This factsheet provides a comprehensive overview of Histosols, also known as organic soils, in Florida, covering the basic definition, distribution, factors influencing soil formation, and key chemical, physical, and biological properties. It also discusses classification, land use, and management practices. The target audience includes agricultural producers, Extension agents, state and local agencies, decision-makers, researchers, students, and interested Florida citizens.
What are Histosols?
Histosols, derived from the Greek word “histos” meaning “tissue,” represent a distinct soil order classified within the United States Soil Taxonomy system, predominantly consisting of organic matter (Figure 1). Histosols typically contain more than 20%–30% organic matter by weight and have a thickness exceeding 40 cm (Soil Survey Staff 2022). These Histosols are also commonly known as “muck” or “peat” soils depending on the degree of organic matter decomposition.
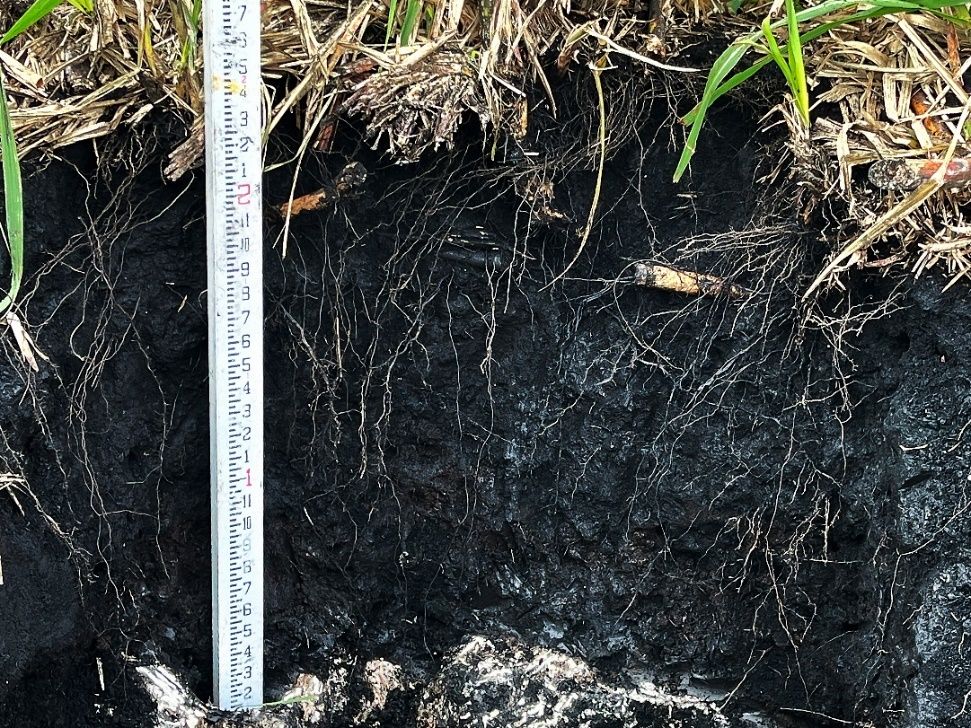
Credit: Xue Bai and Noel Manirakiza, UF/IFAS
Histosols Distribution
Global Distribution
Histosols cover approximately 4.23 million km2 worldwide, constituting roughly 3% of the Earth's total soil resources (Shi et al. 2024). These soils are distributed across all major climate zones on all continents, with their most extensive presence found between latitudes 50° north and 70° north (Figure 2). Specifically, 35% of Histosols are distributed throughout the Siberian region of Russia, 32% are in Canada, and roughly 7% are in Northern Europe (Conchedda and Tubiello 2020). These regions are characterized by cold and humid climates, which slow the decomposition of carbon-based materials, leading to the accumulation of organic matter. Conversely, Histosols are relatively limited in the Southern Hemisphere, where nearly all land mass falls outside of the latitudes necessary for optimal organic soil accumulation (Kats 1966).
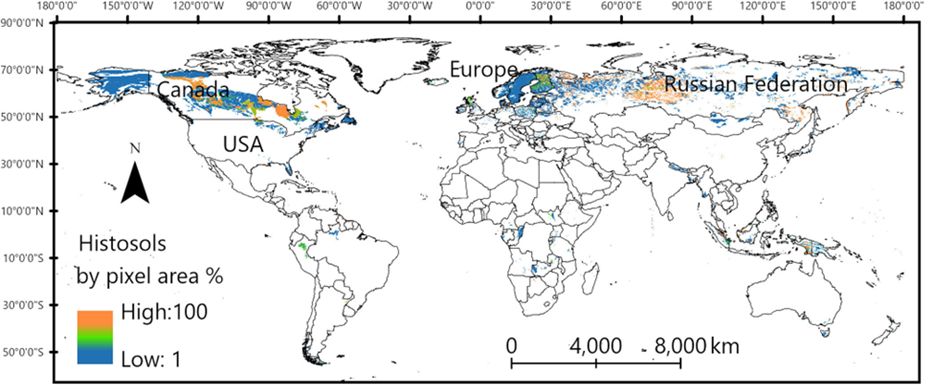
Credit: Adapted by Xue Bai, UF/IFAS; © FAOSTAT 2020 Harmonized World Soil Database v1.2. https://www.fao.org/soils-portal/data-hub/soil-maps-and-databases/harmonized-world-soil-database-v12/en/
Distribution of Histosols in the United States and Florida
The United States has approximately 7.7% of the world’s Histosols, with the most extensive Histosols being found in the Lake Agassiz plain in north-central Minnesota (Wright et al. 1992) (Figure 3). Florida has approximately 16,000 km2 (6,200 square miles) of Histosols, accounting for 17% of the total US deposits. Of that amount, 75% are located in the Everglades, thus forming the largest contiguous organic soil area in the continental United States (Kolka et al. 2016; Mylavarapu et al. 2016). These soils are crucial in supporting unique ecosystems and storing significant carbon reservoirs.
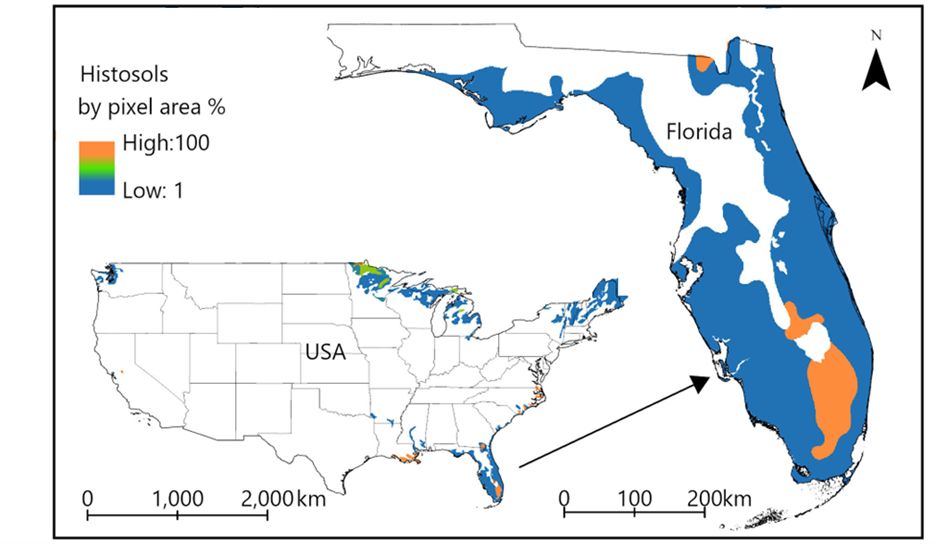
Credit: Adapted by Xue Bai, UF/IFAS; © FAOSTAT 2020 Harmonized World Soil Database v1.2. https://www.fao.org/soils-portal/data-hub/soil-maps-and-databases/harmonized-world-soil-database-v12/en/
Formation of Histosols in Florida
Florida Histosols are formed by the deposition of organic material under anaerobic wetland conditions. The organic matter as parent material, subtropical to tropical climate, decayed vegetation, time, and low-lying topography are five essential factors in the formation of Histosols (Figure 4).
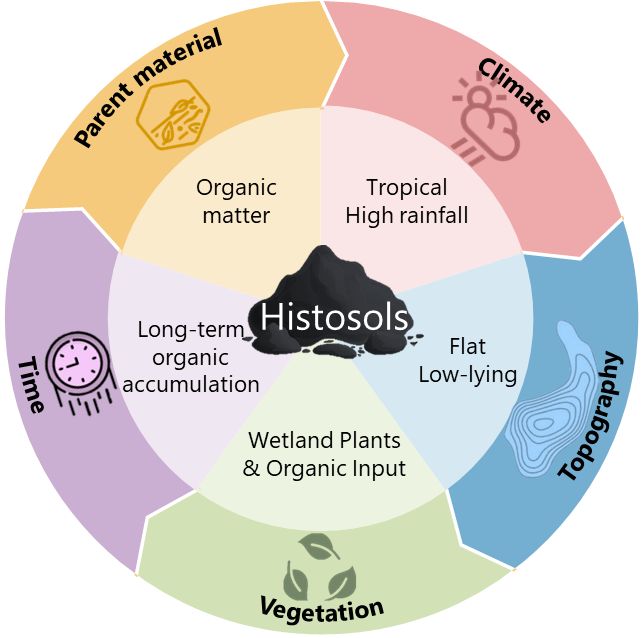
Credit: Xue Bai and Jehangir Bhadha, UF/IFAS
Parent Materials
Unlike other soils developed from mineral materials, most Florida Histosols are formed from the organic parent material deposited by wetland plants. The anaerobic conditions in wetlands slow down organic matter decomposition, leading to significant accumulation and storage of organic matter. Consequently, the accumulated soil inherits the unique properties of its parent material. For instance, the acidity carried by the organic parent material also makes the accumulated organic soil horizon acidic.
Climate
South Florida has a subtropical to tropical climate, characterized by year-round warm temperatures that extend the growing season of crops and provide a continuous source of plant material, contributing to the formation of Histosols. Moreover, the high annual rainfall provides waterlogged conditions conducive to accumulating organic material. However, the intensifying effects of climate change, such as rising temperatures and extreme precipitation events, will significantly impact Histosol formation through the decomposition of organic matter, wetland water levels, and vegetation types.
Vegetation
Decayed vegetation serves as the direct source of Histosols' parent material, and the type of vegetation influences many of the soil's physical and chemical properties. In Florida, various peat types are associated with plant species (Shober and Obreza 2009). For instance, organic soils in the Florida Everglades, stretching from Lake Okeechobee to nearly the end of the Florida peninsula, originate from partially decomposed sawgrass leaves and roots (Bhadha et al. 2020).
Topography
The topography dictates specific locations of Histosols within the landscape. In Florida, Histosols commonly form in flat, low-lying, and waterlogged areas like wetlands, swamps, floodplains, and coastal zones (FDEP 2017). Additionally, the varying elevation of the underlying bedrock and its control of water flow has shaped the diverse plant communities in the Florida Everglades for millennia, further impacting the formation of Histosols (Inglett and Inglett 2013; Dreschel et al. 2018).
Time
The formation of Histosols began approximately 3,200 years ago when a relatively quick sea-level rise following the last glacial period slowed dramatically to an average rise of 4 cm/100 years in south Florida (Wanless et al. 1994). This slow rise allowed many shallow marine environments, such as marsh vegetation, to continue building to keep up with sea level and sometimes higher, leading to the accumulation of organic parent material at 8 cm/100 years and the formation of Histosols before human intervention (McDonwell et al. 1969). However, according to data from the Florida Climate Center (FSU), the rate of sea-level rise has been accelerating in recent decades as ocean temperatures warm, and the rate of global mean sea-level rise since 1993 has been approximately 3.4 mm per year. Rapid sea-level rise can lead to saltwater intrusion, impacting wetland vegetation types and hydrological conditions, which could further alter the rate and process of organic matter accumulation and the formation of Histosols (Watanabe et al. 2019; Reddy et al. 2021).
Properties of Typical Histosols in Florida
Soil properties are influenced by the soil’s formation processes, and variability observed in Florida organic soils can be attributed to the aforementioned soil-forming factors. This section offers additional information about the morphological, physical, hydrological, and biochemical properties of Florida's Histosols and the factors that led to the following soil characteristics.
Morphology
Soil morphology is everything that can be seen and felt about the soil in the field, such as soil color, soil texture, roots, and so forth. Florida Histosols typically feature a dark, organic-rich surface horizon known as the O (organic) horizon, reaching a depth of 41–137 cm (16–54 inches) (Zelazny and Carlisle 1974; Snyder 2005). Florida organic soils are lightweight and porous due to their high organic matter content (> 80%). Plant root systems in Histosols are typically shallow, fibrous, and well-adapted to the waterlogged conditions prevalent in wetland environments.
Physical Properties
The physical properties of soil play a key role in determining soil suitability for agricultural, environmental, and engineering uses. Histosols in Florida have a relatively low bulk density of approximately 0.47g/cm3 (Wright and Hanlon 2019) and high porosity. Bulk density and porosity are influenced by the degree of decomposition of organic materials. As organic materials undergo higher levels of decomposition, bulk density generally increases and porosity decreases. The physical properties of Histosols, particularly bulk density and porosity, impact the soils’ compressibility and reduce their load-bearing capacity, thereby restricting the soils’ suitability for engineering applications such as the construction of roads, buildings, or other structures.
Hydrology
Hydrology is related to the retention, movement, distribution, and management of water in the soil. Histosols have a great capacity to absorb and retain water, a characteristic directly influenced by the degree of organic material decomposition (Boelter 1969). In saturated conditions, water content can reach 100% (by volume) in less decayed material but falls below 80% in fully decayed peat. When drained, fresh or slightly decayed peat lets water pass through quickly, but this process slows down as the peat decomposes further (Kolka et al. 2011).
Chemical/Biological Properties
Biochemical properties play an important role in soil fertility. Florida Histosols, with organic content ranging from 35.1% to 86.2%, create acidic conditions and have a high cation exchange capacity (CEC) due to organic acids from decomposing plants. This high CEC enables these soils to retain and supply nutrients efficiently and to release plant-available forms of nitrogen (N), an essential nutrient for crops in the Everglades Agricultural Area (EAA), resulting in less or no need for nitrogen fertilizer applications (Everett 1983; Sanchez and Porter 1994; McCray et al. 2023).
Classification of Histosols in Florida
The decomposition of organic matter can be broadly categorized into three defined states: little decomposed (fibric), moderately decomposed (hemic), and highly decomposed (sapric). Each decomposition state predominantly exists within a particular soil profile, giving rise to the three most common suborders for organic soils: Fibrists, Hemists, and Saprists. Fibrists represent over 75% fiber content of soil volume after rubbing. Saprists represent <17% fiber content of soil volume after rubbing and consist of almost completely decomposed plant remains. Hemists have five percentages in the intermediate range.
At the most specific level of soil taxonomy, six soil series have been recognized in the EAA, including Torry muck, Terra Ceia muck, Pahokee muck, Lauderhill muck, Okeelanta muck, and Okeechobee muck (Figure 5). All of these series belong to the suborder of Saprists, indicating a high degree of decomposition of the surface horizon. Since the classification of Histosols in the EAA is partly based on depth to limestone bedrock, soil series in the region have undergone changes, with a continuous decrease in soil thickness over past decades. Figure 5 depicts the changes in distribution in Histosol series in the EAA between 1973 and 2024. Compared with 1973, around 15% of Pahokee muck (36–51 inches) in the southern EAA has subsided, changing the soil classification to the shallower Lauderhill muck (20–36 inches) soil series.
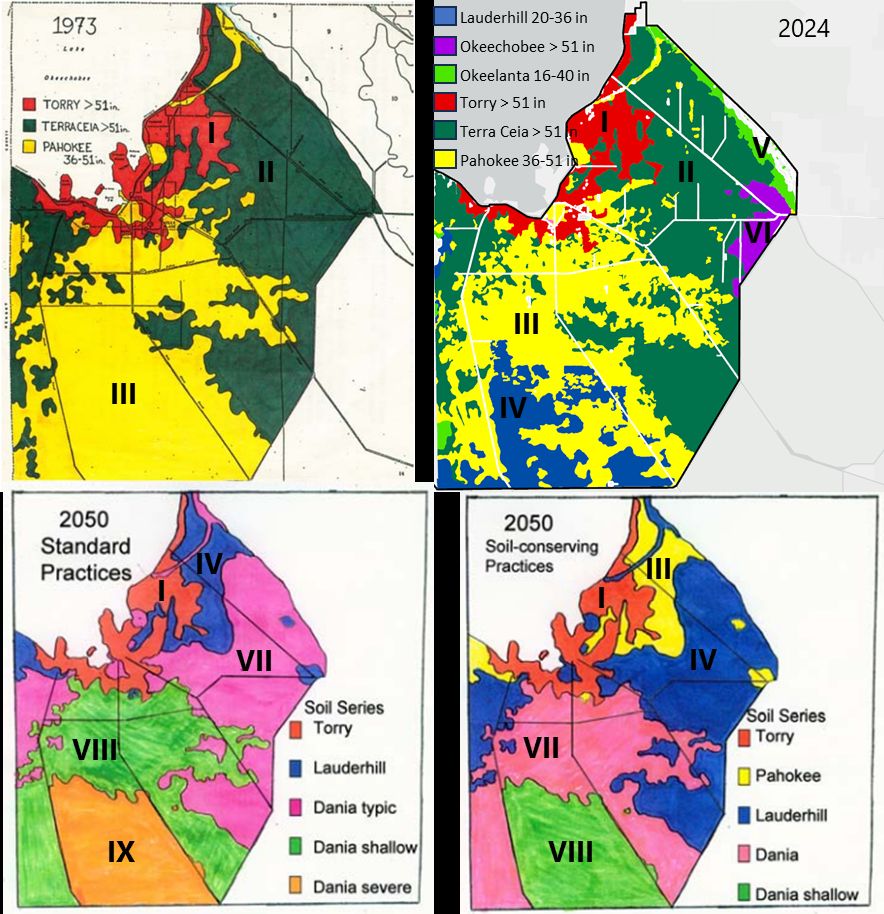
Credit: Xue Bai and Jehangir Bhadha, UF/IFAS
However, soil depletion has become increasingly prevalent in recent years as soil depths in the EAA continue to decline. Soil depth has not yet had a discernible impact on production, which can be attributed to the ample availability of nutrients and water in the region, coupled with advancements in agricultural technologies such as stringent soil testing for nutrient recommendations and effective plant breeding that address the constraints posed by shallow soils (Bai et al. 2024). As no detectable soil depth threshold has been identified at which crop production begins to decline, the risk of abrupt loss in sugar production remains a significant concern in the region and across the United States if current practices continue. Snyder (2005) projected that continuing traditional agricultural methods will lead to the disappearance of the Pahokee series (36–51 inches) and much of the Terra Ceia series (> 51 inches) by 2050. Nearly 70% of the EAA would then have soils only suitable for water-tolerant crops like rice, certain sugarcane varieties, and some types of pasture grass. Similarly, Rodriguez et al. (2020) predicted that Histosols thickness would decrease from 60 cm in 2018 to 25 cm in 2068 under a business-as-usual scenario. However, if soil-conserving practices are implemented (Glaz 1995), then nearly half the EAA soils should be suitable for all currently grown crops, and most of the remaining soils should retain suitability for water-tolerant crops through 2050.
Land Use and Management on Histosols in Florida
Agriculture
Histosols play a significant role in agriculture with well-managed water tables. In Florida, approximately 12% of the Histosols are utilized for crop production, constituting the EAA. During the 1930s and 1940s, vegetable-dominated farming in the EAA required strict water control due to the crops' intolerance of flooded soils. However, with the shift to sugarcane in the 1960s, practices changed to allow more water storage, as sugarcane is more flood tolerant (Wright and Snyder 2009). Approximately 85% of the 2,800 km2 (1,000 square miles) of EAA farmland is used for sugarcane cultivation, contributing to over half of US sugarcane production (USDA-NASS 2024a). As a consequence of soil depletion and additional challenges such as natural disasters and volatile global markets (Glaz and Gilbert 2007), sugar production in the region has faced significant stress. Since 1999, Palm Beach County, which includes the UF/IFAS Everglades Research and Education Center (EREC) and much of the EAA, has lost over 30%—nearly 36,000 hectares—of its sugarcane area (USDA-NASS 2023b).
To the south of the EAA region, the land has changed from grasslands in 2004 to increasingly herbaceous wetlands in 2011 and 2023 (Figure 6). Such conversions can be attributed to various factors, including water management practices and environmental conservation efforts. Restoration projects like the Comprehensive Everglades Restoration Plan (CERP) aim to restore natural water flow to wetlands, which may lead to the conversion of previously drained grasslands into wetland ecosystems.
Rotating rice cultivation during the sugarcane summer fallow period has gained popularity in recent decades. This practice slows down soil loss via microbial organic matter oxidation, controls pests, and introduces additional economic benefits. Approximately 11,912, 15,000, and 24,000 acres of rice were planted in 2008, 2011, and 2023, respectively (Bhadha et al. 2022).
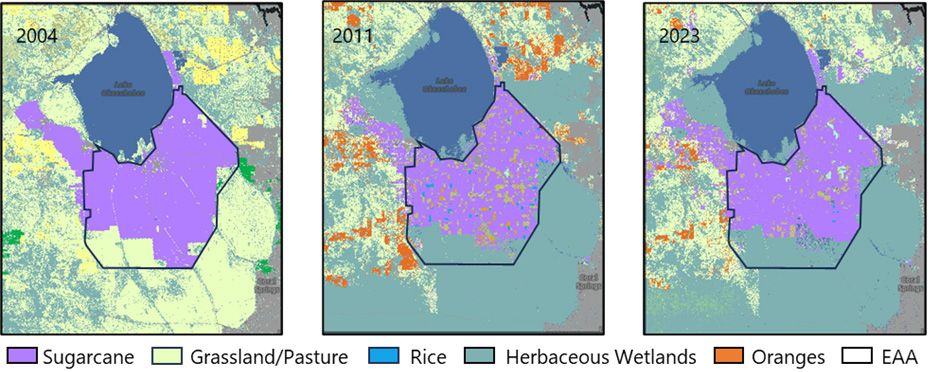
Credit: Xue Bai and Jehangir Bhadha, UF/IFAS; Adapted from USDA-NASS (2023b)
Environmental and Ecological Functions
Histosols are primarily found in wetland areas and contribute to a range of environmental and ecological functions within their local ecosystems. Typically, Histosols can perform wetland-related functions, such as providing habitat for wildlife, controlling and buffering floodwater, recharging groundwater, cycling nutrients through biogeochemical processes, and purifying wastewater. Furthermore, Histosols serve as a sink for terrestrial carbon. Proper soil management practices such as controlled water table management, minimizing soil disturbance, and promoting the growth of native vegetation are essential for minimizing greenhouse gas emissions, as they help to maintain and even increase the carbon sequestration potential of Histosols (Kolka et al. 2011).
Conclusion
Histosols, characterized by their high organic matter content, play vital roles in Florida's agriculture and ecological diversity. These soils have accumulated over thousands of years in anaerobic wetland environments and are inseparable from the region's favorable climate, topography, and vegetation conditions. However, challenges, such as accelerated sea-level rise and soil loss caused by oxidation, constantly threaten the stability of these soils. Sustainable land use and management practices, such as conservation tillage and groundwater level management, are crucial for maintaining the productivity and ecological benefits of these soils.
References
Bai, X., S. J. Smidt, Y. Fan, et al. 2024. “Farming Shallow Soils: Impacts of Soil Depth on Crop Growth in the Everglades Agricultural Area of Florida, USA.” Field Crops Research 316: 109523. https://doi.org/10.1016/j.fcr.2024.109523
Bhadha, J. H., A. L. Wright, and G. H. Snyder. 2020. “Everglades Agricultural Area Soil Subsidence and Sustainability: SL311/SS523, rev. 3/2020.” EDIS 2020 (2). https://doi.org/10.32473/edis-ss523-2020
Bhadha, J. H., L. Trotta, D. Cavazos, and M. VanWeelden. 2024. “Trends in Rice Production and Varieties.” EDIS 2024 (4). https://doi.org/10.32473/edis-ss653-2024
Boelter, D. H. 1969. “Physical Properties of Peats as Related to Degree of Decomposition.” Soil Science Society of America Journal 33 (4): 606–609. https://doi.org/10.2136/sssaj1969.03615995003300040033x
Conchedda, G., and F. N. Tubiello. 2020. “Drainage of Organic Soils and GHG Emissions: Validation with Country Data.” Earth System Science Data Discussions. https://doi.org/10.5194/essd-2020-202
Cox, S., D. Lewis, S. McCollum, M. Bledsoe, and R. Marrotte. 1988. Subsidence Study of the Everglades Agricultural Area. USDA, Soil Conservation Service.
Everett, K. R. 1983. “Chapter 1 Histosols.” In Developments in Soil Science, edited by L. P. Wilding, N. E. Smeck, and G. F. Hall. Vol. 11, Part B. Elsevier. https://doi.org/10.1016/S0166-2481(08)70612-3
Florida Department of Environmental Protection (FDEP). 2017. Digital Elevation Model 2009. ArcGIS Hub. Last updated November 20, 2018. https://hub.arcgis.com/datasets/FDEP::digital-elevation-model-2009
Glaz, B. 1995. “Research Seeking Agricultural and Ecological Benefits in the Everglades.” Journal of Soil and Water Conservation 50 (6): 609–612. https://www.jswconline.org/content/50/6/609
Glaz, B., and R. Gilbert. 2007. “Sugarcane Variety Census: Florida 2005: SS AGR 268/SC083, 6/2007.” EDIS 2007 (19). https://doi.org/10.32473/edis-sc083-2007
Inglett, P. W., and K. S. Inglett. 2013. “Biogeochemical Changes During Early Development of Restored Calcareous Wetland Soils.” Geoderma 192: 132–141. https://doi.org/10.1016/j.geoderma.2012.07.009
Kats, N. Y. 1966. “Bogs of the Subantarctic and Cold Temperate Zone of the Southern Hemisphere.” Soviet Soil Science 2: 180–188.
Kolka, R., S. D. Bridgham, and C. L. Ping. 2016. “Soils of Peatlands: Histosols and Gelisols.” In Wetland Soils: Genesis, Hydrology, Landscapes, and Classification, edited by M. J. Vepraskas and C. L. Craft. CRC Press/Lewis Publishing. https://research.fs.usda.gov/treesearch/49906
Kolka, R. K., M. C. Rabenhorst, and D. Swanson. 2011. “Histosols.” In Handbook of Soil Sciences Properties and Processes, edited by P. M. Huang, Y. Li, and M. E. Sumner. 2nd ed. CRC Press.
McCray, J. M., H. S. Sandhu, R. W. Rice, and D. C. Odero. 2023. “Nutrient Requirements for Sugarcane Production on Florida Muck Soils." SS-AGR-226. University of Florida Institute of Food and Agricultural Sciences. https://edis.ifas.ufl.edu/publication/SC026
Mylavarapu, R., W. Harris, and G. Hochmuth. 2016. “Agricultural Soils of Florida: SL441/SS655, 10/2016.” EDIS 2016 (9). https://doi.org/10.32473/edis-ss655-2016
Reddy, K. R., J. Hu, O. Villapando, R. K. Bhomia, L. Vardanyan, and T. Osborne. 2021. “Long‐Term Accumulation of Macro‐and Secondary Elements in Subtropical Treatment Wetlands.” Ecosphere 12 (11): e03787. https://doi.org/10.1002/ecs2.3787
Rodriguez, A. F., S. Gerber, and S. H. Daroub. 2020. “Modeling Soil Subsidence in a Subtropical Drained Peatland. The Case of the Everglades Agricultural Area.” Ecological Modelling 415: 108859. https://doi.org/10.1016/j.ecolmodel.2019.108859
Sanchez, C. A., and P. S. Porter. 1994. “Phosphorus in the Organic Soils of the EAA.” In Everglades Agricultural Area (EAA): Water, Soil, Crop, and Environmental Management, edited by A. B. Bottcher and F. T. Izuno. University Press of Florida.
Shi, J., W. Liu, R. Li, et al. 2024. “Research Progress in the Field of Peatlands in 1990–2022: A Systematic Analysis Based on Bibliometrics.” Land 13 (4): 549. https://doi.org/10.3390/land13040549
Shober, A. L., and T. A. Obreza. 2009. “Soils & Fertilizers for Master Gardeners: Soil Formation in Florida: SL274/MG455, rev. 1/2009.” EDIS 2009 (1). https://doi.org/10.32473/edis-mg455-2009
Snyder, G. H. 2005. “Everglades Agricultural Area Soil Subsidence and Land Use Projections.” In Soil and Crop Science Society of Florida Proceedings 64. https://original-ufdc.uflib.ufl.edu/AA00067243/00049
Soil Survey Staff. 2022. Keys to Soil Taxonomy. 13th ed. USDA-Natural Resources Conservation Service.
USDA-NASS (National Agricultural Statistics Service). 2024a. “2023 State Agriculture Overview.” Accessed June 5, 2024. https://www.nass.usda.gov/Quick_Stats/Ag_Overview/stateOverview.php?state=FLORIDA
USDA-NASS (National Agricultural Statistics Service). 2023b. “CroplandCROS.” Accessed December 12, 2024.https://croplandcros.scinet.usda.gov/
Wanless, H. R., R. W. Parkinson, and L. P. Tedesco. 1994. “Sea Level Control on Stability of Everglades Wetlands.” In Everglades: The Ecosystem and Its Restoration, edited by S. Davis and J. C. Ogden. CRC Press.
Watanabe, K., K. Seike, R. Kajihara, S. Montani, and T. Kuwae. 2019. “Relative sea‐level change regulates organic carbon accumulation in coastal habitats.” Global Change Biology 25 (3): 1063–1077. https://doi.org/10.1111/gcb.14558
Wright, H. E., B. Coffin, and N. E. Aaseng. 1992. The Patterned Peatlands of Minnesota. University of Minnesota Press.
Wright, A. L., and G. H. Snyder. 2010. “Soil Subsidence in the Everglades Agricultural Area: SL311/SS523.” EDIS 2010 (2). https://doi.org/10.32473/edis-ss523-2009
Zelazny, L. W., and V. W. Carlisle. 1974. “Physical, Chemical, Elemental, and Oxygen‐Containing Functional Group Analysis of Selected Florida Histosols.” In Histosols: Their Characteristics, Classification, and Use, edited by A. R. Aandahl. Vol. 6. https://doi.org/10.2136/sssaspecpub6.c6
Further Reading
Bhadha, J. H., R. Khatiwada, S. Galindo, N. Xu, and J. Capasso. 2018. “Evidence of Soil Health Benefits of Flooded Rice Compared to Fallow Practice.” Sustainable Agriculture Research 7 (4): 31–41. https://doi.org/10.5539/sar.v7n4p31
Davis, J. H. 1943. The Natural Features of Southern Florida, Especially the Vegetation, and the Everglades. Florida Geological Survey: Bulletin 25. https://ufdc.ufl.edu/UF00000488/00001/images
Davis, J. H. 1946. The Peat Deposits of Florida, Their Occurrence, Development and Uses. Florida Geological Survey: Bulletin 30. https://ufdc.ufl.edu/UF00000489/00001/images
McDowell, L. L., J. C. Stephens, and E. H. Stewart. 1969. “Radiocarbon Chronology of the Florida Everglades Peat.” Soil Science Society of America Journal 33 (5): 743–745. https://doi.org/10.2136/sssaj1969.03615995003300050033x
Morris, D. R., R. A. Gilbert, D. C. Reicosky, and R. W. Gesch. 2004. “Oxidation Potentials of Soil Organic Matter in Histosols Under Different Tillage Methods.” Soil Science Society of America Journal 68 (3): 817–826. https://doi.org/10.2136/sssaj2004.8170
Northwest Alliance for Computational Science and Engineering. 2022. “PRISM Climate Data.” PRISM Climate Group, Oregon State University. Accessed May 10, 2023. https://prism.oregonstate.edu
Stephens, J. C. 1969. “Peat and Muck Drainage Problems.” Journal of the Irrigation and Drainage Division 95 (2): 285–306. https://doi.org/10.1061/JRCEA4.0000650
Wright, A. L., and E. A. Hanlon. 2019. “Organic Matter and Soil Structure in the Everglades Agricultural Area.” SL301. University of Florida Institute of Food and Agricultural Sciences. https://edis.ifas.ufl.edu/publication/SS514