This publication outlines the importance of biological nitrogen fixation (BNF) and offers advice to Extension agents on how to reduce the use of chemical fertilizer by improving BNF on commercial farms. Florida-grown BNF crops with different seed rates, planting dates, and potential biomass productions are detailed below. The publication also discusses the world’s nitrogen (N) sources, including BNF, to inform the reader of N sources other than chemical production.
This information, previously unavailable as one publication on EDIS, is more critical than ever: Florida Senate Bill (SB) 712, the “Clean Water Act,” requires multiple water quality protection provisions that aim to minimize the effect of nutrient pollution sources. This law impacts agriculture producers and landowners enrolled in the Florida Department of Agriculture and Consumer Services (FDACS) Best Management Practices Program (BMPs). Agriculture producers and landowners are now required to adhere to the nutrient recommendations provided by the University of Florida as a result of this statute.
Introduction
Every year, nitrogen fixation adds approximately 413 teragrams (Tg) of N to the earth system, including marine and terrestrial ecosystems. (1 Tg equals 2.2046 billion lb.) Much of this fixation contributes to the landmass at the rate of approximately 240 Tg. Figure 1 represents the total N additions and losses across all sources on Earth (N cycling).
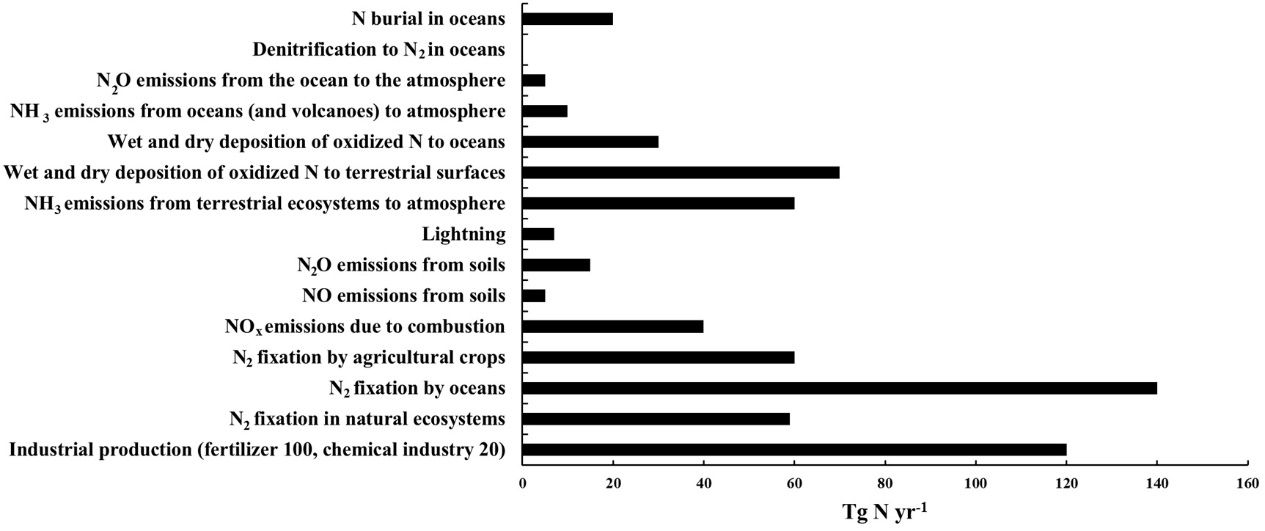
Credit: Adapted from Fowler et al. (2013)
Nitrogen (N) is one of the most crucial nutrient elements for plant growth and optimum yields. The synthesis of essential plant structural components such as proteins, amino acids, and chlorophyll depends on N. About 80% of the atmosphere consists of nitrogen as N2, but plants cannot absorb or uptake N2. N fixation transforms atmospheric N (N2) into plant-available forms such as nitrate (NO3-) or ammonium (NH4+). The process can occur naturally or in industrial labs; synthetic fertilizers are the product of N fixation through an industrial process known as Haber-Bosch. Various commonly used ammonium N fertilizer sources are prone to volatilization; and nitrate sources or ammonium sources converted into nitrate by soil microbes are prone to leaching losses. Coating fertilizers with other secondary elements such as sulfur, neem (Azadirachta indica A. Juss.), or polymers, including polyolefins ethylene-vinyl acetate copolymers, can help address this issue. These coatings permit a gradual release of fertilizer-N into the soil. By slowly releasing the fertilizer to synchronize with crop plant requirements, the coatings decrease the soil’s susceptibility to losses. Despite this, these approaches are not enough to maximize N use efficiency. A significant amount of N is still lost in the atmosphere and bodies of water, leading to multiple negative impacts on the environment, such as the eutrophication of water bodies.
One may reduce synthetic N fertilizer use, increase N use efficiency, and protect water quality by utilizing biological nitrogen fixation (BNF)—a form of nitrogen fixation by biological entities such as leguminous plants or soil bacteria. BNF is an economically and environmentally sustainable source of N fertilizer. BNF could play a major role in N management in Florida and enhance the sustainability of food production systems. This article discusses the process of BNF, potential crops capable of BNF, and its importance to the sustainability of crop production in Florida. Extension agents can utilize this information to select cover crops that can fix N for their Extension programs.
Biological nitrogen fixation can be better understood by dividing it into two broad categories based on the relationship between plant and biological entities: symbiotic and non-symbiotic N fixation. Both symbiotic and non-symbiotic biological organisms can fix atmospheric N at different intensities, soil conditions, and seasons. Most significantly, legumes fix N through symbiotic relationships with some bacteria. Microorganisms that can fix N outside of plants are known as N fixers, and they belong to various groups, including heterotrophic bacteria (Rhizobium, Azotobacter, Azospirillum, Bacillus, Mycobacterium, and Frankia), archaea (Methanobacteriales, Methanococcales, and Methanobacteriales), and cyanobacteria (Anabaena, Nostoc, Anabaenopsis, and Tolypothrix) (Soumare et al. 2020).
Symbiotic N Fixation
Symbiotic BNF can be defined as an association where both types of organisms (microorganisms and plants) mutually benefit from each other. Microorganisms obtain food and energy from the plant, while the plant utilizes the N produced by microorganisms for its growth and development. From the agricultural point of view, Rhizobium spp. (symbiotic bacteria) are the most important N-fixing species. In symbiotic fixation, flavonoids (plant chemicals also known as nod factors) released from the legume plant root activate the nearby rhizobia to form the nodules. The symbiotic N fixation process is host-specific: each Rhizobium strain can only fix N with a specific crop. That symbiotic relationship will thus fail to fix N if a mismatch between the appropriate bacterium and legume occurs. Figure 2 summarizes the process of symbiotic BNF.
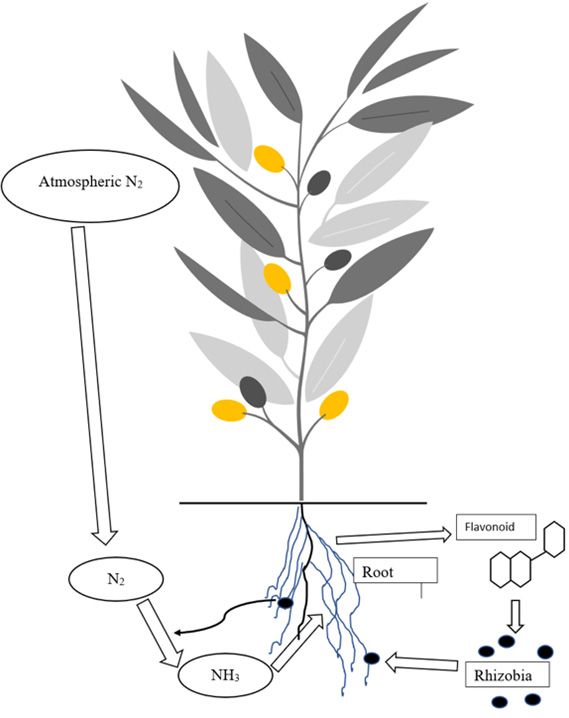
Credit: K. Sharma, UF/IFAS
Non-Symbiotic N Fixation
In contrast to symbiotic BNF, non-symbiotic (asymbiotic) N fixation is accomplished by free-living microbes that do not require a plant host to complete the fixation process. Asymbiotic nitrogen fixation can be performed by free-living aerobic or anaerobic N-fixing bacteria such as Chlorobium, Azotobacter, Azomonas, Derxia, Beijerinckia, and Cyanobacterium. For instance, research has documented that Azotobacter biofertilizer has increased the available N by 12.5 lb. per acre per year for lettuce-lettuce-turnip crop rotation (Rodrigues et al. 2018).
Fixed Nitrogen: Where to Find It and How Much to Use
Since symbiotic N-fixing bacteria dwell in the nodules formed on the roots of legume plants, it is often assumed that the fixed N is stored in the roots. However, most of the N fixed by legumes is stored in the plant leaves and stems. Short-season annual legumes have smaller root systems, and N contribution from root systems is thus relatively smaller. The fixed N accumulates in different parts (roots, stems, and leaves) made available to the following crop on the decomposition of legumes after their termination. In pastures, animals may consume the N-rich above-ground biomass of legumes as a forage and recycle it through manure and urine.
The amount of N fixed depends on the biomass produced and the protein content of the biomass. For example, alfalfa may yield up to 8 tons of dry matter per acre per year if appropriately managed, and biomass may have about 20% crude protein. This results in a total 1.6 tons of crude protein per acre. Assuming the average N content of proteins is about 16%, one can harvest 512 lb. per acre of N from the alfalfa field. The N fixation in the annual legume system is much lower due to lower biomass production. However, the amount of N fixed by different legumes varies widely. (Typical ranges of the amount of biomass produced and N fixed by different legumes in Florida conditions are given in Table 1.) However, it is estimated that only about half of this fixed N is available for the following crop; the other 50% is lost to the environment.
Factors Affecting BNF
Since BNF is associated with microorganisms, all factors impacting microbial diversity and population can potentially impact BNF. These factors include environmental stresses, utilization of chemical fertilizers, herbicides, soil fumigation, and soil pH. For example, at low temperatures during winter, most microbes are less active than in spring and summer. BNF can be similarly affected by extremely high temperature conditions, and repetitive, long-term fumigation affects the soil microbial population (Dangi et al., 2017). Typically, fumigation is practiced to help farmers control soilborne pathogens such as fungi, bacteria, and nematodes, but fumigation also has a negative impact on beneficial microorganisms that are essential for the mineralization and decomposition of crop residues. Studies report a negative relationship between chemical fertilizers and BNF.
Increasing BNF
Biological nitrogen fixation depends on the soil microbial communities and cropping pattern. When intensively grown along with higher rhizobium populations, legume crops fix more N, and both crop and rhizobium populations are positively grown together. The maximum population occurs when farmers grow legume crops in consecutive years to maintain hosts for rhizobia survival. Therefore, soil’s microbial communities are crucial for an effective BNF. Additionally, the rate of N fixation is higher in perennial legumes such as alfalfa than in annual legumes such as soybeans, peas, and beans. In annual legumes, root nodules are formed fresh every year, while in perennial legumes, the nodules are formed in the very first year of their growth. These first-year nodules persist into subsequent years and, therefore, fix more atmospheric N (N2).
To achieve the maximum benefits of BNF, growers need to adopt management that promotes soil health and reduce fallow periods, using appropriate cover crops and rotations to maintain the required microbial population for N fixation. Also, the factors favoring photosynthesis, such as adequate soil moisture, warm temperatures, and higher CO2 levels, are reported to enhance BNF. For example, the increase in the seed rate of cover crop has helped farmers from northeast Florida increase their soil’s organic matter and N content. Please follow Table 1 for selecting the crop for increasing BNF.
Inoculants and their Handling
Inoculants are generally powdered forms of microorganisms (bacteria or fungi) added to the soil or coated on seed (inoculated seed). As mentioned earlier, the symbiotic N fixation process is host-specific, in which the strain of bacteria can only fix N with a specific crop. Therefore, it is important that the proper strain of bacteria be introduced into the cropping system. Planting inoculated seeds is the best way to introduce new bacteria into soils. Inoculation of legume seed should occur before planting if one:
- Buys pre-inoculated seed.
- Applies a sticking agent to the seed, then adds a peat-based inoculant. One should mix seed and inoculant until all seeds are well coated. The sticking agent may be a commercial preparation sold by the inoculant manufacturer. Coating seed with a 10 percent sugar solution can also be effective.
- Uses inoculants with clay; the clay helps bacteria adhere to seeds when well mixed.
- Handling inoculants with care, so that the live microorganisms (bacterial or fungi) that the inoculants contain are not killed. Inoculants are ineffective in the field if these microorganisms die. One should also use the inoculant prior to its expiration date as mentioned on the container.
- Stores the inoculant and inoculated seed at favorable temperatures. Avoid mixing the inoculant or inoculated seed with fertilizers or other chemicals. Unfavorable temperature and mixing can be lethal for the organisms and result in ineffectiveness.
Potential of BNF using Legumes in Florida
Biological nitrogen fixation has been widely studied in the Leguminosae family (i.e., legumes)—which includes green beans, peas, red beans, black beans, soybeans, peanuts, etc. These are some of the economically important legume crops in Florida; they can fix N from the atmosphere with the aid of the Rhizobium’s symbiosis. Legumes can be intercropped as an N source for the other crops; or legumes can be used as cover crops to replace the fallow period, providing N after termination to the following cycle. Reports show that intercropping perennial peanut species with bahiagrass can reduce the N requirement of bahiagrass in North Florida (Jaramillo et al. 2018). Legume crops such as Sunn hemp (Crotalaria juncea L.) could fix about 100 lb./acre of N from over 5000 lb. of biomass. Figure 3 shows nodules in the Sunn hemp crop. Sunn hemp is a widely used cover crop in several other states around the nation, and it does not host any nematode species. In Florida, Sunn hemp has been recently adopted by several big farmers, especially potato growers. Reduced Sunn hemp seed prices also encourage farmers to use it as a cover crop. Replacing the fallow period using a leguminous cover crop provides more benefits than just serving as N source. Leaving land fallow poses several environmental and economic sustainability issues. Fallow land is susceptible to higher nutrient leaching and soil erosion. Growing legumes as a cover crop helps fix N from the atmosphere, supplying it to the soil, and can also avoid leaching losses and erosion since legumes provide soil coverage. Cultivating cover crops also helps to maintain soil health by increasing organic matter and stimulating microbial populations. The N present in the cover crop residues will be mineralized and has the potential to partially supply the N demands of the following crop. However, the N release from cover crop biomass depends on several other factors, such as C:N ratio, temperature, and soil moisture; these factors influence the decomposition of cover crop residue and N release. Moreover, a lower C:N ratio equates to a faster release of N. Therefore, it is recommended to include legume crops in the crop rotation and terminate cover crops at proper maturity to take advantage of these benefits.
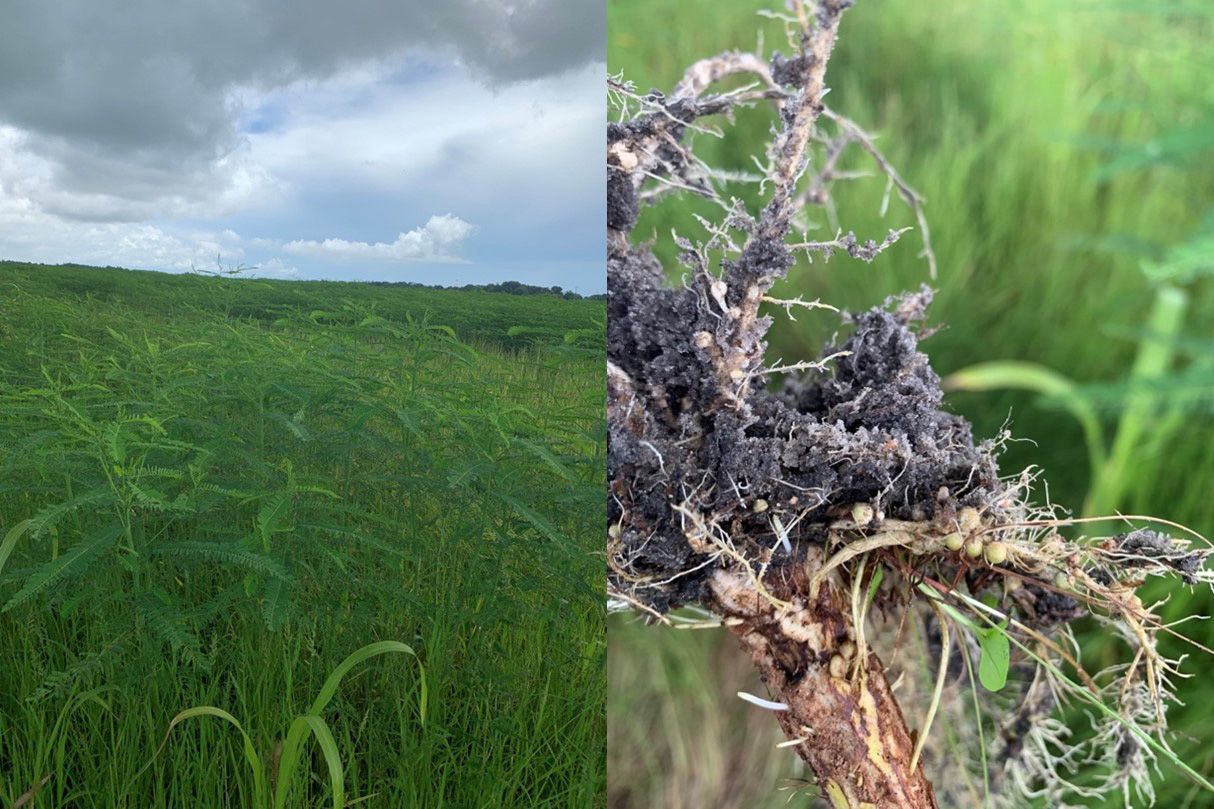
Credit: L. Sharma, UF/IFAS
Legumes are excellent cover crops for N fixation and should be leveraged to partially supply N for subsequent crop plants. Growers need to estimate the N credits available from these leguminous cover crops to adjust fertilizer N recommendations to the following crops (please follow Table 1 for N credit). As mentioned earlier, the total amount of N supplied by a legume depends upon its biomass productivity and nitrogen content. Legume cover crops have the potential for the highest BNF and can fix substantial amounts of plant-available N for the next crop. Legume cover crops could yield between 70 to 100 lb. N per acre when grown to the bud stage to gain sufficient size (biomass). The total N fixation potential for different legumes grown in Florida is presented in Table 1.
Table 1. This table presents Florida's nitrogen fixation potential of different winter, summer, and perennial legumes. The information in this table was adopted from Wright et al., SS-AGR-66.
References
Dangi, S. R., R. Tirado-Corbalá, J. Gerik, and B. D. Hanson. 2017. “Effect of Long-Term Continuous Fumigation on Soil Microbial Communities.” Agronomy 7 (2): 37. https://doi.org/10.3390/agronomy7020037
Fowler, D., J. A. Pyle, J. A. Raven, and M. A. Sutton. 2013. “The Global Nitrogen Cycle in the Twenty-First Century: Introduction.” Philosophical Transactions of the Royal Society B: Biological Sciences 368 (1621): 20130165. https://doi.org/10.1098%2Frstb.2013.0165
Jaramillo, D. M., J. C. Dubeux Jr., C. Mackowiak, L. E. Sollenberger, N. DiLorenzo, D. L. Rowland, A. R. S. Blount, E. R. S. Santos, L. Garcia, and M. Ruiz‐Moreno. 2018. “Annual and Perennial Peanut Mixed with ‘Pensacola’ Bahiagrass in North Florida.” Crop Science 58 (2): 982–992. https://doi.org/10.2135/cropsci2017.09.0542
Rodrigues, M. Â., L. C. Ladeira, and M. Arrobas. 2018. “Azotobacter-Enriched Organic Manures to Increase Nitrogen Fixation and Crop Productivity.” European Journal of Agronomy 93: 88–94. https://doi.org/10.1016/j.eja.2018.01.002
Sharma, L.K. and S. K. Bali. 2018. “A Review of Methods to Improve Nitrogen Use Efficiency in Agriculture.” Sustainability 10 (1): 51. https://doi.org/10.3390/su10010051
Soumare, A., A. G. Diedhiou, M. Thuita, M. Hafidi, Y. Ouhdouch, S. Gopalakrishnan, and L. Kouisni. 2020. “Exploiting Biological Nitrogen Fixation: a Route towards a Sustainable Agriculture.” Plants 9(8): 1011. https://doi.org/10.3390/plants9081011
Wright, D.L., C. Mackowiak, and A. Blount. 2017. Cover crops. Publication# SS-AGR-66, University of Florida, IFAS Extension Service, Gainesville, Florida, USA.