Background
Agriculture has historically provided much more than just food. It offers a multitude of societal and environmental benefits, including water storage, climate regulation, wildlife habitat, and biodiversity. However, today’s agriculture faces increased pressure to meet global demand for food, while balancing economic and environmental objectives. In addition, weather uncertainties and extreme flood and drought events made worse by climate changepose additional threats to agricultural production. For instance, projected increases in temperatures and changes in precipitation patterns are expected to result in reduced agricultural productivity and threaten food security (Brown et al. 2015). However, these challenges also provide new opportunities for farmers to play an active role in climate change mitigation. In many parts of the world, there has been significant emphasis on encouraging farmers to manage their natural resources in ways that enhance the provisioning of ecosystem services. The ability of agriculture to produce noncommodity outputs and specifically the possibility of farmers to participate in carbon trading markets or payment programs for ecosystem services are potential feasible opportunities for agriculture to play an important role in climate change mitigation.
This publication provides information about the role of native and cultivated pastures in climate change mitigation and the opportunities and challenges for improving carbon sequestration in agroecosystems. Climate change mitigation in the context of this publication refers to actions that reduce the rate of climate change. This information should be of interest to stakeholders, students, scientists, and environmental agencies interested in enhancing ecosystems services provided by grazing lands.
Greenhouse Gas Emissions from Agriculture
Agricultural lands have a significant impact on the global biogeochemical cycle of carbon and nitrogen, including agriculture activities that often result in the release of greenhouse gasses (GHG) to the atmosphere. Global estimates suggested that GHG emissions from agriculture contributed from 10% to 12% of total anthropogenic emissions (Smith et al. 2007). In 2018, the US EPA (2020) estimated that agriculture and forestry activities (including carbon dioxide emissions associated with agricultural electricity consumption) accounted for 9.3% of US GHG emissions. Within the agriculture sector, grazing lands (pastures and rangelands) are responsible for more than half of agricultural emissions. However, large uncertainties exist around these estimates. Decreasing agricultural GHG emissions is undoubtedly important; however, the lack of accurate estimates presents a major challenge to understand agriculture’s potential for contributing to climate change mitigation potential. For instance, emission factors linked to beef cattle production (i.e., emissions from manure and enteric fermentation) vary significantly regionally and temporarily. Differences in production efficiencies, new breed introductions, and implementing more efficient production methods (e.g., rotational grazing, dietary modifications) can result in significant reductions in GHG emissions. There is also evidence suggesting significant regional differences in agricultural emissions with emissions increasing at a faster rate in developing countries than those in developed countries.
Pastures as a Viable Climate Change Mitigation Strategy
Although agriculture is currently a substantial source of GHG at a global scale, there is a growing body of evidence suggesting that agriculture can also capture significant amounts of carbon dioxide from the atmosphere, mainly through soil carbon storage. Estimates suggest that cropland and grazing land soils can store up to 8.6 gigatons of carbon dioxide per year (IPCC 2019). Increases in carbon storage in agricultural soils can offset approximately 12% of total US GHG emissions (US EPA 2020). Improvements in grazing land management, forage production, and associated soil carbon stocks and manure management are among the most effective options for increasing soil carbon sequestration and mitigation potential of livestock systems. Scientists are currently using integrated approaches to better understand and quantify the potential benefits and tradeoffs associated with implementing different management practices specifically targeted at a particular region, climate, soil type, and cropping system. Ideally, the goal is to use science-based information as part of decision-support tools that will help farmers choose the most effective practices. Most of these practices also provide cobenefits, including improvements in soil health, wildlife habitat, and farm resilience to drought and flooding. However, some beneficial practices are not being implemented, which suggests that multiple barriers to implementation still exist. A major hurdle is the lack of studies documenting specific benefits and tradeoffs associated with different management practices. Lack of data also increases the uncertainty and errors associated with GHG emission estimates. Generating reliable metrics that can also be used as benchmarks for ecosystem services credit market may also provide a critical step in increasing adoption of conservation measures.
Opportunities to Increase Soil Carbon Accumulation in Pastures
Pasture management can have a major role on ecosystem carbon balance. Management practices that increase productivity, such as fertilization, irrigation, use of productive perennial grass species, presence of legumes, and proper grazing management, are often associated with increased soil carbon stocks. An evaluation of research in several states indicated that conversion of croplands to pastures is expected to initially add 0.4 to 1.1 metric ton of soil carbon per hectare each year (Follett et al. 2001). However, pasture soils generally accumulate carbon for 15 to 25 years after establishment or through significant improvement in management, but then tend to stabilize. This suggests that soils have a finite ability to accumulate carbon (also known as soil carbon saturation) that is often determined by the soil’s inherent chemical, biological, and physical properties. Once surface soils become saturated with respect to carbon, the use of deep-rooted species can be viable option to continue sequestering carbon at deeper soil depths. Studies in Florida demonstrated that both native rangelands and cultivated pastures are strong carbon sinks sequestering as much as 0.9 metric tons of carbon ha-1 yr-1 (Adewopo et al. 2014). Our data also demonstrated that pasture intensification through the introduction of more productive plant species (i.e., conversion of native vegetation into warm-season grasses) and adoption of proper grazing and fertilization management strategies increased soil carbon by 50%, or the equivalent of ~1 metric ton of carbon per year (Adewopo et al. 2014; Xu et al. 2016). In addition, data also suggested that adoption of improved pasture management practices were also often beneficial to forage and livestock production. This can be an incentive for producers to adopt strategies that enhance soil carbon sequestration while simultaneously increasing forage production and farm income.
In a recent study using an atmospheric measurement technique known as eddy covariance (Figure 1), we evaluated the impact of fire on ecosystem carbon fluxes. Our data (Bracho et al. 2021) demonstrated that native rangeland vegetation fully recovered its photosynthetic capacity 60 days following prescribed fire and aboveground biomass four months after fire. Our data also showed that native rangelands represent a strong carbon sink, sequestering over 3.7 Mg carbon ha-1 per year. Similarly, native rangelands act as methane sinks, offsetting between -17 and -39 kg CO2eqv ha-1 yr-1 as methane.
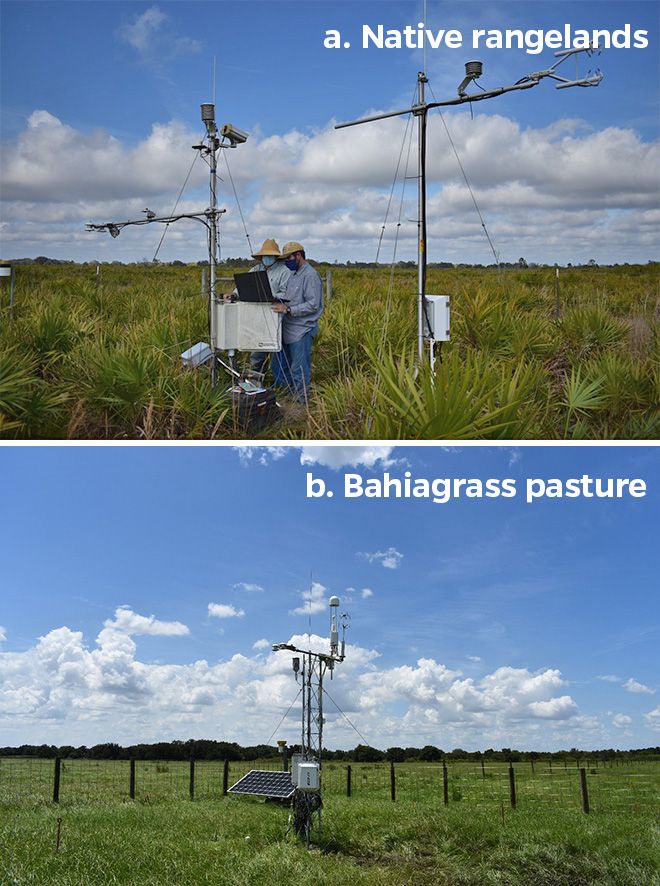
Credit: Maria L. Silveira, UF/IFAS
Challenges to Measuring Climate Mitigation Benefits
Despite the fact that soil carbon sequestration credit is gaining popularity in many countries, including Australia and the United States, these emerging agriculture-based climate credit programs are still facing many challenges, including a lack of practical tools to accurately measure and monitor carbon sequestration benefits. Reliable measurement tools and models are critical to design and validate the benefits of adopting climate-smart practices and to inform land managers and policy makers. A combination of field measurements and modelling approaches are necessary to accurately measure and predict the impacts of management practices on GHG balance at different spatial scales.
Although there are well-established laboratory methods to accurately measure soil carbon, assessing changes in soil carbon in a field over time requires significant investment in time and financial resources. Soil carbon concentrations in a field vary significantly spatially, which complicates detection of management effects on soil carbon sequestration. For example, a large number of samples (collected at 10 m or smaller intervals) might be needed to adequately estimate the average soil carbon concentration within a field. Accurate carbon accounting will also require collecting deep soil samples that better represents the crop rooting zone (Figure 2), which often extends beyond the minimum of 12 inches recommended by the Intergovernmental Panel on Climate Change (IPCC) or the typical 6 inches for soil fertility purposes. The extensive labor requirement for sampling and analysis cost should be considered in the design of carbon credit programs if measured verification is required.
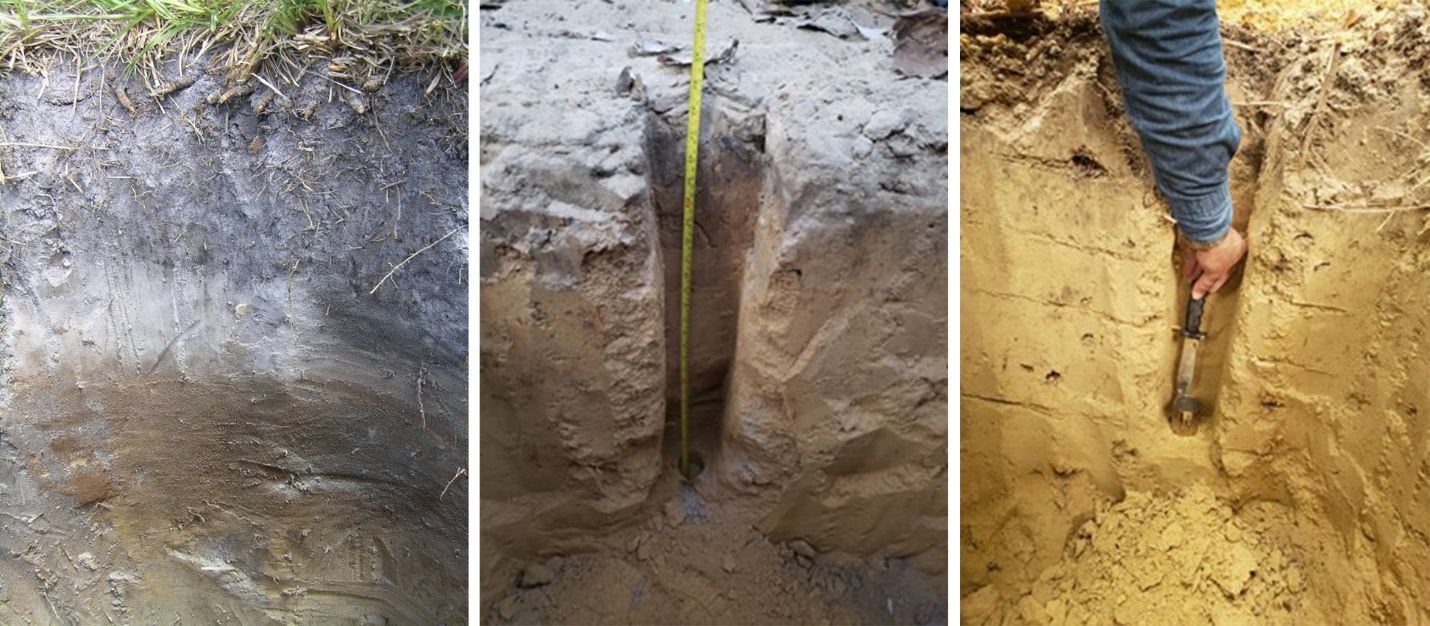
Credit: Maria L. Silveira and Abmael Cardoso, UF/IFAS
Another approach to estimate ecosystem carbon balance is through the eddy covariance technique (Figure 1). Eddy covariance consists of a micrometeorological method that allows continuous measurement of ecosystem-level net carbon dioxide and methane exchange. This technique has been widely applied to different ecosystems (croplands, grasslands, forests). Despite its high accuracy and many other advantages associated with using the eddy covariance method, the high cost and required skill to operate and maintain flux towers limit the extent to which this technique can be used more broadly.
Implications for Producers
- Despite challenges, increased opportunities currently exist for agriculture to play a vital role in climate change mitigation. However, for this to happen it will be important to improve the accuracy of estimates associated with the adoption of specific management practices on ecosystem carbon balance at multiple scales (from field, farm, and national level).
- Although carbon accounting can be accomplished in a research setting, direct farm-level measurement of year-to-year changes in soil organic carbon is highly unrealistic.
- Additional investment into long-term, coordinated research is necessary to develop methodologies for quantifying and verifying the benefits and trade-offs associated with climate smart practices.
- Financial incentive and voluntary programs should be expanded to incentivize producers to adopt management practices that reduce GHG emissions and/or increase soil carbon sequestration.
- Many of these “soil C enhancing” practices can also provide multiple agronomic and environmental cobenefits, which can further strengthen their adoption.
References
Adewopo, J. B., M. L. Silveira, S. Xu, S. Gerber, L. E. Sollenberger, and T. A. Martin. 2014. “Management Intensification Impacts on Soil and Ecosystem Carbon Stocks in Subtropical Grasslands.” Soil Science Society of America Journal 78 (3): 977–986. https://doi.org/10.2136/sssaj2013.12.0523
Bracho, R., M. L. Silveira, R. Boughton, J. M. D. Sanchez, M. M. Kohmann, C. B. Brandani, and G. Celis. 2021. “Carbon Dynamics and Soil Greenhouse Fluxes in a Florida's [sic] Native Rangeland before and after Fire.” Agricultural and Forest Meteorology 311:108682. https://doi.org/10.1016/j.agrformet.2021.108682
Brown, M. E., J. M. Antle, P. Backlund, E. R. Carr, W. E. Easterling, M. K. Walsh, C. Ammann, W. Attavanich, C. B. Barrett, M. F. Bellemare, V. Dancheck, C. Funk, K. Grace, J. S. I. Ingram, H. Jiang, H. Maletta, T. Mata, A. Murray, M. Ngugi, D. Ojima, B. O’Neill, and C. Tebaldi. 2015. Climate Change, Global Food Security, and the US Food System. https://doi.org/10.7930/J0862DC7
Follett, R. F., J. M. Kimble, and R. Lal. 2001. “The Potential of US Grazing Lands to Sequester Soil Carbon.” In The Potential of U.S. Grazing Lands to Sequester Carbon and Mitigate the Greenhouse Effect, edited by R. F. Follett, J. M. Kimble, and R. Lal, chapter 16, pp. 399–428. Lewis Publishers. https://doi.org/10.1201/9781420032468
Intergovernmental Panel on Climate Change (IPCC). 2019. Climate Change and Land: An IPCC Special Report on Climate Change, Desertification, Land Degradation, Sustainable Land Management, Food Security, and Greenhouse Gas Fluxes in Terrestrial Ecosystems, edited by P. R. Shukla, J. Skea, E. Calvo Buendia, V. Masson-Delmotte, H.-O. Pörtner, D. C. Roberts, P. Zhai, R. Slade, S. Connors, R. van Diemen, M. Ferrat, E. Haughey, S. Luz, S. Neogi, M. Pathak, J. Petzold, J. Portugal Pereira, P. Vyas, E. Huntley, K. Kissick, M. Belkacemi, and J. Malley. https://www.ipcc.ch/site/assets/uploads/2019/11/SRCCL-Full-Report-Compiled-191128.pdf
Smith, P., D. Martino, Z. Cai, D. Gwary, H. Janzen, P. Kumar, B. McCarl, S. Ogle, F. O’Mara, C. Rice, B. Scholes, and O. Sirotenko. 2007. “Agriculture.” In Climate Change 2007: Mitigation. Contribution of Working Group III to the Fourth Assessment Report of the Intergovernmental Panel on Climate Change, edited by B. Metz, O. R. Davidson, P. R. Bosch, R. Dave, and L. A. Meyer, pp. 497–540. Cambridge University Press. https://archive.ipcc.ch/pdf/assessment-report/ar4/wg3/ar4-wg3-chapter8.pdf
US EPA. 2020. Inventory of U.S. Greenhouse Gas Emissions and Sinks: 1990–2018. https://www.epa.gov/ghgemissions/inventory-us-greenhouse-gas-emissions-and-sinks-1990-2018
Xu, S. T., M. L. Silveira, K. S. Inglett, L. E. Sollenberger, and S. Gerber. 2016. “Effect of Land-Use Conversion on Ecosystem C Stock and Distribution in Subtropical Grazing Lands.” Plant Soil 399 (1–2): 233–245. https://doi.org/10.1007/s11104-015-2690-3