Introduction
Just like a sponge, soils with high organic matter (OM) and aggregates can absorb and hold water during rainfall events and deliver it to plants during dry spells. Water is increasingly becoming the most limited natural resource supporting agriculture (Rijsberman 2006), but growers can improve their water storage capacity by raising their soil's OM content. The United States Department of Agriculture Natural Resources Conservation Service states that "for every 1% increase in soil OM, US cropland could store the amount of water that flows over Niagara Falls in 150 days" (Nichols 2015). Increasing soil OM is key to improving soil health (Doran and Zeiss 2000); however, this can be a challenging task in regions where the soils are sandy and depleted of OM. The purpose of this fact sheet is to (i) demonstrate how soil OM content can help increase water holding capacity (WHC) of soils; and (ii) describe the laboratory procedure to measure WHC.
Value of Improving Soil WHC to Florida Agriculture
Mineral soils in south Florida are generally sandy in texture and low in OM, reducing their available WHC and their ability to hold nutrients in the soil profile. Organic amendments have been shown to improve soils' chemical and physical properties and increase sugarcane yield on mineral soils (Gomez 2013). Bagasse, a dry, fibrous residue left after the extraction of juice from sugarcane, has not been tested as a soil amendment, and there is presently an overabundance of bagasse accumulating from the milling process. Applying this material to mineral soils as an amendment would be a valuable end-point to the waste stream, could improve soil health, and could potentially result in higher yields (Barzegar et al. 2002).
Commonly referred to as "muck" soils, the organic soils (histosols) in the Everglades Agricultural Area are excellent for agricultural production. Presently, all of the acres that are available are in production, and the subsidence of muck soils will cause soils to get shallower and therefore less suitable for agriculture. There has been a trend over the last 30 years of planting more crops on the less productive mineral soils in the region. In 2007, there were around 76,000 acres of sugarcane on the sandy soils; by 2015, the acreage on mineral soils had increased to almost 104,000 acres. This trend will most likely continue with the continued subsidence of the muck soils. Mineral soils are less economically productive than organic soils: production on organic soils is estimated to return $400/ha to land and management, whereas mineral soils only return $70/ha (Rokai et al. 2010). If agriculture in Florida is to prosper, production and economic return to the growers will need to increase.
Farming in Florida on sandy soils has a wide range of limiting factors, including nutrient deficiencies, acidity, poor physical attributes, and low water storage. The soils are characterized by their particle size, typically having low clay content, and being dominated by coarse particles. This coarse or sandy texture confers certain physical properties on the soils that influence the ability of the soil to hold water. In terms of WHC, sandy soils of Florida have larger pores than finer textured soils, making it more difficult to hold soil water against gravity; consequently, sandy soils have a lower capacity to store soil water for plant uptake. To plants, soil OM is a source of nutrients that also supports biological and physical characteristics (such as improved WHC); to farmers, it is a currency of exchange. By adopting farming methods that increase soil OM, Florida growers can increase the WHC of their soils. According to the USDA-NRCS, the most conservative estimates suggest that every 1% increase in soil OM will help soils hold up to 20,000 gallons more water per acre (Bryant 2015) (Figure 1). This would mean savings in energy cost associated with farming practices that depend on pump irrigation, and, for growers that depend on rain-fed irrigation, would equate to more "savings" in operating expenditure.
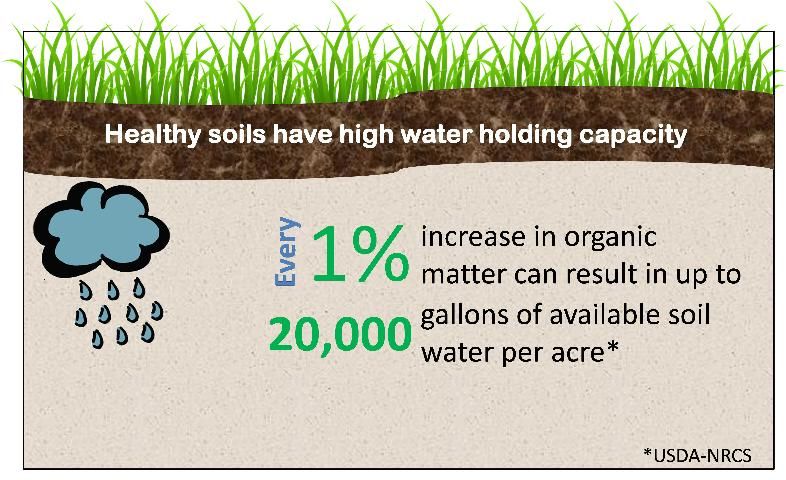
Credit: Jehangir Bhadha
Practices that Help Increase Soil Organic Matter
Conservation practices such as no-till, cover-cropping with high-residue crops, and diversifying crop rotations are some of the practices that increase WHC and improve soil health (Ashworth et al. 2017).
No/Low-Tillage
Tillage breaks soil aggregates, reducing the space in between that is necessary for air and water to flow through. By aerating the soil, tillage intensifies microbial activity in the soil, causing the soil OM to be consumed faster. This results in unhealthy soils with less structure, low OM, and low WHC. The "sponge" is lost, and so is the soil's water storing capacity; this can prove problematic during severe weather events. When heavy rain occurs, water and suspended particles are lost to ditches via seepage and runoff; this contributes to the loss of excess nutrients. During droughts, the soils lack moisture to sustain plants. Alternatively, no-till practices help improve the structure of the soil, and incorporating high-residue cover crops between planting seasons can increase soil OM and decrease erosion.
Cover Crops
Cover crops are proving to be vital in the development of soil health. Cover crops provide benefits such as reduced erosion and additional residue cover, but their greatest benefit may be what is happening under the soil surface. Cover crops provide active living roots that form symbiotic relationships with fungi (Steenwerth and Belina 2008). These relationships are crucial to building a healthy soil. Different types of mycorrhizal fungi can be found on almost 90% of all plants in the world. Fungi cannot develop their own food, so they attach themselves to living roots. The living roots provide carbohydrates to the mycorrhizal fungi, which in turn provide nutrients and water from the soil profile to the living root. The fungi then release polysaccharides and sugars that feed the soil bacteria. In turn, the bacteria supply nutrients to the mycorrhizal fungi, which can then be provided to the living root. The result is a more balanced ecosystem in which the bacteria populations are being fed at a steady rate and are not consuming existing OM in the soil. Mixes of cover crops that consist of warm- or cool-season grasses, broadleaves, and legumes mixed together appear to maximize the quantity and diversity of mycorrhizal fungi populations. Some commonly used cover crops suitable for Florida conditions are sunn hemp (Crotalaria juncea), sorghum, sorghum sudangrass (Sorghum bicolor), cowpea (Vigna unguiculata), and velvetbean (Mucuna deeringiana) (Wang et al. 2015).
Organic Amendments
One of the most desirable and readily accessible forms of biomass is crop residues, the biomass that remains after a crop is harvested. Considered "the greatest source of soil OM," crop residues have historically been used for animal bedding, burned, left on fields, and (more recently) converted into biofuels and other products. Utilizing locally derived crop residues such as bagasse (Figure 2), rice hulls, and palm fronds as soil amendments on sandy soils can potentially increase soil OM. Leaving this residue on the soil to naturally break down provides additional carbon to feed the soil. Gould (2015) showed that the application of compost increased water holding capacity of droughty soils.
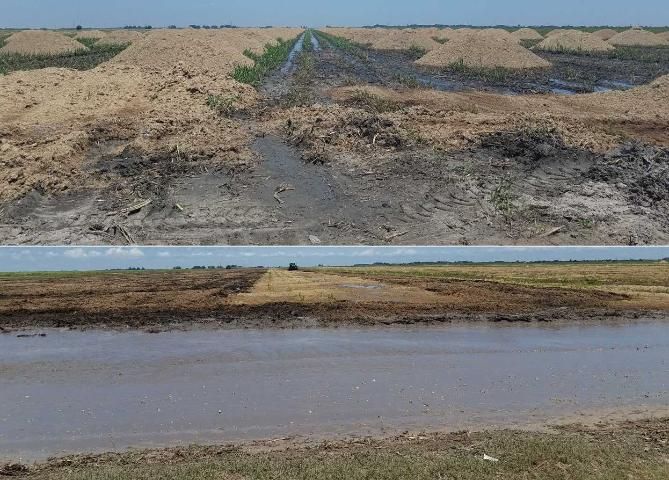
Credit: Raju Khatiwada and Stewart Swanson.
Effect of OM Addition on Sand and Limerock
A laboratory-based experiment was conducted to study the effect of OM addition on soil WHC. The study was conducted on two types of soil: sandy soil (with less than 1% OM) and calcareous soil (primarily composed of limerock, with less than 6% OM). Muck soil containing 80% OM was added at one percent increments as a source of OM to mimic field conditions. Treatments included 0, 1, 2, 3, 4, 5, and 10% OM added to two different matrices (sand and limerock). The experiment was repeated three times, and the mean values have been reported. Water holding capacity was determined by using a modified version of the method described by Jenkinson and Powlson (1976), in which 50 g of mixture (sand/limerock with OM) was placed in a funnel with a Whatman No.5 filter paper (185 mm diameter). The funnel was attached to a rubber hose with a stopper attached to the neck. Then 50 mL of water was added to the mixture and allowed to settle for 30 mins. After 30 mins, the stopper was removed from the rubber hose and allowed to drain for 30 mins. The drained water was collected in a measuring tube (Figure 3). Water holding capacity was calculated based on the difference between the volume of water added and the volume of water drained, minus the water held in the filter paper (1.98 mL ± 0.18), plus the water previously in the soil, as shown in Equation 1.
Eq. 1
WHC% = ([50 mL – water drained – 1.98 mL + water in soil previously]/50 g) × 100
Results indicated that there was a steady increase in WHC with every 1% increase in OM content for both sandy soils and limerock (Figure 4). Based on our study, every 1% increase of OM equates to a 2.3% and a 1.3% increase in soil WHC for sand and limerock, respectively. This would equate to an average of 10 gallons of water stored per acre-foot on sandy soils and 9.1 gallons of water stored per acre-foot of limerock if OM content were to be increased by 1%.
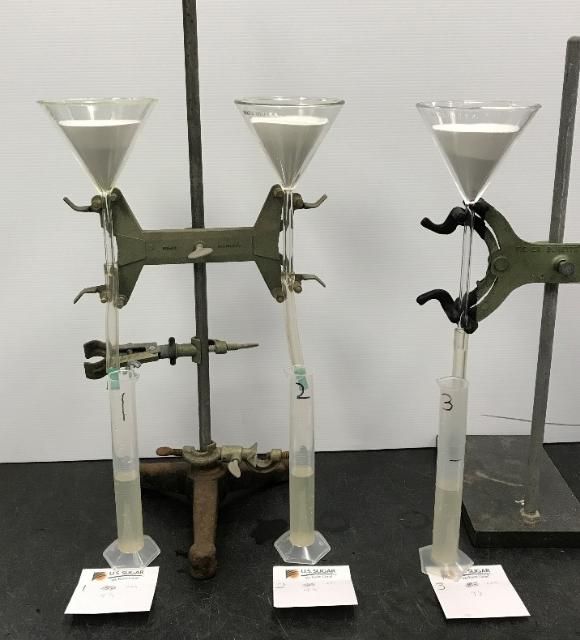
Credit: Jay Capasso
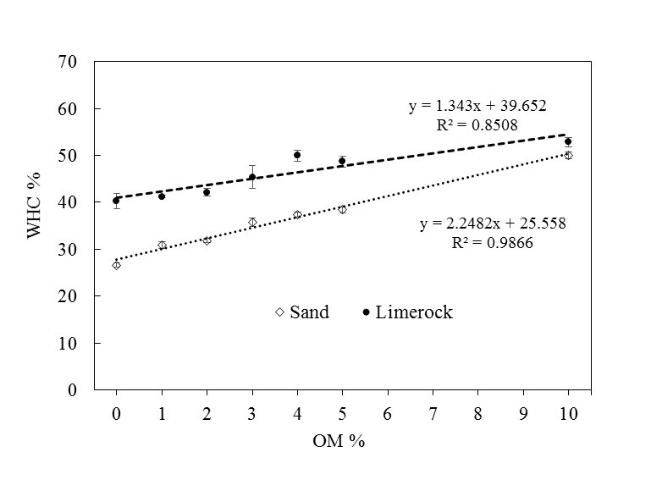
The Importance of Soil Water Holding Capacity
The natural WHC of Florida's mineral soils is low due to their sandy nature and low OM content. The small amount (often less than 2%) of OM in Florida's mineral soils is responsible for most of the natural soil fertility and WHC. This means that almost 100% of the nutrient and moisture content depends on <2% of soil OM present in Florida's sandy soils. As a result, Florida's sandy soils usually struggle to provide a sufficient amount of nutrients and soil moisture to growing plants. Consequently, copious amounts of fertilizer and water are applied to the soils in order to correct or prevent nutrient and water-related deficiencies, causing runoff of nutrients to surface waters and leaching into groundwater.
In addition, climate change is already affecting weather patterns; this makes farming riskier, as drought and flood expose soil to erosion and degradation. The weather pattern in Florida has shifted. Hard, fast rains will be followed by long periods of drought, and soil cannot keep up with the changing climate under conventional production agriculture (Borisova 2014). This can be combated by finding ways to slow the water down, trap it at the surface, give it time to infiltrate, and make sure it is retained in the soil for prolonged periods. This could be achieved by gradually increasing the OM content of the soils. Organic matter can retain up to ten times its weight of water, because OM particles have a charged surface that attracts water so that it adheres to the surface like static cling. Recent studies have shown strong positive correlations between OM content and WHC within soils. For example, the WHC of a silt loam containing 4% OM by weight is more than twice that of a silt loam containing 1% OM by weight (Hudson 1994). The pronounced effect of soil OM on WHC suggests that soil OM must be considered a key factor in agricultural productivity and can have a significant impact on water conservation and usage.
Ultimately, we have to consider the human aspect in dealing with issues related to soil and water conservation. Human population has quadrupled in the last century, partly because of advances in agricultural and industrial technology (Taiz 2013). However, this boom in population is generating continued pressure on agricultural production systems to feed the growing numbers. As a result, agricultural practices are getting more intensive with higher demand for water use, and that has started to take a toll on the quality of agricultural soils. Awareness of the environmental aspects of soil health and water conservation has been increasing in recent years among both the general public and the scientific community, which has led to renewed interest in identifying ways soil and water can be managed sustainably for agricultural production.
References
Ashworth, A.J., F.L. Allen, A.M. Saxton, and D.D. Tyler. 2017. "Impact of crop rotations and soil amendments on long-term no-tilled soybean yield." Agronomy Journal 109: 938–946.
Barzegar A.R., A. Yousefi, and A. Daryashenas. 2002. "The effect of addition of different amounts and types of organic materials on soil physical properties and yield of wheat." Plant and Soil 247: 295–301.
Borisova T., N. Breuer, and R. Carriker. 2014. Economic Impacts of Climate Change on Florida: Estimates from Two Studies. FE-787. Gainesville: University of Florida Institute of Food and Agricultural Sciences. https://edis.ifas.ufl.edu/FE787
Bryant, L. 2015. "Organic Matter Can Improve Your Soil's Water Holding Capacity." NRDC Expert Blog. https://www.nrdc.org/experts/lara-bryant/organic-matter-can-improve-your-soils-water-holding-capacity
Doran, J.W., and M.R. Zeiss. 2000. "Soil health and sustainability: managing the biotic component of soil quality." Applied Soil Ecology 15: 3–11.
Gomez, S.M. 2013. Recycling agricultural by-products to grow sugarcane on sandy soils in South Florida. MS Thesis. University of Florida.
Gould C. M. 2015. "Compost increases the water holding capacity of droughty soils." Michigan State University Extension. https://www.canr.msu.edu/news/compost_increases_the_water_holding_capacity_of_droughty_soils
Hudson, B.D. 1994. "Soil organic matter and available water capacity." Journal of Soil and Water Conservation 49: 189–194.
Jenkinson, D.S., and D.S. Powlson. 1976. "Effects of biocidal treatments on metabolism in soil-V: A method for measuring soil biomass." Soil Biology and Biochemistry 8: 209–213.
Nichols, R. 2015. "A Hedge against Drought: Why Healthy Soil is 'Water in the Bank'." United States Department of Agriculture. Natural Resources Conservation Service. https://www.usda.gov/media/blog/2015/05/12/hedge-against-drought-why-healthy-soil-water-bank
Rijsberman, F.R. 2006. "Water scarcity: Fact or fiction?" Agricultural Water Management 80: 5-22.
Rokai, F.M., L.E. Baucum, R.W. Rice, and J. Alvarez. 2010. "Comparing costs and return for sugarcane production on sand and muck soils of Southern Florida, 2008–2009." Journal American Society of Sugarcane Technologist 30: 50–66.
Steenwerth, K., and K.M. Belina. 2008. "Cover crops enhance soil organic matter, carbon dynamics and microbiological function in a vineyard agroecosystem." Applied Soil Ecology 40: 359–369.
Taiz, L. 2013. "Agriculture, plant physiology, and human population growth: past, present, and future." Theoretical and Experimental Plant Physiology 25: 167–181.
Wang, Q., Y. Li, E.A. Hanlon, W. Klassen, T. Olzcyk, and I.V. Ezenwa. 2015. Cover Crop Benefits for South Florida Commercial Vegetable Producers. SL-242. Gainesville: University of Florida Institute of Food and Agricultural Sciences. https://edis.ifas.ufl.edu/SS461