Managing Fruit Splitting in Florida Citrus
Defining Pre-Harvest Fruit Splitting
Research on pre-harvest fruit splitting (PFS) often focuses on mandarins, sweet oranges, and lemons—the citrus cultivars most prone to exhibiting PFS—but other cultivars are not immune to the physiological disorder. PFS symptoms appear as a splitting of the fruit rind, usually passing through the stylar end. Three forms of cracking exist: radial, the most common (Figures 1A, B, and C); transverse (Figure 1D); and irregular (Figures 1E and F).
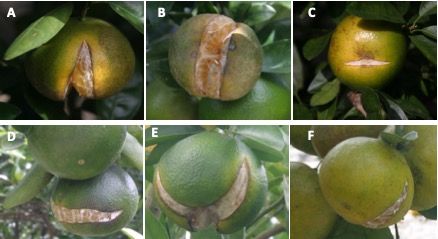
Credit: Krajewski et al. (2022)
Pre-harvest fruit splitting is not fruit creasing which appears as narrow sunken irregular grooves on the fruit. Fruit creasing is also known as albedo splitting or albedo breakdown, where albedo is the white part of the rind. Both PFS and fruit creasing can occur on the same fruit. If PFS is the only problem, though, then the flavedo distant from the crack in PFS-affected fruits will look normal and not have additional grooves. Fruit can also split when subjected to pressure during handling and transport; but the focus of this paper is on PFS, not these other issues.
Affected Cultivars
Though pre-harvest fruit splitting affects all cultivars, certain cultivars suffer from PFS more than others. Some orange cultivars had up to 30% of the fruits split, while 50% of certain mandarins were deemed unmarketable thanks to PFS. ‘Cleopatra’ rootstock had up to 40% of the fruits split, but on ‘Carrizo’ only up to 27% split. Under the right conditions, most cultivars will experience some PFS. These conditions begin with a defect that forms at flowering or shortly thereafter, splitting fruit when the fruit fill with juice faster than the peels can expand. New hybrids under development should be assessed for their risk of developing PFS.
While resulting in yield losses, split fruits also increase the need for grove sanitation. Split fruits are easily invaded by pathogens such as Alternaria alternata and Penicillium spp. (blue and green molds). Softer interior tissues are exposed when the fruit splits, and the exposed parts attract insects if the fruit was close to maturity. Surrounding fruits that have not split are then at greater risk of becoming infested or diseased because of the greater pest presence.
Underlying Mechanisms
PFS is initiated with damage to the stylar end of the fruit. Improper plant nutrition (see “Mitigating PFS” below) and unfavorable weather (see “Factors Affecting PFS Severity” below) causes developmental deformities during flower development through shortly after petal fall. Once initiated, the symptoms are expressed during periods of rapid expansion of the fruits, mostly when the fruits are past the cell-division stage.
The presence of a “navel” increases the risk of PFS, but fruits without navels may still have PFS. In flower anatomy, the style is a structure at the opposite end of the ovary from the stem. At some point in fruit maturation, the style falls off (abscises), leaving a scar. Fruits with abnormal scars, voids, or a complex rind structure have structurally weaker rinds relative to fruits with no voids and simple structures. Navels are a genetic mutation where a rudimentary second fruit is embedded at the stylar end. While more complex than fruits from cultivars without the mutation, there is a range of complexity even with navels. To illustrate this, Figure 2 shows three ‘Dancy’ mandarins from the same tree, displaying external and internal morphological differences between large and small stylar end openings. This inherent weakness was worsened if an “open hole at the pole of the oblate fruit” existed (Figure 2, B and C). The risk of PFS is also related to fruit shape: oblate (“flat”) fruit are at greater risk of developing PFS because the mechanical stress on their rinds is greater than on those of rounder fruit.
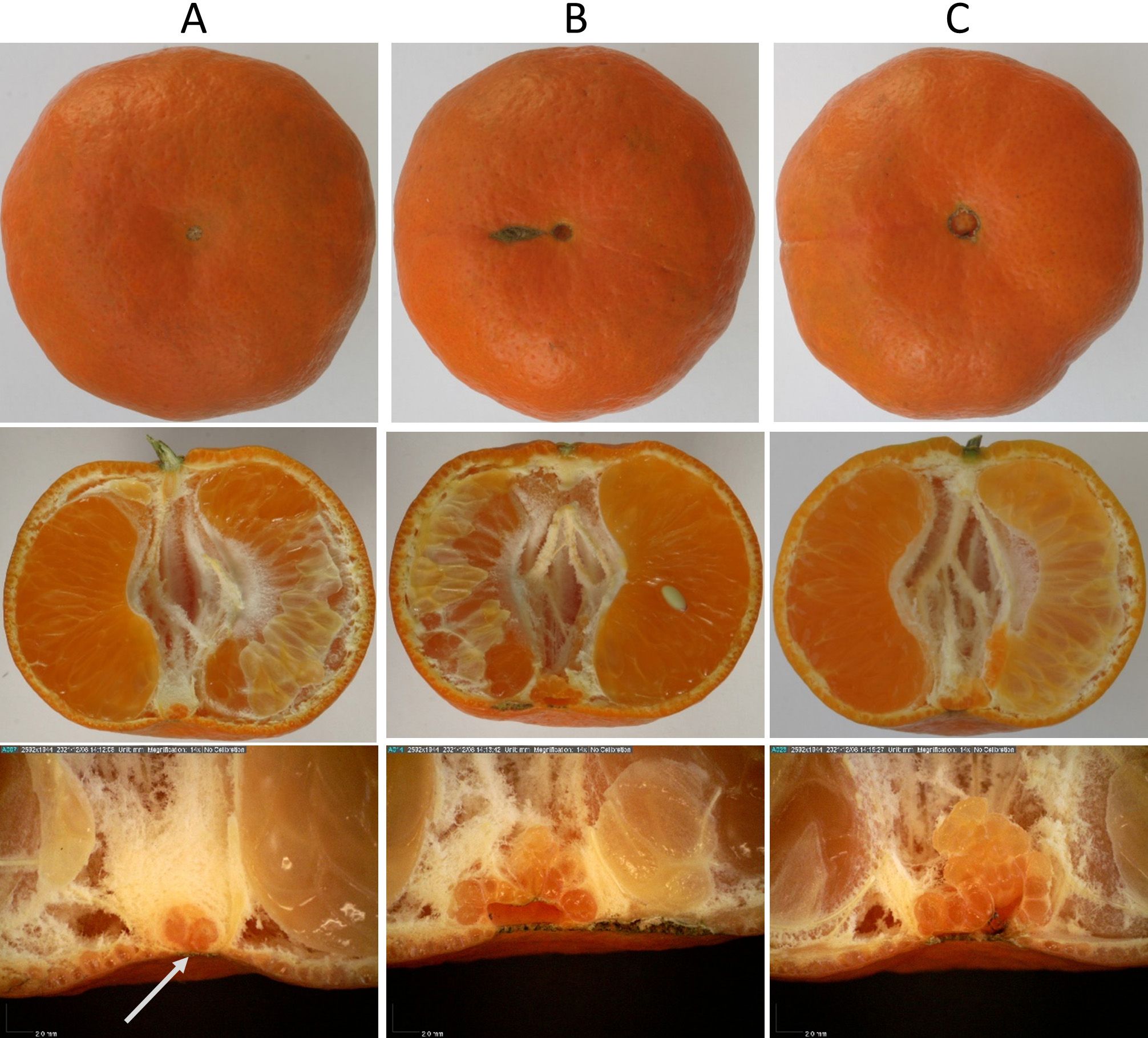
Credit: Krajewski et al. (2022)
Internal, mechanical stress increases at the poles of fruits that change shape from globose to oblate as they mature. This stress notably increases at the stylar end. Thus, the genetic factors that control fruit morphology influence overall susceptibility to PFS.
The rootstock can also affect the incidence of PFS. The reason appears to be the presence of wide xylem vessels in fruit peduncles of trees with some rootstocks; wide vessels enable a more rapid expansion of the fruits after release from some period of water stress.
Initiation
The risk for PFS was reported to be from flower bud formation through the period of style abscission of fruitlets. Since the cell-division stage defined the structural integrity of fruits, the occurrence of any stress on trees during this stage was critical in determining susceptibility to PFS. Any lesion at the stylar end of the rind could have been a starting point for fruit splitting. Key risk factors during bloom were high temperatures, low humidity, and high winds. Such conditions favored high vapor pressure deficits and result in damage to fruitlets. Once PFS was initiated, factors later in fruit development regulated the severity of symptom expression.
Symptom Expression
Fluctuating environmental factors (e.g., temperature, relative humidity, and soil moisture) that facilitate a rapid expansion of pulp relative to rind can result in PFS. A rapid influx of water into fruits transitioning from drought to flood conditions often triggers PFS, too, as can a spasm of growth from any cause. This disorder is expressed in some cultivars throughout the fruit growth and development period but is more commonly expressed during the cell enlargement and cell maturation stages. Crack location and shape are determined by mechanical stress intensity and rind resistance and plasticity.
Early signs of PFS (in stages I and II of fruit development) include small cracks or a localized yellowing of the fruit at the stylar end. As internal pressure builds, the cracks expand, often along the gap between two segments of the fruit. However, sometimes rinds split at the fruit’s equator. Such cracks may be transverse, but if longitudinal the crack eventually splits the stylar end. Stage III of fruit development can also be stressful on rinds as fruits achieve their final size and shape, the rind softening as part of maturation. In this stage, a rapid influx of water into the pulp increases the severity of splitting.
Factors Affecting PFS Severity
A rapid influx of water has already been mentioned as increasing PFS severity. However, having many fruits on the tree might buffer this effect. There is no generally accepted quantitative definition of low, light, normal, average, high, or heavy crop load. This makes it more difficult to assess the effect of crop load. However, it seems that a tree with few fruits usually produces oversized fruits that may more commonly split, whilst a tree with many fruits produces small, thin-skinned fruit with weaker rinds more predisposed to PFS. Avoiding the extremes reduces the risk of PFS expression.
If all else has failed (see the following sections), water management is the key to minimizing PFS. While rainfall is uncontrollable, changing the contours of the land at planting can manage where the water goes; and irrigation can be adjusted to make water deficits less extreme. Irrigation just before or after a major rain event is asking for trouble. Water management within the tree is easier with a dryer surface and wetter deeper soil layer.
Rind quality is critical in being able to resist the pressures from expanding pulp. The environmental factors that result in a thin rind include warm and humid conditions during stage I of fruit development. Fruit developing in full sun will be warmer and therefore have a greater tendency to split. The thickness and hardness are measures of rind strength. Thick, hard rinds are less likely to split.
In some cases, PFS damage fails to result in an open wound; the damaged flavedo can heal, sometimes leaving a longitudinal scar (Figure 3). These scars are typically aligned with intersegmental areas internally (Figure 3, C) and align with microscopic defects in the rind (Figure 3, D).
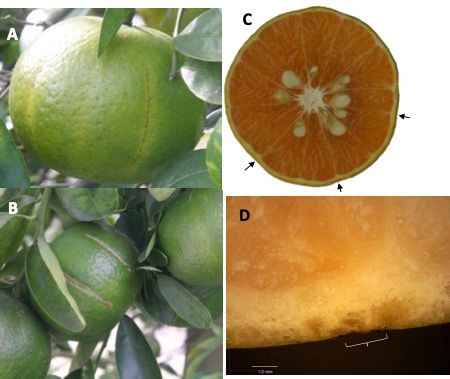
Credit: Krajewski et al. (2022)
Mitigating PFS
The best approach for mitigating PFS is early season nutrient management; this ensures that trees have the resources necessary to produce healthy fruits. The second-best approach is to manage fertilizer rates all through fruit development possibly with the application of plant hormones for the development of strong rinds. This must be consistent with a steady growth rate. This is achieved by frequent but small doses of fertilizer matched to the changing physiological needs of the fruits as they grow and ripen.
Fertilization to prevent PFS needs to start before bloom so that the plants have time to absorb the nutrients and move essential components to the developing buds. Plants should be monitored thereafter to adjust fertilization in support of strong rinds. Late-season fertilization can be reduced; fertilizing late in the season benefits little and risks inhibiting color break in fruits.
Boron
Boron application reduced cracking in lemon from 33% in the control to 23.7%. A 0.8% foliar boric acid spray reduced splitting from 52% in the control to 8.89% in mandarin. Boron may act synergistically with calcium applications. Boron is important in cell division, calcium mobility, carbohydrate metabolism and transport, nitrogen metabolism, and flowering and early fruit growth (Zekri and Obreza 2019). The existing literature is inconsistent in recommending when to apply boron. One study in India applied the element to lemons in May; another in Thailand, four months post fruit set in ‘Shogun’ mandarin. Both experiments reduced PFS.
Calcium
Calcium is best absorbed through the roots. Any factor reducing calcium transport (e.g., lowered vapor pressure deficit) during the critical stages of cell division and growth reduces Ca levels in the fruit and may result in PFS later if the conditions are right in fruit development. The year with the highest PFS in UF/IFAS CREC-CUPS (Citrus Research and Education Center – citrus under protective screen) coincided with the lowest calcium levels recorded in the leaves. Calcium levels in leaves showed a distinct seasonal variation with a peak in the middle or late flowering period (typically April) followed by a 2% (or greater) drop in Ca levels shortly thereafter (June–July) (Figure 4).
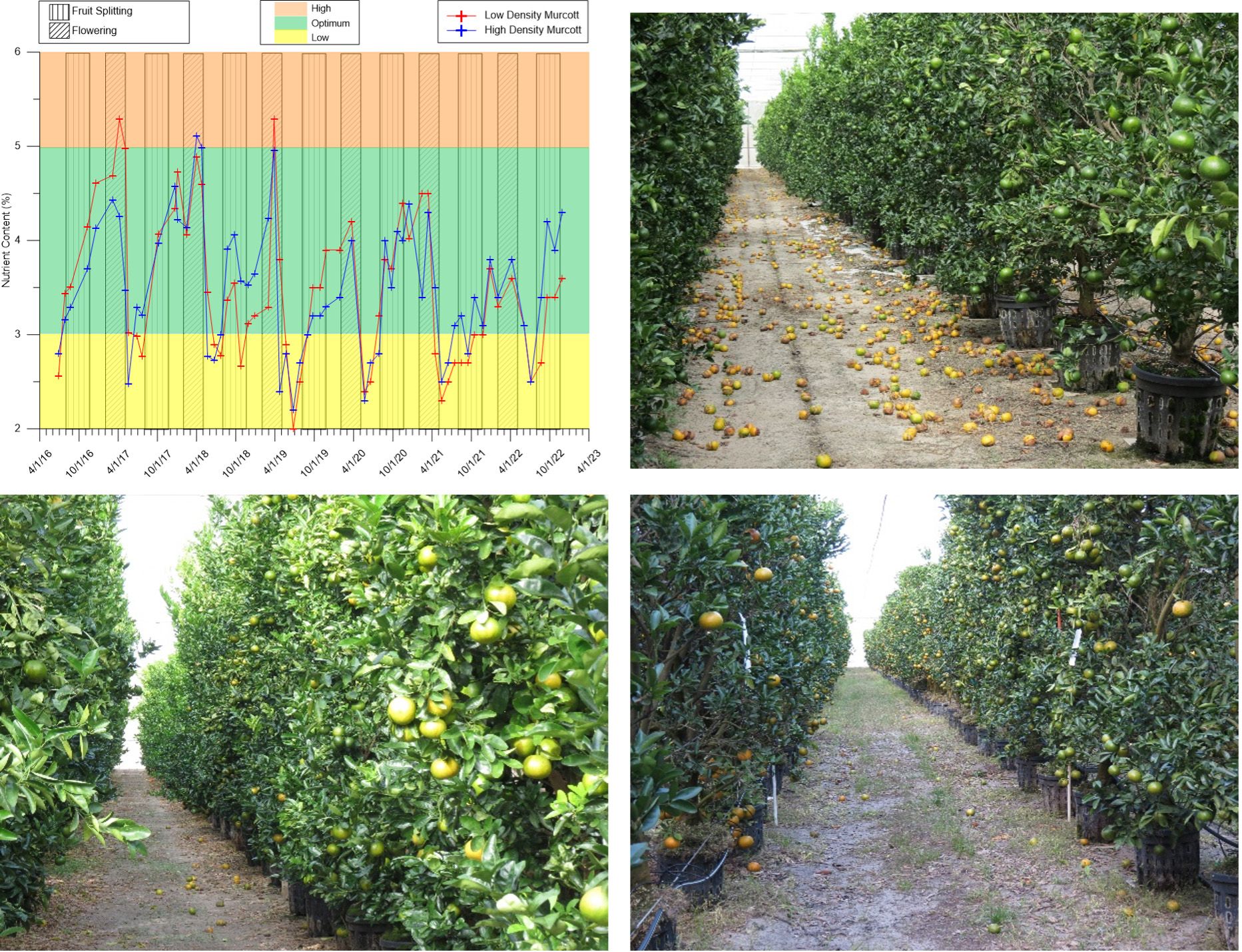
Credit: Arnold Schumann, UF/IFAS
One report showed no effect of calcium fertilization, but the application was at the start of the cell enlargement stage and therefore too late to be effective. Most studies show significant reductions in PFS following calcium applications even as foliar sprays. Tested products include calcium nitrate and calcium chloride. In one ‘Shogun’ mandarin trial, a 1% calcium chloride spray reduced splitting from 52% to 6%. The researchers ensured that calcium levels were sufficient at flowering and fruit set; the most effective applications occurred during these two stages because that is when plant demand for calcium is at its peak. The application to ‘Shogun’ mandarin at 4 months post-bloom (about 2/3 through stage II, cell enlargement) had a significant reduction in splitting, too. Despite this, fruit splitting was first observed 3 months post-bloom, thereby indicating that calcium has both a curative and preventative role.
Nitrogen
Nitrogen fertilization can increase fruit set and could thereby influence fruit size and rind thickness. However, no study has made any link between PFS and nitrogen fertilization.
Phosphorus
High levels of phosphorus result in fruits with thinner, smoother rinds; thinner rinds are more prone to splitting. However, there is no direct field data linking elevated phosphorus with increased PFS despite frequent claims of the association.
Potassium
Potassium fertilization in Florida-grown ‘Hamlin’ reduced PFS. Potassium fertilization of ‘Valencia’ in South Africa suggested that leaf potassium levels below 0.8% had an increased propensity for PFS, whilst K levels above 0.9% reduced PFS. Vashisth, Dewdney, and Diepenbrock (2021) recommended foliar potassium nitrate spray (4%) after the end of physiological fruit drop to increase fruit size at harvest. Low potassium and calcium in rinds appeared to be related to an increased cracking rate. A 6% potassium nitrate application at full bloom reduced splitting from 7% to 2% and was as effective as an application when fruits were 19/32" to 29/32" (1.5 cm to 2 cm).
A later application was more effective than an earlier application at lower fertilization rates (4% and 2%). When applied at the end of petal fall, rates as low as 1% reduced PFS from 33% to 20% in ‘Page’ mandarin. Potassium sulfate has also been used to reduce PFS problems. The fertilization appeared to increase rind hardness as potassium levels increased. Potassium also increased amino acid levels in rinds, and the amino acids could function in osmoregulation.
Zinc
Zinc fertilization has not been directly tied to PFS. While there are no studies examining the effect of zinc fertilization on PFS, there are some metabolic studies that suggest zinc could have benefits.
Water
While no correlation has been shown between rainfall patterns and PFS, several studies have shown that irrigation schedules affect PFS. This link is strongest in sand-dominated soils. However, tree water status is an interaction between soil moisture, soil type, rootstock, daylight, temperature, wind, and humidity. According to irrigation studies, the critical factor is an abrupt increase in available water that results in fruit rapidly filling with water. The resulting expansion splits fruit.
Plant Growth Regulators
The benefits of increasing fruit set by foliar GA3 applications must be weighed against the risk of increased PFS. Heavy flowering is physiologically stressful during the sensitive period of cell division. Thus, increasing bloom to increase crop load may have the opposite effect if many fruit split. While plant growth regulators can be applied to increase flowering and fruit set thereby indirectly affecting PFS, they can also be applied to manage PFS. There are four products that have been tested outside of Florida for their effect on PFS: gibberellic acid, 2,4-D; 1-naphthaleneacetic acid (NAA); and 3,5,6-trichloro-2-piridil oxyacetic acid (3,5,6-TPA). However, only gibberellic acid and 2, 4-D are registered for use in Florida consistent with managing PFS.
Gibberellic acid applications reduce cracking, though the timing of the application is critical. Treatments at full bloom are sometimes less effective than treatments to small fruits (< 1 inch diameter). While gibberellic acid applications can protect against several other rind defects as well as PFS, such applications may delay color development in ripening fruits. Internal quality is unaffected, but the fruits look greener. The benefits from gibberellic acid applications are affected by dose, diluent pH < 8, crop phenology, time of day, and adjuvants.
2,4-dichlorophenoxyacetic acid (2,4-D) is also effective at reducing pre-harvest fruit splitting. Applications at the end of petal fall may be more effective than applications at full bloom or at the end of the June fruit drop (northern hemisphere). 2,4-D increases rind thickness and strength, and the proportion of fruits with closed stylar ends. However, it also increases rind coarseness. 2,4-D can be combined with products like potassium nitrate or gibberellic acid. While the combination of 2,4-D and gibberellic acid was somewhat better than either alone, gibberellic acid alone was more effective than 2,4-D alone.
Summary
PFS is linked to citrus fruit morphology and early fruit development. Proper fruit development from flower initiation through the cell division stage is the best prevention for PFS. Genetic traits like fruit shape, a navel, or a hollow space beneath the stylar end increase the chance of PFS problems. Proper nutrition and the application of growth regulators can significantly reduce fruit splitting. As part of a good fertilizer program, it is necessary to know what nutrients are deficient in the plant. The definition of deficient changes depending on the growth needs of the plant. For PFS management one needs to anticipate fertilizer needs because it may be too late by the time one analyses plant tissue, finds a deficiency, schedules a fertilizer application, and the deficient nutrient gets into the plant. Key nutrients are calcium, potassium, and boron. Excess phosphorus is likely a problem, though like most nutrients more research is needed to better understand the relationships with all aspects of plant performance. Soil properties affect the bioavailability of nutrients and should be considered before applying fertilizer. Despite an ideal nutritional program, developing fruits can be damaged by hot, dry, windy conditions.
If problems are caught early, the application of plant growth regulators can minimize later problems. Gibberellic acid is applied in early fruit development to close the stylar end and increase rind strength. When used alone, gibberellic acid seemed to be most effective. However, additional benefits are seen with the addition of a potassium fertilizer or 2,4-D. The application timing and dose are critical to achieving the desired result and avoiding undesirable side effects. Other plant growth regulators should be researched more carefully in Florida to understand their benefits and risks.
There are several other factors that can influence PFS and are under grower control. Fruits exposed to the full sun are more likely to split. The grower controls this when they prune the trees. Opening the canopy to increase light penetration also increases fruit exposure to sunlight. This might also happen as branches bend under the weight of a large crop of fruit. Due to source-sink relationships, an overabundant fruit load will stress a tree and likely increase PFS. However, an unusually small fruit load will result in excessively large fruits which also have a greater risk of cracking. While PFS can cause considerable fruit loss, the problem is manageable through plant nutrition management and several agronomic practices. Application timing is critical, and one should anticipate problems by understanding the growers needs of their trees based on data gathered in previous years.
Recommendation
Little can be done to protect the current crop once fruit start to split. In these cases, water management to maintain a uniform moisture level will help; if potassium levels in the plant are low, K fertilization helps, too.
Keep records of grove management practices and fruit split. If PFS gradually increases each year or you suddenly lose many fruits, then take remedial action to protect next year’s crop. Ensure that plant calcium levels are very high pre-bloom; calcium levels will drop 2 or more percentage points shortly after flowering. Splitting may occur more often if Ca gets into the “deficient” range, but remember that this only initiates the problem. (Some yields with very low Ca may have low PFS because conditions are not right for symptom expression that year.) Maintain sufficient boron (>36 ppm in leaves), and potassium (>1.2% in leaves). Gibberellic acid can be applied at or post-bloom to reduce PFS. 2, 4-D can also be applied to reduce PFS, following label directions.
Funding
This research was funded by the National Institute of Food and Agriculture (grant 2018-70016-27387). Any opinions, findings, conclusions, or recommendations expressed in this publication are those of the author(s) and do not necessarily reflect the view of the U.S. Department of Agriculture. The use of product names is only for accurate reporting of methods and is not an endorsement of those products over other similar products.
References
Krajewski, A., T. Ebert, A. Schumann, and L. Waldo. 2022. “Pre-Harvest Fruit Splitting of Citrus.” Agronomy 12 (7): 1505. https://doi.org/10.3390/agronomy12071505
Vashisth, T., M. Dewdney, and L. Diepenbrock. 2021. “Understanding Physiological Fruit Drop of Citrus.” Citrus Industry: Tip of the Week. https://citrusindustry.net/2021/06/29/understanding-physiological-fruit-drop-of-citrus/#:~:text=By%20Tripti%20Vashisth%20and%20Lisa,resources%20into%20non%2Dharvested%20fruit
Zekri, M., and T. Obreza. 2019. “Boron (B) and Chlorine (Cl) for Citrus Trees.” EDIS 2019 (8). https://edis.ifas.ufl.edu/publication/SS619