This article is brought to you by the faculty and students of University of Florida’s Institute of Food and Agricultural Science, Cervidae Health Research Initiative. Please visit the CHeRI website at https://wec.ifas.ufl.edu/cheri/ for more information about farmed deer health and management.
Introduction
White-tailed deer are susceptible to infection by gastrointestinal parasites of numerous taxonomic groups, including helminths (roundworms, tapeworms, and flukes) and protozoans (coccidia and flagellates). Although not all parasite infections cause clinical disease, some gastrointestinal parasite species can affect the health and productivity of infected hoofstock, causing economic loss and necessitating management action. An understanding of parasite life cycles, host susceptibility, and environmental factors affecting parasite transmission is critical to developing effective parasite management strategies.
This publication will serve as a guide to common parasites of farmed white-tailed deer in Florida and will provide farmed deer producers with recommendations for diagnosis and management of gastrointestinal parasites.
Impacts of Parasitism on Deer Production
Little information is available to quantify production loss in North American white-tailed deer operations, but gastrointestinal parasitism presents a significant challenge to captive cervid industries in Europe and New Zealand (Haigh et al. 2002; Castillo-Alcala et al. 2007), and to small ruminant production systems globally (Stromberg and Gasbarre 2006; Verocai et al. 2020). Additionally, disease and death associated with gastrointestinal parasite infection have been reported in free-ranging white-tailed deer (Forrester et al. 1974a and 1974b; Davidson et al. 1980; Prestwood and Pursglove 1981).
Gastrointestinal parasites can cause both clinical and subclinical disease in infected livestock. Infection with parasitic gastrointestinal roundworms reduces production by suppressing host appetite, impairing gastrointestinal function, and altering protein metabolism (Fox 1997). Even in the absence of clinical disease, the effects of chronic parasitism can be dramatic; for example, in pair-feeding studies, the appetite of sheep with chronic, subclinical gastrointestinal parasite infections was 15% to 20% lower than in animals without parasite burdens (Coop et al. 1982; Sykes et al. 1988). Production of trophy bucks for hunting preserves is a primary goal of many deer farming operations, and antler development in male white-tailed deer is affected considerably by nutrition (French et al. 1956; Magruder et al. 1957; Brown 1990; Demarais and Strickland 2011). Thus, depressed appetite and reduction in voluntary feed intake due to gastrointestinal parasitism could prevent bucks from achieving their genetic potential for antler growth. Additionally, there is some suggestion that parasites may be associated with the development of antler asymmetry in reindeer (Folstad et al. 1996), though the effect of parasites on antler symmetry in white-tailed deer has not been evaluated.
Common Parasites of Florida White-tailed Deer
Farmed white-tailed deer are affected by many of the same parasite taxa that infect traditional ruminant livestock (sheep, goats, and cattle) (Foreyt 1981; Kingston 1981). Common gastrointestinal parasites of white-tailed deer can be divided into four major groups: roundworms (nematodes), tapeworms (cestodes), flukes (trematodes), and coccidia (protozoans).
Roundworms
Gastrointestinal roundworms are the most frequently encountered and economically significant parasites of livestock (Stromberg and Gasbarre 2006). White-tailed deer are parasitized by a diversity of roundworms, but only a few of these roundworms are known to cause disease. Of the 32 species of gastrointestinal roundworms that have been found in white-tailed deer (Prestwood and Pursglove 1981), 21 of these species have been reported in Florida (Forrester 1992). Though infection with gastrointestinal roundworms is nearly ubiquitous among ruminants including white-tailed deer, infection intensity among free-ranging white-tailed deer is generally low, and resulting disease is rare or mild (Prestwood and Pursglove 1981). However, two roundworm species, the barberpole worm (Haemonchus contortus) and Strongyloides papillosus,are known to cause disease in both farmed and free-ranging white-tailed deer.
Haemonchus contortus has a direct life cycle with free-living intermediate stages and parasitic adult stages. Adult worms produce eggs that are excreted with host feces. Eggs develop in the environment and hatch into first-stage larvae (L1). These larvae feed on bacteria in feces and develop into second-stage larvae (L2). Second-stage larvae develop into highly motile third-stage larvae (L3) measuring 0.65–0.74 mm in length (Zajac and Conboy 2012). These L3 larvae are the infective life stage of H. contortus. L3 larvae migrate vertically from feces to herbaceous vegetation and are ingested by browsing deer. L3 larvae are predominantly found at the base of vegetation, but may migrate to the top of blades of grass when wet environmental conditions promote L3 motility (Silva et al. 2008; Santos et al. 2012). About 48 hours after ingestion, the L3 larvae molt and become fourth-stage larvae (L4). L4 larvae take blood meals from the abomasum (true stomach) and mature into hematophagous (blood-feeding) adult worms measuring 11–27 mm (Prestwood and Pursglove 1981; Lichtenfels et al. 1994).
Survival of free-living larvae on pastures is determined by environmental factors, most importantly temperature and moisture (Waller 2006; Besier et al. 2016). In warm temperate regions such as Florida, spring and early summer conditions are especially permissive to rapid H. contortus hatch rates and survival of free-living larvae on pasture (Besier et al. 2016). In moist environments, H. contortus hatch rates increase with temperature, reaching the optimal temperature range for egg development and larval survival between 68˚F –86˚F (Besier et al. 2016).
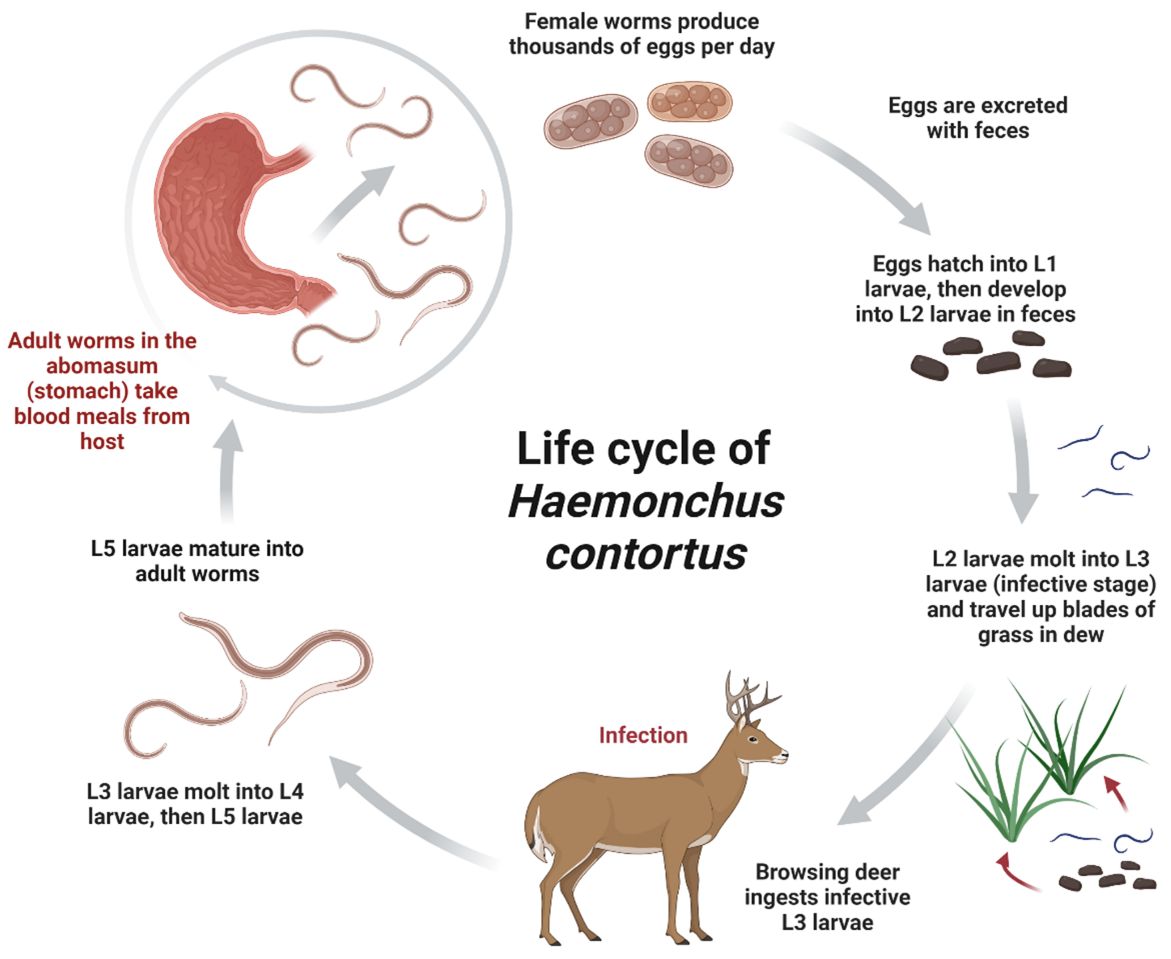
Credit: S. L. Cottingham, CHeRI. Created with BioRender.com
Adult Haemonchus contortus are aggressive blood-feeders, and each adult can consume 0.05 mL of host blood per day (Alba-Hurtado and Muñoz-Guzmán 2013). Disease caused by H. contortus infection (“haemonchosis”) is a result of moderate to severe blood loss due to feeding worms. Clinical signs of haemonchosis include mucosal pallor, weakness, emaciation, stunted growth, enlarged lymph nodes, and submandibular edema (“bottle jaw”) (Prestwood and Pursglove 1981). Deer younger than 6 months of age are most likely to be affected (Prestwood and Pursglove 1981). Haemonchus contortus have a wide geographic distribution but are found at greater prevalence and intensity in white-tailed deer in coastal plains in the southeastern United States (Davidson et al. 1980).
Strongyloides papillosus worms have a unique life history where the life cycle can be completed with or without a host, and in which only female worms are parasitic (Prestwood and Pursglove 1981). Adult female worms living within a host can produce eggs in the absence of male worms (“parthenogenesis”). Eggs are shed with the host feces and either develop directly into infective larvae or differentiate into male and female adults that undergo sexual reproduction to produce eggs that hatch into first-stage larvae (L1), then undergo two molts to become infective third-stage larvae (L3). Deer may become infected by Strongyloides papillosus by ingesting food or water contaminated with L3 larvae (Prestwood and Pursglove 1981). Additionally, L3 larvae are capable of penetrating unbroken skin and infecting new hosts. Fawns may become infected before birth via intrauterine transmission or shortly after birth by consuming L3 larvae shed in the milk of an infected doe (Forrester et al. 1974a; Prestwood and Pursglove 1981).
Infection with Strongyloides does not seem to cause disease (“strongyloidosis”) in free-ranging white-tailed deer due to its uncommon occurrence and low infection intensity in this population (Prestwood and Pursglove 1981). However, outbreaks of strongyloidosis can be a significant cause of mortality in captive white-tailed deer fawns in Florida (Forrester et al. 1974b, 1974a). Clinical signs of strongyloidosis include diarrhea, weakness, dehydration, and weight loss (Prestwood and Pursglove 1981). Death often occurs 12–36 hours after the appearance of clinical signs (Forrester et al. 1974b; Pursglove et al. 1976; Prestwood and Pursglove 1981).
Tapeworms
Throughout their range, white-tailed deer serve as intermediate or definitive hosts for six tapeworm species. Three taxa of tapeworms, Moniezia spp., Taenia hydatigena, and Taenia omissa have been reported in white-tailed deer in Florida (Forrester 1992). White-tailed deer serve as a definitive host for Moniezia sp. and an intermediate host for T. hydatigena and T. omissa (Foreyt 1981; Forrester 1992).
White-tailed deer can become infected with Moniezia spp. following ingestion of free-living orbatid mites containing cysticercoids, the infective intermediate stage of this tapeworm (Figure 2). Moniezia cysticercoids develop into adults in the small intestines of the host. Adult Moniezia spp. shed gravid proglottids that are excreted in the feces. Once in the environment, Moniezia spp. oncospheres are ingested by the species’ intermediate host, orbatid mites found in soil. Once ingested, the oconocpheres infect the orbatid mite’s digestive system. The oncospheres develop into infective cysticercoids within the orbatid mite, and the life cycle continues when a deer ingests the infected orbatid mite (Niilo 1969; Foreyt 1981). Infections of white-tailed deer by Moniezia sp. may occur incidentally on pasture grazed by both deer and livestock when deer consume orbatid mites that have been infected by Moniezia tapeworm shed in the feces of infected domestic livestock (Foreyt 1981). White-tailed deer serve as intermediate hosts of Taenia spp. and can become infected by ingesting vegetation contaminated with Taenia eggs (Figure 2). The eggs containing hexacanth embryos hatch in the deer’s digestive tract, penetrate the wall of the digestive tract, and travel through the hepatic parenchyma before emerging from the surface of the liver into the peritoneal cavity. The hexacanth embryos then develop into cysticerci, an intermediate larval tapeworm stage, and encyst in the host’s muscle tissue. The life cycle of Taenia spp. tapeworms is completed when intermediate host tissue containing cysticerci is consumed by the definitive host, a carnivore, particularly wild and domestic canids. Taenia mature into adults in the intestines of infected carnivores and shed gravid proglottids into the feces. The life cycle of Taenia continues when a white-tailed deer ingests vegetation contaminated with carnivore feces containing Taenia eggs (Niilo 1969).
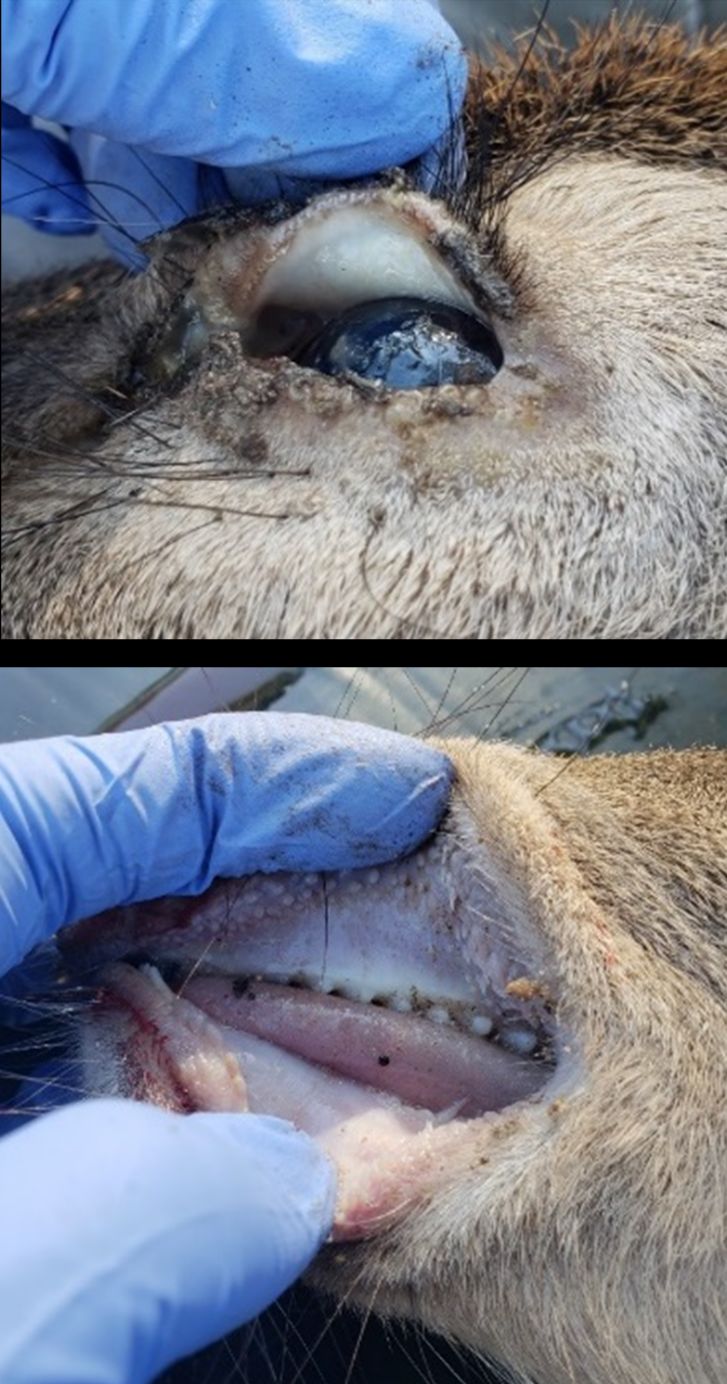
Credit: S. L. Cottingham, CHeRI
Infection of white-tailed deer by Moniezia spp., T. hydatigena, and T. omissa is uncommon and infection is considered innocuous in white-tailed deer (Foreyt 1981), though migration of Taenia hexacanth embryos may cause damage to the liver and other organs (Cheatum 1951; Prestwood et al. 1976).
Flukes
Infection by six different species of flukes have been recorded in white-tailed deer, but the giant liver fluke (Fascioloides magna) and the rumen fluke (Paramphistomum liorchis) are the only species known to infect white-tailed deer in Florida (Foreyt et al. 1977; Forrester 1992). Giant liver flukes and rumen flukes have similar indirect life cycles (Figure 3). The eggs of both fluke species are shed in the feces of infected deer. Once in the environment, the eggs develop and hatch in water into a free-swimming miracidium. The miracidium penetrates the tissue of an aquatic snail, developing and multiplying into many free-swimming cercariae. The cercariae attach to vegetation and form a protective cyst, becoming metacercariae, the infective stage of the giant liver fluke and rumen fluke. Metacercariae encysted on vegetation are ingested by browsing deer. The metacercariae of giant liver flukes excyst and migrate to the liver where they mature into adults (Figure 5), while the metacercariae of rumen flukes excyst and mature in the small intestine. Infection with giant liver flukes and rumen flukes is more common in moist sites due to the presence of the aquatic snail intermediate host necessary to the parasite life cycle (Pursglove et al. 1977). Giant liver fluke prevalence is higher in Florida than in the rest of the southeast United States, and three enzootic zones have been identified in the southeastern Panhandle, east-central Florida, and the southern tip of the Florida peninsula (Forrester 1992). Infections with rumen fluke are widely distributed throughout the state (Dinaburg 1939; Prestwood et al. 1970; Harlow and Jones 1965; orrester 1992; Forrester and Wright 1989).
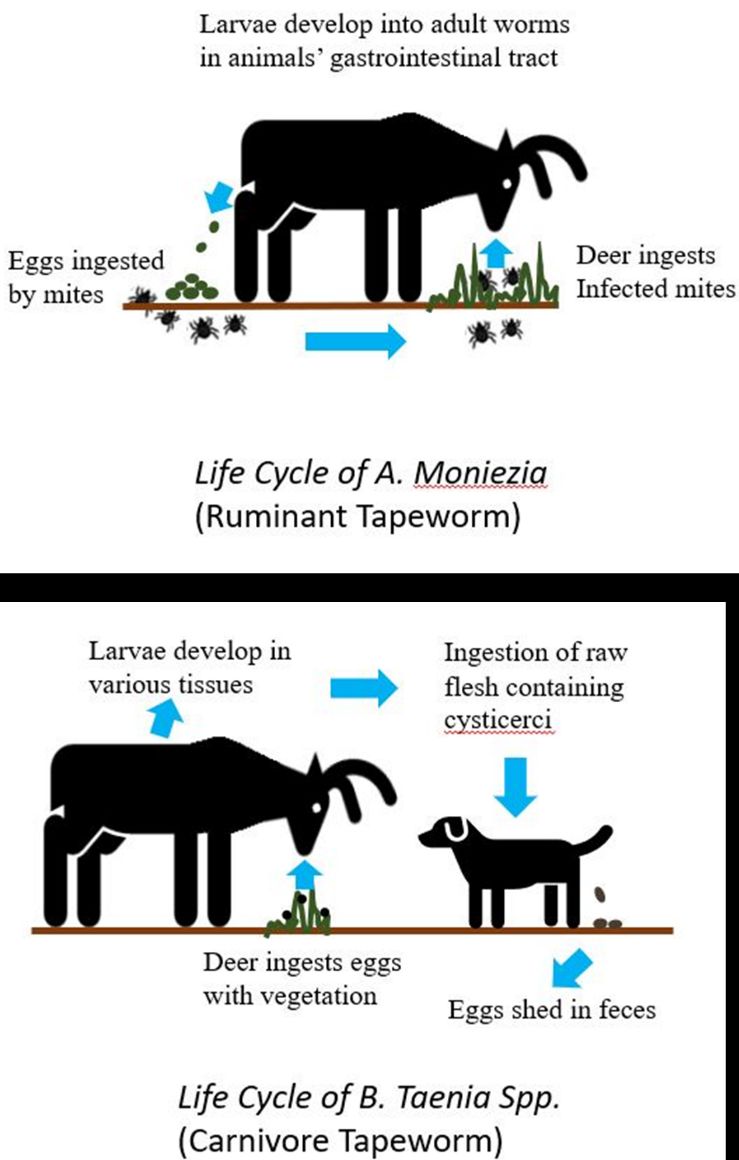
Credit: J. M. Campos-Krauer, CHeRI
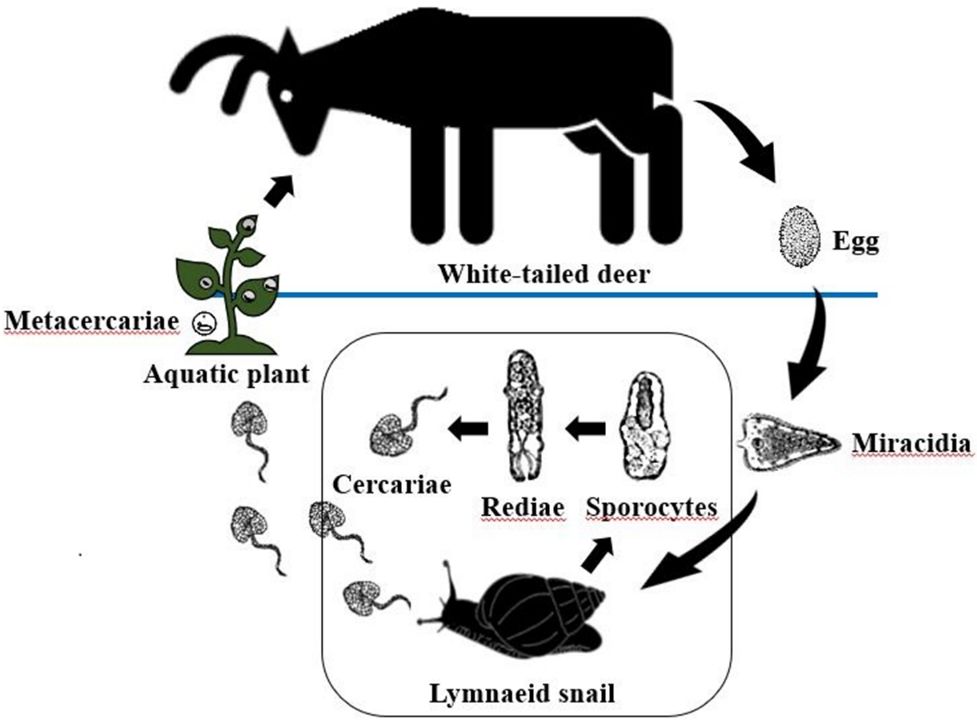
Credit: J. M. Campos-Krauer, CHeRI
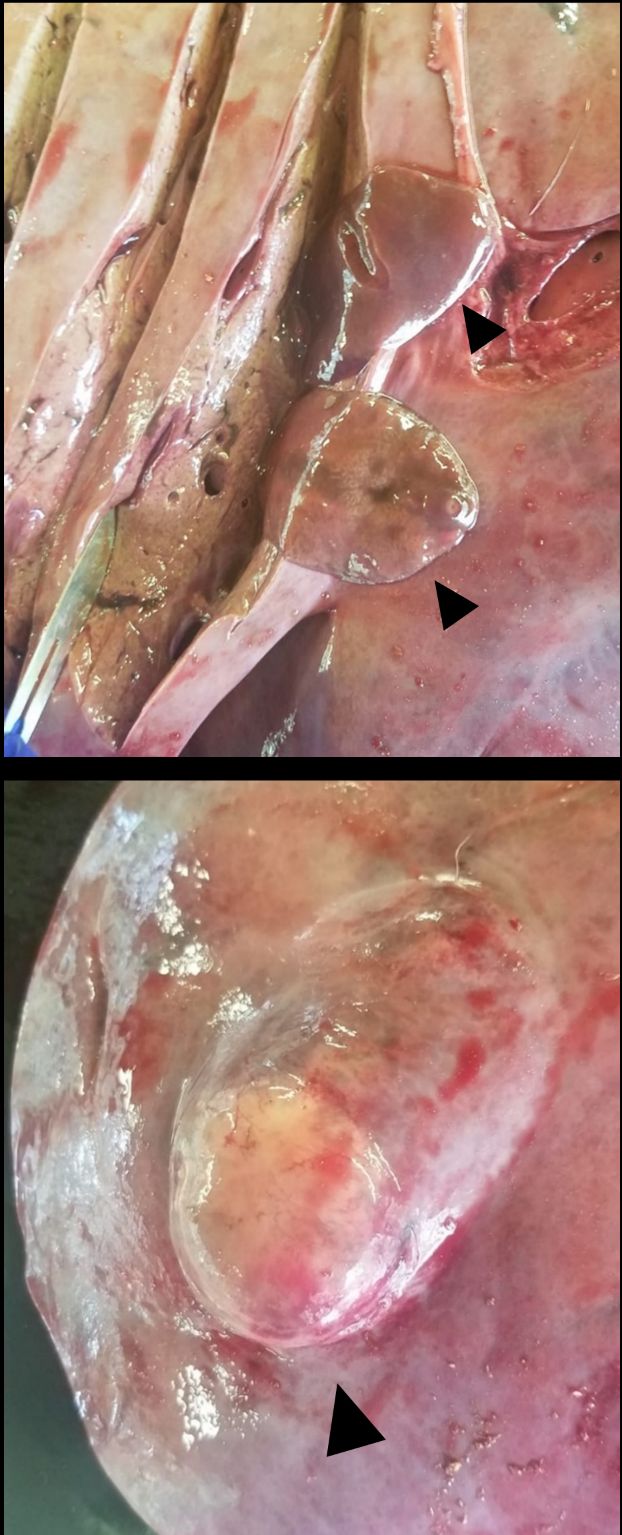
Credit: J. L. Smith, CHeRI
Giant liver fluke and rumen fluke generally cause little harm to otherwise healthy deer, thoughmigration of immature giant liver fluke causes hemorrhage in the hepatic parenchyma, and juvenile stages of the rumen fluke may induce enteritis in the small intestine (Whitlock 1939).
Coccidia
Coccidia are single-celled protozoan parasites of the genera Isospora and Eimeria. Infections by four different species of coccidia (Eimeria odocoilei, E. virginianus, E. mccordocki, and E. madisonensis) have been recorded in white-tailed deer, and all have been found in Florida white-tailed deer (Kingston 1981; Forrester 1992). The life cycle of Eimeria is direct. Infection occurs through ingestion of sporulated Eimeria oocysts found in contaminated food, water, soil, or bedding. The wall of the oocyst is digested in the host’s gut, releasing sporozoites. Sporozoites penetrate the epithelial cells of the intestinal mucosa and undergo asexual reproduction within the host cell to produce numerous merozoites. The merozoites escape the host cell and infect surrounding cells, undergoing a second round of asexual reproduction. Second-generation merozoites continue to invade and rupture host cells, proliferating through asexual reproduction and eventually differentiating into male and female gametocytes. Gametocytes penetrate epithelial cells and undergo sexual reproduction, then rupture the host cell releasing oocysts into the intestine. The oocysts are passed with the feces and sporulate (develop sporozoites within oocysts) in the environment. These sporulated oocysts are the infective stage of the Eimeria life cycle (Kingston 1981).
Eimeria infections may be found in both sub-adult and adult deer, and infection is more common in animals confined at high densities. Clinical signs of disease caused by Eimeria infection (“coccidiosis”) include weight loss, watery yellow or greenish diarrhea, or blood and mucus in the feces (Kingston 1981). Mild infection may not produce observable signs of disease, but severe coccidiosis may be fatal (Levine 1961).
Diagnosing Infection
Infection by gastrointestinal parasites is essentially ubiquitous among grazing ruminant livestock, and it is generally assumed that stock are infected, even if fecal egg shedding is below the detectible threshold (Verocai et al. 2020). Diagnostic tests for gastrointestinal parasites are an important tool for assessing individual and herd health and for developing effective parasite management strategies. Microscopic fecal examination is the most commonly used technique to diagnose gastrointestinal parasite infection in livestock, including captive white-tailed deer (Verocai et al. 2020). Gastrointestinal parasites shed eggs, oocysts, and larvae in host feces, and these life stages can be detected and identified through microscopic examination. The eggs of some roundworms are indistinguishable by microscopic examination. To differentiate between species, a fecal sample may be cultured until eggs hatch into an identifiable larval stage. Additionally, identification and enumeration of adult worms collected during post-mortem examination may be used to identify gastrointestinal worms to species. Microscopic fecal examination, coproculture, and post-mortem parasite identification are services offered by veterinary diagnostic laboratories and many veterinary clinics. Contact diagnostic laboratories and veterinary clinics directly for sample submission requirements.
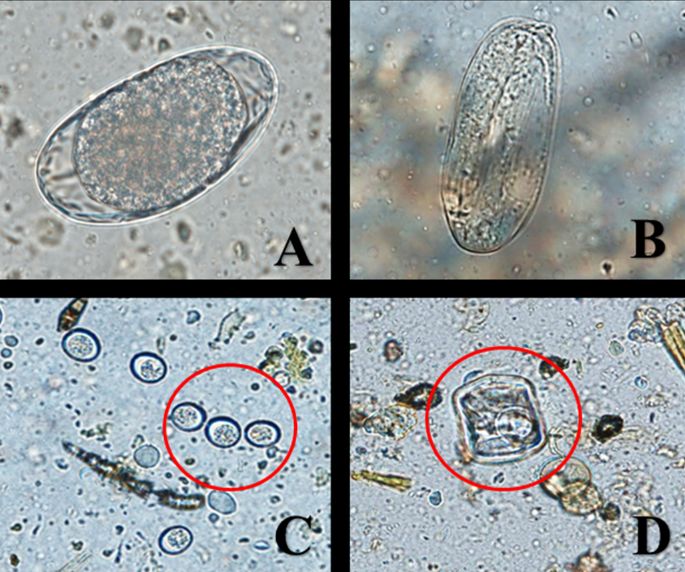
Credit: S. Cottingham, UF/IFAS
Integrative Parasite Management
The goal of parasite management is to break the transmission cycle and reduce parasite burdens below the threshold of economic loss. A combination of anthelmintic drugs (dewormers) and non-chemical management actions may be used to control GI parasites in farmed deer herds. Broad-spectrum anthelmintics are widely available and target both roundworms and flatworms (flukes and tapeworms). Modern broad-spectrum anthelmintics are grouped into five chemical categories: benzimidazoles, macrocyclic lactones, imidazothiazoles, spiroindoles, and amino-acetonitrile derivatives. Anthelmintics may be administered as an oral drench or feed top-dress, by injection, or as a topical application. Feed top-dresses are easy to administer and do not require direct handling of animals, but the exact dosage administered to each animal is difficult to determine. Oral drenches, injections, and topically administered products allow precise dosing but necessitate handling of individuals for treatment. No anthelmintics or anticoccidials are specifically authorized by the FDA for use in white-tailed deer, and off-label anthelmintic use should be approved and administered under the supervision of your herd veterinarian.
Anthelmintic resistance has been reported in parasites of all conventional livestock species and to drugs of all anthelmintic classes (Kaplan 2004). Drug resistance has not yet been reported in parasites of farmed white-tailed deer, however a survey of parasite control on Pennsylvania deer farms has raised concerns that parasite management practices promoting resistance may be common (Brooks and Jayarao 2008).
The distribution of gastrointestinal nematodes is “overdispersed” among hosts, meaning that parasites are non-randomly distributed in the host population, with most hosts carrying low parasite numbers and onlya small number of individuals carrying most of the parasites burden (Anderson and May 1978).
Rather than treating all animals in the herd, treating only disease-susceptible individuals and those animals shedding high numbers of eggs has proven effective in slowing the development of resistance (Kenyon et al. 2009). Producers should work with their veterinarians to develop criteria of physical indicators (body condition score, diarrhea, etc.) and fecal egg count values that must be met before anthelmintics are administered to an individual. This method of targeted selective deworming maintains a proportion of susceptible parasites in the “refugia” of untreated animals by removing the selection pressure of anthelmintic exposure. Evaluating the efficacy of anthelmintic treatments is critical to achieving sustainable parasite control in your deer. Fecal egg counts from a sample of your herd should be evaluated before anthelmintic treatment and again 14 days after treatment to evaluate efficacy. Consult your herd veterinarian to develop an anthelmintic treatment plan suitable for the parasite challenges facing your herd.
Non-chemical parasite management can complement anthelmintic treatment by reducing contamination of pastures by parasite eggs, thereby slowing reinfection of treated animals (Fox 2014). Slowing reinfection allows for reduced use of anthelmintic drugs, which in turn slows the development of anthelmintic resistance in parasite populations. Decreasing stocking rates and removing fecal material from pastures reduces the number of eggs, oocysts, and larvae to which deer are exposed. Additionally, mowing pastures may reduce exposure of browsing deer to infective larvae (Vegora 1960). Rotational grazing methods for parasite control rely on an understanding of regional parasite epidemiology and fall broadly into three categories: evasive, preventative, and diluting strategies (Barger 1997). Evasive strategies depend on moving the herd from a parasite egg-contaminated pasture to a “clean” pasture before the seasonal emergence of infective parasite larvae in the original pasture. Preventative strategies use anthelmintics to suppress fecal egg output until the level of infective larvae on the pasture has declined. Diluting strategies involve simultaneous grazing of deer alongside non-cervid livestock that are not susceptible to the same species of parasites. Concurrent grazing of multiple species with different parasite susceptibility can reduce the intensity of pasture contamination by deer parasites (Barger 1997).
Many forages in the natural diet of deer contain chemical compounds called tannins that may have natural anthelmintic effects (Hoste et al. 2006). However, the use of tannin-rich plants as deworming agents in white-tailed deer shows mixed results, with one study showing that tannin-supplemented diets did not affect fecal parasite loads, but were associated with decreased feed intake, reduced protein digestibility, and reduced weight gain (Chapman 2007). Another study found that the effect of dietary tannins on nutritional status and dietary intake varied by season and suggested that there may be substantial individual variation in preference for tannin-rich forages, with some individual deer selecting forages with up to 5% tannins (Chapman et al. 2010). As in other ruminant hoofstock species, the utility of tannins as feed additives in deer may be concentration dependent, with high tannin contents (>7%) causing undesirable production outcomes including decreased feed intake and decreased weight gain (Hoste et al. 2006).
Conclusion
Gastrointestinal parasites are a constant challenge to farmed white-tailed deer and effective, sustainable management is essential to maintaining herd health and preventing loss of production. Florida’s warm, humid climate promotes survival of parasites on pasture, and infection pressure can be high during spring and summer months. Microscopic fecal examination is a critical tool used to guide anthelmintic treatment and to evaluate and maintain the efficacy of deworming regimens. The timing of fecal egg counts and anthelmintic treatment may vary according to herd demographics, and targeted selective treatment should be used as part of a refugia-based control strategy. Non-chemical management techniques provide a valuable complement to anthelmintic deworming and may decrease the number of deworming treatments necessary in your herd. Consult your veterinarian to develop a plan to provide safe, effective, and sustainable parasite management for your deer herd.
Literature Cited
Alba-Hurtado, F., and M. A. Muñoz-Guzmán. 2013. "Immune Responses Associated with Resistance to Haemonchosis in Sheep." BioMed Research International 2013:1–11. https://doi.org/10.1155/2013/162158
Anderson, R. M., and R. M. May. 1978. "Regulation and Stability of Host-Parasite Population Interactions: I. Regulatory Processes." [Wiley, British Ecological Society]. Journal of Animal Ecology 47:219–247. https://doi.org/10.2307/3933
Barger, I. 1997. "Control by Management." Veterinary Parasitology 72:493–506. https://doi.org/10.1016/S0304-4017(97)00113-1
Besier, R. B., L. P. Kahn, N. D. Sargison, and J. A. Van Wyk. 2016. "Chapter Four - The Pathophysiology, Ecology and Epidemiology of Haemonchus contortus Infection in Small Ruminants." In: Advances in Parasitology, edited by R. B. Gasser and G. V. Samson-Himmelstjerna. Academic Press. pp. 95–143. https://www.sciencedirect.com/science/article/pii/S0065308X16300227. Accessed July 2020. https://doi.org/10.1016/bs.apar.2016.02.022
Brooks, J. W., and B. M. Jayarao. 2008. "Management Practices Used by White-Tailed Deer Farms in Pennsylvania and Herd Health Problems." American Veterinary Medical Association. Journal of the American Veterinary Medical Association 232:98–104. https://doi.org/10.2460/javma.232.1.98
Brown, R. D. 1990. "Nutrition and Antler Development." In: Horns, Pronghorns, and Antlers: Evolution, Morphology, Physiology, and Social Significance, edited by G. A. Bubenik and A. B. Bubenik. Springer, New York, NY. pp. 426–441. https://doi.org/10.1007/978-1-4613-8966-8_16 Accessed August 2020.
Castillo-Alcala, F., P. R. Wilson, W. E. Pomroy, and S. O. Hoskin. 2007. "A Survey of Anthelmintic Use and Internal Parasite Control in Farmed Deer in New Zealand." New Zealand Veterinary Journal 55:87–93. https://doi.org/10.1080/00480169.2007.36747
Chapman, G. A. 2007. "Forages and Tannin Supplementation for White-Tailed Deer. Thesis." University of Alberta, Edmonton, Alberta.
Chapman, G. A., E. W. Bork, N. T. Donkor, and R. J. Hudson. 2010. Effects of Supplemental Dietary Tannins on the Performance of White-Tailed Deer (Odocoileus virginianus)." Journal of Animal Physiology and Animal Nutrition 94 (1): 65–73. https://doi.org/10.1111/j.1439-0396.2008.00883.x
Cheatum, E. L. 1951. "Disease in Relation to Winter Mortality of Deer in New York." The Journal of Wildlife Management 15:216–220. https://doi.org/10.2307/3796614
Coop, R. L., A. R. Sykes, and K. W. Angus. 1982. "The Effect of Three Levels of Intake of Ostertagia circumcincta Larvae on Growth Rate, Food Intake and Body Composition of Growing Lambs." The Journal of Agricultural Science 98:247–255. https://doi.org/10.1017/S0021859600041782
Davidson, W. R., M. B. McGhee, V. F. Nettles, and L. C. Chappell. 1980. "Haemonchosis in White-Tailed Deer in the Southeastern United States." Journal of Wildlife Diseases 16:499–508. https://doi.org/10.7589/0090-3558-16.4.499
Demarais, S., and B. K. Strickland. 2011. "Antlers." In: Biology and Management of White-tailed Deer, edited by D. G. Hewitt. CRC Press, Boca Raton, Florida. pp. 125–126.
Dinaburg, A. G. 1939. "Helminth Parasites Collected from Deer, Odocoileus virginianus, in Florida." Proceedings of the Helminthological Society of Washington 6:102–104.
Folstad, I., P. Arneberg, and A. J. Karter. 1996. "Antlers and Parasites." Oecologia 105:556–558. https://doi.org/10.1007/BF00330020
Foreyt, W. J. 1981. "Trematodes and Cestodes." In: Diseases and Parasites of White-Tailed Deer, edited by W. R. Davidson, F. A. Hayes, V. F. Nettles, and F. E. Kellog. Miscellaneous Publication No. 7 of Tall Timbers Research Station, Tallahassee, FL.
Foreyt, W. J., W. M. Samuel, and A. C. Todd. 1977. "Fascioloides magna in White-Tailed Deer (Odocoileus virginianus): Observations on the Pairing Tendency." The Journal of Parasitology 63:1050–1052. https://doi.org/10.2307/3279843
Forrester, D. J. 1992. Parasites and diseases of wild mammals in Florida. University Press of Florida, Gainesville, FL. https://ufdc.ufl.edu/AA00025659/00001. Accessed July 2020.
Forrester, D. J., W. J. Taylor, and P. P. Humphrey. 1974. "Strongyloidiasis in White-Tailed Deer Fawns in Florida." Journal of Wildlife Diseases 10:146–148. https://doi.org/10.7589/0090-3558-10.2.146
Forrester, D. J., W. J. Taylor, and K. P. C. Nair. 1974. "Strongyloidosis in Captive White-Tailed Deer." Journal of Wildlife Diseases 10:11–17. https://doi.org/10.7589/0090-3558-10.1.11
Fox, M. T. 1997. "Pathophysiology of Infection with Gastrointestinal Nematodes in Domestic Ruminants: Recent Developments." Veterinary Parasitology 72:285–308. https://doi.org/10.1016/S0304-4017(97)00102-7
———. 2014. Overview of Gastrointestinal Parasites of Ruminants. In: Merck Veterinary Manual. https://www.merckvetmanual.com/digestive-system/gastrointestinal-parasites-of-ruminants/overview-of-gastrointestinal-parasites-of-ruminants?query=gastrointestinal%20parasites%20ruminants. Accessed July 2020.
French, C. E., L. C. McEwen, N. D. Magruder, R. H. Ingram, and R. W. Swift. 1956. "Nutrient Requirements for Growth and Antler Development in the White-Tailed Deer." [The Journal of Wildlife Management 20:221–232. https://doi.org/10.2307/3796954
Haigh, J. C., C. Mackintosh, and F. Griffin. 2002. "Viral, Parasitic and Prion Diseases of Farmed Deer and Bison." Rev Sci Tech OIE 21:219–248. https://doi.org/10.20506/rst.21.2.1331
Hoste, H., F. Jackson, S. Athanasiadou, S. M. Thamborg, and S. O. Hoskin. 2006. The Effects of Tannin-Rich Plants on Parasitic Nematodes in Ruminants." Trends in Parasitology 22 (6): 253–261. https://doi.org/10.1016/j.pt.2006.04.004
Kaplan, R. M. 2004. "Drug Resistance in Nematodes of Veterinary Importance: A Status Report." Trends in Parasitology 20:477–481. https://doi.org/10.1016/j.pt.2004.08.001
Kenyon, F., A. W. Greer, G. C. Coles, G. Cringoli, E. Papadopoulos, J. Cabaret, B. Berrag, M. Varady, J. A. Van Wyk, E. Thomas E, et al. 2009. "The Role of Targeted Selective Treatments in the Development of Refugia-Based Approaches to the Control of Gastrointestinal Nematodes of Small Ruminants." Veterinary Parasitology 164:3–11. https://doi.org/10.1016/j.vetpar.2009.04.015
Kingston, N. 1981. "Protozoan Parasites." In: Diseases and Parasites of White-tailed Deer, edited by W. R. Davidson, F. A. Hayes, V. F. Nettles, and F. E. Kellog. Miscellaneous Publication No. 7 of Tall Timbers Research Station, Tallahassee, FL.
Levine, N. D. 1961. Protozoan Parasites of Domestic Animals and of Man. Burgess Publishing Co., Minneapolis, Minnesota. https://www.cabdirect.org/cabdirect/abstract/19632901174. Accessed July 2020. https://doi.org/10.5962/bhl.title.7000
Lichtenfels, J. R., P. A. Pilitt, and E. P. Hoberg. 1994. "New Morphological Characters for Identifying Individual Specimens of Haemonchus spp. (Nematoda: Trichostrongyloidea) and a Key to Species in Ruminants of North America." The Journal of Parasitology 80:107–119. https://doi.org/10.2307/3283353
Magruder, N. D., C. E. French, L. C. Mcewen, and R. W. Swift. 1957. "Nutritional Requirements of White-Tailed Deer for Growth and Antler Development" 2. Pennsylvania Agric. Exp. Stat. Nutritional requirements of White-tailed deer for growth and antler development 2. https://www.cabdirect.org/cabdirect/abstract/19591401692. Accessed August 2020.
Niilo, L. 1969. "Helminths, Arthropods and Protozoa of Domesticated Animals" Canadian Veterinary Journal 10:223.
Prestwood, A. K., and S. R. Pursglove. 1981. "Gastrointestinal Nematodes." In: Diseases and Parasites of White-tailed Deer, edited by W. R. Davidson, F. A. Hayes, V. F. Nettles, and F. E. Kellog. Miscellaneous Publication No. 7 of Tall Timbers Research Station, Tallahassee, FL. pp. 318–350.
Prestwood, A. K., S. R. Pursglove, and F. A. Hayes. 1976. Parasitism among White-Tailed Deer and Domestic Sheep on Common Range." Journal of Wildlife Diseases 12:380–385. https://doi.org/10.7589/0090-3558-12.3.380
Prestwood, A. K., J. F. Smith, and W. E. Mahan. 1970. "Geographic Distribution of Gongylonema pulchrum, Gongylonema verrucosum, and Paramphistomum liorchis in White-Tailed Deer of the Southeastern United States." The Journal of Parasitology 56:123–127. https://doi.org/10.2307/3277467
Pursglove, S. R., A. K. Prestwood, V. F. Nettles, and F. A. Hayes. 1976. "Intestinal Nematodes of White-Tailed Deer in Southeastern United States." Journal of the American Veterinary Medical Association 169:896–900.
Pursglove, S. R., A. K. Prestwood, T. R. Ridgeway, and F. A. Hayes. 1977. "Fascioloides magna Infection in White-Tailed Deer of Southeastern United States." Journal of the American Veterinary Medical Association 171:936–938.
Santos, M. C., B. F. Silva, and A. F. T. Amarante. 2012. "Environmental Factors Influencing the Transmission of Haemonchus contortus." Veterinary Parasitology 188:277–284. https://doi.org/10.1016/j.vetpar.2012.03.056
Silva, B. F., M. R. V. Amarante, S. M. Kadri, J. R. Carrijo-Mauad, and A. F. T. Amarante. 2008. "Vertical Migration of Haemonchus contortus Third Stage Larvae on Brachiaria decumbens Grass." Veterinary Parasitology 158:85–92. https://doi.org/10.1016/j.vetpar.2008.08.009
Stromberg, B. E., and L. C. Gasbarre. 2006. "Gastrointestinal Nematode Control Programs with an Emphasis on Cattle." Veterinary Clinics of North America: Food Animal Practice 22:543–565. https://doi.org/10.1016/j.cvfa.2006.08.003
Sykes, A. R., D. P. Poppi, and D. C. Elliot. 1988. "Effect of Concurrent Infection with Ostertagia circumcincta and Trichostrongylus colubriformis on the Performance of Growing Lambs Consuming Fresh Herbage." The Journal of Agricultural Science 110:531–541. https://doi.org/10.1017/S0021859600082113
Vegora, H. H. 1960. "The Effect of Forage Height on the Development of Cattle Nematode Larvae." Journal of Parasitology 46:39–40.
Verocai, G. G., U. N. Chaudhry, and M. Lejeune. 2020. "Diagnostic Methods for Detecting Internal Parasites of Livestock." Veterinary Clinics of North America: Food Animal Practice 36:125–143. https://doi.org/10.1016/j.cvfa.2019.12.003
Waller, P. J. 2006. "Sustainable Nematode Parasite Control Strategies for Ruminant Livestock by Grazing Management and Biological Control." Animal Feed Science and Technology 126:277–289. https://doi.org/10.1016/j.anifeedsci.2005.08.007
Whitlock, S. C. 1939. "The Prevalence of Disease and Parasites of White-Tail Deer." in: Transactions of the 4th North American Wildlife Conference. American Wildlife Institute, Washington, D.C., United States of America. pp. 244-249.
Zajac, A. M., and G. A. Conboy. 2012. Veterinary Clinical Parasitology, 8th edition. Wiley-Blackwell. https://www.amazon.com/Veterinary-Clinical-Parasitology-Anne-Zajac/dp/0813820537. Accessed July 2019.