PPCPs at trace levels. Note that these compounds might have existed for a long time since some of them (e.g., carbamazepine, sulfamethoxazole, and ibuprofen) have been approved for use in the United States for more than three decades. Due to the improvement in analytical methods in the last 15+ years, researchers now have the ability to measure very small concentrations at ng/L (parts per trillion).
Although environmental concentrations of PPCPs are low, the risks to human health and the environment cannot be excluded because they are still poorly understood. Research suggests that certain PPCPs (e.g., hormones) may cause reproductive and developmental harm in aquatic organisms even at trace levels (ng/L to sub-μg/L); these are the levels that are frequently observed in ground- and surface waters (Vajda et al. 2008). Furthermore, a wide range of studies have found that environmentally realistic concentrations of a variety of PPCPs can have sub-lethal ecological impacts (reviewed by Richmond et al. 2017).
Impacts on Aquatic Health: Most of the research on the effects of PPCPs involves antibiotics and steroid hormones. Even though these chemicals are present only at trace levels (part per trillion, or ng/L), PPCPs can cause changes in the behavior and biochemistry (i.e., functioning) in aquatic animals such as fish, frogs, and mussels. More notably, estrogens (or hormones)—such as estrone, estradiol, and ethinyl estradiol—have been observed to cause feminization of male fish at concentrations as low as a few ng/L (Jobling et al. 2003; Vajda et al. 2008). For example, exposure to 2–10 ng/L of 17α-ethinylestradiol (primarily used in hormonal contraceptives) induced synthesis of vitellogenin (an egg yolk protein precursor found only in females) in male zebrafish (Danio rerio), Japanese medaka (Oryzias latipes), and Chinese rare minnow (Gobiocypris rarus) (Ma et al. 2005; Xu et al. 2008; Zha et al. 2007). In a study of PPCP impacts on microbial communities, Rosi et al. (2018) found that cimetidine (an antacid and antihistamine) and ciprofloxacin (an antibiotic) both reduce microbial respiration in streams rarely exposed to these compounds, and that microbial community composition was drastically different for microbial communities exposed to ciprofloxacin.
In personal care products, triclosan is an antibacterial and antifungal agent present in products such as soaps, deodorants, and toothpastes. Triclosan and its degradation product, triclocarban, have been investigated mainly because of their relative high toxicity to aquatic organisms (Dussault et al. 2008; Ishibashi et al. 2004; Tamura et al. 2013). Triclosan and triclocarban were found to be toxic with the 50% effect concentration (dose at which 50% of the test population is killed) values from 4 to 35 μg/L (72-hour exposure ) for green algae, 7 to 230 μg/L (48-hour exposure) for daphnia, and 45 to 340 μg/L (96-hour exposure) for fish (Tamura et al. 2013). The marketing and development of triclosan-containing products was banned by the United States Food and Drug Administration in 2017.
Impacts on Human Health: Studies have shown that some PPCPs originating from human therapies are not completely eliminated in the sewage treatment systems and are discharged as contaminants into the receiving waters (Stackelberg et al. 2004; Ternes et al. 2002). There may be a potential risk of drinking water contamination by PPCPs, especially in urban areas with high sewage wastewater outputs and low surface-water flows.
Eighty percent of the antibiotics sold in the United States are used for animal agriculture (Hollis and Ahmed 2013). According to the Food and Drug Administration (FDA), farm animals ingest nearly 30 million pounds of antibiotics annually. Research has verified the occurrence of veterinary antibiotics in manure, agricultural fields, and surface water bodies (Kim et al. 2010; Pinheiro et al. 2013). Once PPCPs enter the soil, compounds with strong fixation and resistance to degradation remain in surface soils for a long time. These compounds then could be potentially taken up by plants (Holling et al. 2012; Sabourin et al. 2012) and leach or run off to ground- and surface waters.
A relation between use of antibiotics and the occurrence of antibiotic-resistance in bacterial isolates from manure or manure-contaminated surface water has been found (Heuer and Smalla 2007; Peak et al. 2007). Antibiotic-resistant bacteria—that is, the bacteria that cannot be controlled or killed by antibiotics—can cause serious disease and are an important public health issue. Antibiotic-resistant bacteria attach to crops or become endophytes (i.e., bacteria living within plants) that may lead to exposure to humans through food supply. For more information on antibiotic resistance and food safety, see the Centers for Disease Control and Prevention website (2014; http://www.cdc.gov/narms/faq.html).
Some chemicals and bacteria in food may represent hazards but at trace levels do not represent a human health risk, and research has yet to demonstrate an impact on human health from the trace levels at which PPCPs occur in the environment. However, according to the National Institute of Environmental Health Sciences (NIEHS), endocrine disruptors may pose the greatest risk during prenatal and early development when organ and neural systems are forming.
What do we Not know about PPCPs?
To date, the improved methods and progress in technology has made it possible to detect very low levels of PPCPs in the environment and their effects on aquatic and terrestrial organisms. However, there are still many questions that need to be addressed before we can eventually determine whether residues of PPCPs in the environment are a threat to human and environmental health.
First, how much of an impact do PPCPs have in the natural environment? For example, a related issue is whether we should worry about transformation products. There is sparse information on the formation of PPCP degradation products, the ecotoxicity of PPCP metabolites, and mixtures of PPCPs. In some instances, these degradation products may be more ecotoxic, more persistent, and more mobile than the parent compounds. Furthermore, many of the environmental impacts of PPCPs are sublethal. Although these sublethal effects may not directly kill organisms, changes in organismal behavior or function can have subsequent environmental impacts (Richmond et al. 2017). During risk assessment, an improved understanding of the sublethal effects and transformation of PPCPs would help identify the circumstances in which metabolites should receive closer attention.
Second, does environmental exposure to PPCP residues result in more antibacterial resistance? To help determine how important PPCP residues are in relation to public health, we would need a better understanding of (1) the role of environmental antibiotic residues in the selection of antibiotic resistance and (2) the potential for antibiotic resistances to transfer from the environment to human and animal pathogens.
Finally, what wastewater treatment methods are effective in reducing the effects of PPCPs in the environment? It may be necessary to develop advanced treatment systems that consider local conditions and constraints in order to remove PPCPs during the treatment processes. If the wastewater treatment methods were dramatically improved, then most of the PPCPs would never enter the environment. We suggest that this process should be a priority in our ongoing efforts to manage PPCPs, although this would require considerable amount of resources and infrastructure and the willingness of elected officials.
What can you do to protect the environment?
We all can use common-sense approaches to protect the environment. For example, do not flush unused or expired drugs down the drain. Proper disposal of drugs is a straightforward way for us to prevent pollution. The United States Environmental Protection Agency offers guidelines on proper disposal procedures for PPCPs, which can be access here: https://www.epa.gov/sites/production/files/2015-06/documents/how-to-dispose-medicines.pdf. Some recommendations for consumers (US EPA 2009) include the following:
- Keep drugs in the original container.
- Scratch out patient information on labels.
- Place liquids in glass bottles and then in plastic resealable bags to contain leakage.
- Add some water to dry tablets or capsules to dissolve them slightly.
- Place the items in the trash.
- Check to see if your pharmacy has a drug "take back" program.
- Try to purchase only the quantity of drugs that you will use.
- Cut down on the amount of PPCPs that you use.
- Follow any specific disposal instructions associated with your prescription.
For more information about proper disposal of prescription drugs, consult this website: http://www.fda.gov/ForConsumers/ConsumerUpdates/ucm101653.htm.
Other potential approaches to reduce the amount of PPCPs released to the environment include the following:
- Introduction of improved wastewater treatment options.
- Medicine labels that provide information on possible environmental impact, allowing consumers to consider environmental outcomes if the medication is not disposed of correctly.
- Learning more about these issues and sharing the message.
Appendix: Calculating the Amount of PPCPs in Wastewater
We provide this tentative calculation as a way to determine the levels of compounds in the environment, if the occurrence data are not widely available.
The amount of PPCPs in wastewater varies by the type of compound and by time of the year. The load of a pharmaceutical in wastewater can be estimated with a model based on the sale volume, wastewater production, disposal rate of unused medicines, excretion rate, and removal efficiency by the wastewater treatment plant (Zhang and Geißen 2010). For example, carbamazepine is an anticonvulsant and mood-stabilizing medicine used primarily in the treatment of seizure disorders and neuropathic pain. It is one of the most frequently detected pharmaceuticals in water bodies and is known to be persistent in the environment with removal efficiencies less than 20% (Oosterhuis, Sacher, and ter Laak 2013).
The example below demonstrates the amount of carbamazepine that may be excreted using the sale, wastewater production, excretion, and removal efficiency data from the literature.

Equation 1
Calculation of the human excretion of PPCPs, using Equation 1:
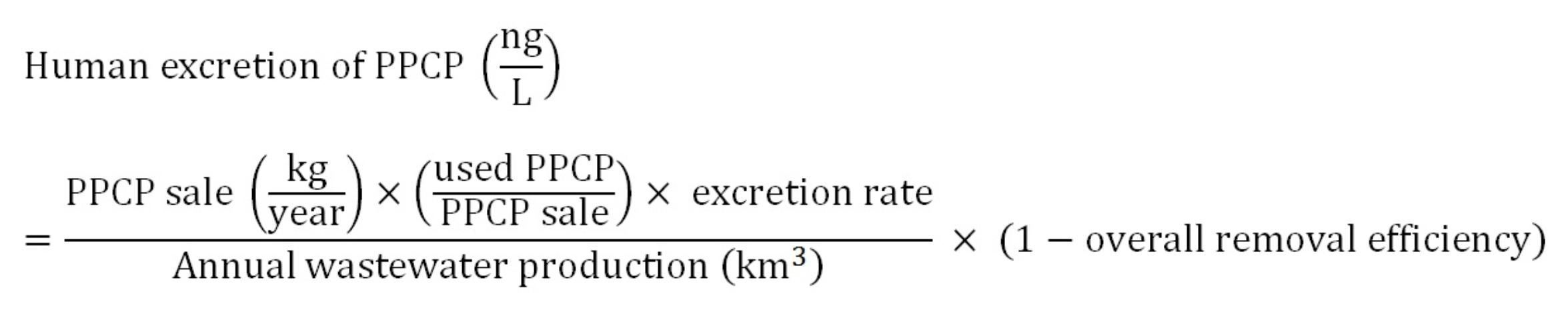
For example, sale volume of carbamazepine in 2007 was 124,556 kg in the United States. The annual wastewater production in 2007 was 61 km3 (Zhang and Geißen 2010). The ratio of used carbamazepine to the carbamazepine sale is assumed to be 0.67, as indicated in a survey (Bound and Voulvoulis 2005). Assuming that approximately 30% of carbamazepine is excreted in the original form (Lienert, Güdel, and Escher 2007; Zhang, Geißen, and Gal 2008); and thus the excretion rate of carbamazepine is set at 0.3. The overall removal rate of carbamazepine in sewage systems is set at 0.1 based on literature (10%–13% removal).
Substitution of these values in Equation 1:
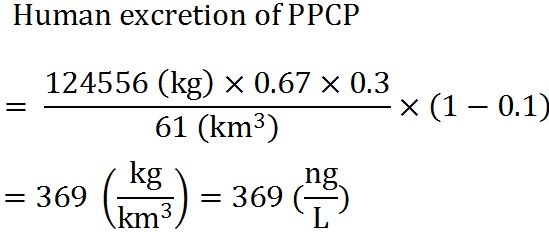
As 1 kg = 1012 ng and 1 km3 = 1012 L, this equation evaluates to 369 ng/L of carbamazepine in wastewater.
Equation 2
Calculation of the amount of PPCP that is directly disposed or flushed, using Equation 2:
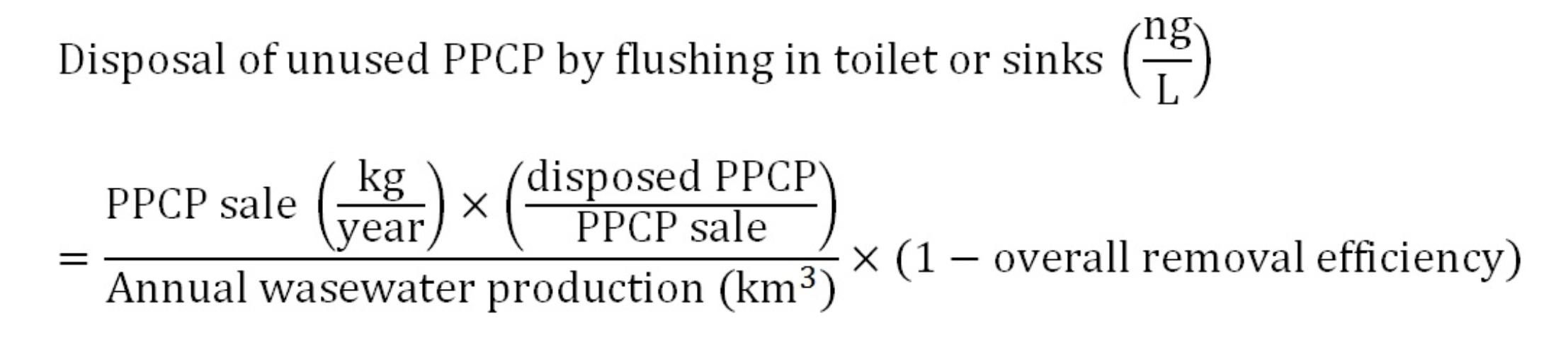
In this case, the ratio of disposing carbamazepine to the carbamazepine sale is set at 0.17, as indicated in a survey on disposal practices of unused medications (Bound and Voulvoulis 2005). All other parameters will remain the same as human excretion of PPCP (Equation 1).
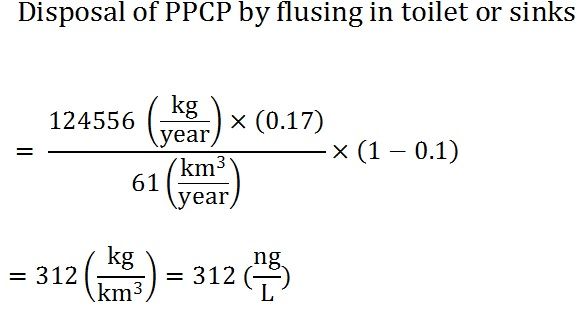
Thus, the predicted concentration of carbamazepine in the sewage effluent/wastewater will be the sum of human excretion (369 ng/L), as calculated by Equation 1, and direct disposal (312 ng/L), as calculated by Equation 2, resulting in total concentration of 681 ng/L of wastewater.
The concentrations of other PPCPs in the wastewater can be calculated using the above equations, if other data used in equations 1 and 2 are available. This may provide a way to calculate the likely concentrations of other PPCPs in the environment.
References
Barnes, K. K., D. W. Kolpin, E. T. Furlong, S. D. Zaugg, M. T. Meyer, and L. B. Barber. 2008. "A National Reconnaissance of Pharmaceuticals and Other Organic Wastewater Contaminants in the United States - I) Groundwater." Science of the Total Environment 402: 192–200.
Benotti, M. J., R. A. Trenholm, B. J. Vanderford, J. C. Holady, B. D. Stanford, and S. A. Snyder. 2009. "Pharmaceuticals and Endocrine Disrupting Compounds in US Drinking Water." Environmental Science and Technology 43: 597–603.
Bound, J. P., and N. Voulvoulis. 2005. "Household Disposal of Pharmaceuticals as a Pathway for Aquatic Contamination in the United Kingdom." Environmental Health Perspectives 113: 1705–1711.
Boxall, A. B. A. 2004. "The Environmental Side Effects of Medication." EMBO Rep 5: 1110–1116.
Center for Disease Control and Prevention. 2013. "Frequently Asked Questions about Antibiotic Resistance and Food Safety." Accessed February 10, 2014. http://www.cdc.gov/narms/faq.html.
Dussault, È. B., V. K., Balakrishnan, E. Sverko, K. R. Solomon, and P. K. Sibley. 2008. "Toxicity of Human Pharmaceuticals and Personal Care Products to Benthic Invertebrates." Environmental Toxicology and Chemistry 27: 425–432.
Ellis, J. B. 2006. "Pharmaceutical and personal care products (PPCPs) in urban receiving waters." Environmental Pollution 144: 184–189.
Focazio, M. J., D. W. Kolpin, K. K. Barnes, E. T. Furlong, M. T. Meyer, S. D. Zaugg, L. B. Barber, and M. E. Thurman. 2008. "A National Reconnaissance for Pharmaceuticals and Other Organic Wastewater Contaminants in the United States - II) Untreated Drinking Water Sources." Science of the Total Environment 402: 201–216.
Fram, M. S., and K. Belitz. 2011. "Occurrence and Concentrations of Pharmaceutical Compounds in Groundwater Used for Public Drinking-Water Supply in California." Science of the Total Environment 409: 3409–3417.
Heuer, H., and K. Smalla. 2007. "Manure and Sulfadiazine Synergistically Increased Bacterial Antibiotic Resistance in Soil Over at Least Two Months." Environmental Microbiology 9: 657–666.
Holling, C. S., J. L. Bailey, B. V. Heuvel, and C. A. Kinney. 2012. "Uptake of Human Pharmaceuticals and Personal Care Products by Cabbage (Brassica campestris) from Fortified and Biosolids-Amended Soils." Journal of Environmental Monitoring 14: 3029–3036.
Hollis, A., and Z. Ahmed. 2013. "Preserving antibiotics, rationally." New England Journal of Medicine 369: 2474–2476.
Ishibashi, H., N. Matsumura, M. Hirano, M. Matsuoka, H. Shiratsuchi, Y. Ishibashi, Y. Takao, and K. Arizono. 2004. "Effects of Triclosan on the Early Life Stages and Reproduction of Medaka Oryzias latipes and Induction of Hepatic Vitellogenin." Aquatic Toxicology 67: 167–179.
Jobling, S., D. Casey, T. Rodgers-Gray, J. Oehlmann, U. Schulte-Oehlmann, S. Pawlowski, T. Baunbeck, A. P. Turner, and C. R. Tyler. 2003. "Comparative Responses of Molluscs and Fish to Environmental Estrogens and an Estrogenic Effluent." Aquatic Toxicology 65: 205–220.
Kim, S. C., J. G. Davis, C. C. Truman, J. C. Ascough, and K. Carlson. 2010. "Simulated Rainfall Study for Transport of Veterinary Antibiotics—Mass Balance Analysis." Journal of Hazardous Materials 175: 836–843.
Kinney, C. A., E. T. Furlong, S. L. Werner, and J. D. Cahill. 2006. "Presence and Distribution of Wastewater-Derived Pharmaceuticals in Soil Irrigated with Reclaimed Water." Environmental Toxicology and Chemistry 25: 317–326.
Kolpin, D. W., E. T. Furlong, M. T. Meyer, E. M. Thurman, S. D. Zaugg, L. B. Barber, and H. T. Buxton. 2002. "Pharmaceuticals, Hormones, and Other Organic Wastewater Contaminants in U.S. Streams, 1999–2000: A National Reconnaissance." Environmental Science and Technology 36: 1202–1211.
Lapworth, D. J., N. Baran, M. E. Stuart, and R. S. Ward. 2012. "Emerging Organic Contaminants in Groundwater: A Review of Sources, Fate and Occurrence." Environmental Pollution 163: 287–303.
Lienert, J., K. Güdel, B. I. Escher. 2007. "Screening Method for Ecotoxicological Hazard Assessment of 42 Pharmaceuticals Considering Human Metabolism and Excretory Routes." Environmental Science and Technology 41: 4471–4478.
Ma, T., X. Wan, Q. Huang, Z. Wang, and J. Liu. 2005. "Biomarker Responses and Reproductive Toxicity of the Effluent from a Chinese Large Sewage Treatment Plant in Japanese medaka (Oryzias latipes)." Chemosphere 59: 281–288.
Miller, K. J., and J. Meek. 2006. "Helena Valley Ground Water: Pharmaceuticals, Personal Care Products, Endocrine Disruptors (PPCPs) and Microbial Indicators of Fecal Contamination." Montana Bureau of Mines and Geology, Open File Report 532.
National Center for Health Statistics. 2013. "Health, United States, 2012: With Special Feature on Emergency Care." Hyattsville, MD.
Oosterhuis, M., F. Sacher, and T. L. ter Laak. 2013. "Prediction of Concentration Levels of Metformin and Other High Consumption Pharmaceuticals in Wastewater and Regional Surface Water Based on Sales Data." Science of the Total Environment 442: 380–388.
Peak, N., C. W. Knapp, R. K. Yang, M. M. Hanfelt, M. S. Smith, D. S. Aga, and D. W. Graham. 2007. "Abundance of Six Tetracycline Resistance Genes in Wastewater Lagoons at Cattle Feedlots with Different Antibiotic use Strategies." Environmental Microbiology 9: 143–151.
Pinheiro, A., R. M. Rosa Albano, T. C. Alves, V. Kaufmann, and M. R. da Silva. 2013. "Veterinary Antibiotics and Hormones in Water from Application of Pig Slurry to Soil." Agricultural Water Management 129: 1–8.
Richmond, E. K., M. R. Grace, J. J. Kelly, A. J. Reisinger, E. J. Rosi, and D. M. Walters. 2017. "Pharmaceuticals and personal care products (PPCPs) are ecological disrupting compounds (EcoDC)." Elementa: Science of the Anthropocene 5: 66.
Rosi, E. J., H. A. Bechtold, D. Snow, M. Rojas, A. J. Reisinger, and J. J. Kelly. 2018. "Urban stream microbial communities show resistance to pharmaceutical exposure." Ecosphere 9: e02041.
Sabourin, L., P. Duenk, S. Bonte-Gelok, M. Payne, D. R. Lapen, and E. Topp. 2012. "Uptake of Pharmaceuticals, Hormones and Parabens into Vegetables Grown in Soil Fertilized with Municipal Biosolids." Science of the Total Environment 431: 233–236.
Stackelberg, P. E., E. T. Furlong, M. T. Meyer, S. D. Zaugg, A. K. Henderson, and D. B. Reissman. 2004. "Persistence of Pharmaceutical Compounds and Other Organic Wastewater Contaminants in A Conventional Drinking-Water Treatment Plant." Science of the Total Environment 329: 99–113.
Tamura, I., K. Kagota, Y. Yasuda, S. Yoneda, J. Morita, N. Nakada, Y. Kameda, K. Kimura, N. Tatarazako, and H. Yamamoto. 2013. "Ecotoxicity and Screening Level Ecotoxicological Risk Assessment of Five Antimicrobial Agents: Triclosan, Triclocarban, Resorcinol, Phenoxyethanol and p-thymol." Journal of Applied Toxicology 33: 1222–1229.
Ternes, T. A., M. Meisenheimer, D. McDowell, F. Sacher, H. Brauch Jr., B. Haist-Gulde, G. Preuss, U. Wilme, and N. Zulei-Seibert. 2002. "Removal of Pharmaceuticals during Drinking Water Treatment." Environmental Science and Technology 36: 3855–3863.
Toor, G. S., M. Lusk, and T. Obreza. 2011. Onsite Sewage Treatment and Disposal Systems: Trace Organic Chemicals. Gainesville: University of Florida Institute of Food and Agricultural Sciences. http://www.edis.ifas.ufl.edu/ss554.
United States Environmental Protection Agency (US EPA). 2009. How to Dispose of Medicines Properly.
United States Food and Drug Administration. 2013. "How to Dispose of Unused Medicines." Accessed February 10, 2014. http://www.fda.gov/ForConsumers/ConsumerUpdates/ucm101653.htm.
United States Pharmaceutical Statistics. 2013. "US Pharmaceuticals Sales—2012." Accessed December 2, 2013. http://www.drugs.com/stats/top100/2012/sales.
Vajda, A. M., L. B. Barber, J. L. Gray, E. M. Lopez, J. D. Woodling, and D. O. Norris. 2008. "Reproductive Disruption in Fish Downstream from an Estrogenic Wastewater Effluent." Environmental Science and Technology 42: 3407–3414.
Whitacre, D. M. 2011. "Reviews of Environmental Contamination and Toxicology." Springer 202: 79–84.
Xu, H., J. Yang, Y. Wang, Q. Jiang, H. Chen, and H. Song. 2008. "Exposure to 17α-ethynylestradiol Impairs Reproductive Functions of Both Male and Female zebrafish (Danio rerio)." Aquatic Toxicology 88: 1–8.
Zha, J., Z. Wang, N. Wang, and C. Ingersoll. 2007. "Histological Alternation and Vitellogenin Induction in Adult Rare Minnow (Gobiocypris rarus) after Exposure to Ethynylestradiol and Nonylphenol." Chemosphere 66: 488–495.
Zhang, Y., and S.-U. Geißen. 2010. "Prediction of Carbamazepine in Sewage Treatment Plant Effluents and Its Implications for Control Strategies of Pharmaceutical Aquatic Contamination." Chemosphere 80: 1345–1352.
Zhang, Y., S.-U. Geißen, and C. Gal. 2008. "Carbamazepine and Diclofenac: Removal in Wastewater Treatment Plants and Occurrence in Water Bodies." Chemosphere 73: 1151–1161.