Introduction
This document explores the use of several compost sources in vegetable production fields at Ft. Pierce and Homestead, Florida. Water quality measurements of three nitrogen forms (nitrate, ammonium, and total nitrogen) were used to identify any changes that would indicate potential nutrient losses from the composts compared with a traditional inorganic fertilizer treatment. The target audience for this publication includes Certified Crop Advisors, vegetable production managers, organic farm managers, compost producers, state and federal agency representatives, and those interested in nutrient management for agricultural and environmental purposes.
Vegetable production in southern Florida occurs on soils with low water-holding capacity that require active irrigation management and sufficient nutrient supply to satisfy crop nutrient requirements for a commercially marketable commodity. High soil hydraulic conductivity and shallow groundwater conditions in southern Florida can result in unintended nutrient movement from crop fields to groundwater and surface water. Balancing the production needs of the farm to be profitable and minimizing the environmental losses of nutrients is a challenge. One method that addresses both nutrient management and water control is the use of compost.
Compost can be produced from a variety of organic materials (feedstocks), such as: wastes produced by urban populations, including municipal solid waste; yard trash/trimmings; food wastes from restaurants and grocery stores; wood wastes from construction and/or demolition; wastewater (from water treatment plants); and biosolids (sewage sludge). Agriculture also produces organic wastes that can be composted: poultry, dairy, horse, feedlot, and swine manures; wastes from food processing plants; spoiled feeds; harvest wastes; and mushroom media. These organic materials can be composted through a biological decomposition process aided by proper aeration and moisture conditions and assisted by some mechanical means to convert raw organic materials into relatively stable humus-like material which reduces the feedstock particle size. During decomposition, microorganisms assimilate complex organic substances and release inorganic nutrients. The process itself can take place using in-vessel (variety of forced aeration and mechanical turning techniques), static aerated pile (a blower to supply air to the composting materials without turning or agitating), or windrow (consists on placing the mixture of raw materials in long narrow piles, or windrows, which are agitated on a regular basis) composting methods. These organic materials are composted through normal microbial decomposition aided by proper aeration and moisture conditions and assisted by some mechanical means to reduce feedstock particle size. After composting, mature compost is relatively uniform in its characteristics, contains essential plant nutrients, and offers other advantages for commercial vegetable production, such as potentially improved water holding capacity and improved organic matter levels in the soil over time.
Nutrients within the compost are a function of the original feedstocks, production methods, and maturity. Some of these nutrients are available to the vegetable crop during the growing season. Thus, addition of compost may also reduce the amount of inorganic fertilizer required for a growing season. Not all nutrients within the compost may be plant-available immediately or even during a short-term growing season. The compost may act as a slow-release fertilizer source, releasing additional nutrient amounts as it further decays. Nutrients from the compost are not immediately soluble. Slow release of nutrients from compost exposes fewer nutrients to possible leaching from rainfall, as opposed to the use of pre-plant inorganic fertilizer that exposes most of the nutrients residing in the soil. This positive effect of nutrient retention is both beneficial for the vegetable crop and for reducing possible environmental concerns.
Many growers are aware of these environmental aspects and have taken positive actions through implementation of Best Management Practices (BMPs). While BMPs deal with a much wider range of management strategies than just water and nutrients, all BMPs are a result of the 1972 Clean Water Act that established the concept of Total Maximum Daily Loads (TMDLs). A TMDL is the maximum amount of a pollutant, such as N, that a waterbody can receive and still meet water quality standards. If the TMDL is exceeded, a water body might be impaired, i.e., could not be used for designated purpose(s), such as potable water, swimming, fishing, etc. Implementation of BMPs is a means of partly or fully achieving the TMDL for a waterbody such as a lake or river.
This document focuses on nitrogen (N), which is mostly supplied to vegetable crops via inorganic fertilizers. However, N can also be supplied from organic sources, such as compost, if it is added to the vegetable nutrient management plan. From a water quality standpoint, N is one of the most important nutrients since it can be easily transported off site in its nitrate form, possibly affecting surface and ground waters adversely.
When considering the quality of human drinking water, N is a primary contaminant of concern, and a limit of 10 parts per million (ppm) of N in the nitrate form has been set by the US Environmental Protection Agency. Environmental loss of N should be considered when developing a nutrient management plan for commercial vegetable production, ensuring that both the crop nutrient requirement for N is satisfied and that N does not migrate from the field. It is important to make a distinction between potable groundwater, which is withdrawn from wells that are usually more than 50 times deeper than the shallow groundwater beneath a farm.
Keeping nutrients, especially N, in the field equates to reduced production costs and is smart economically. Compost use can help growers meet production goals and at the same time recycle both nutrients and organic matter that originate from urban communities. This recycling is, therefore, good for Florida, because it reuses residential organic materials that would otherwise end up in a landfill or incinerator.
The practice of composting with subsequent application to vegetable fields results in a more sustainable system for both the urban and farming communities. An additional energy savings is realized by reducing inorganic N fertilizer use, because manufacturing inorganic N fertilizer requires considerable supplies of natural gas.
Best Management Practices
As defined by rule, BMPs must be economical and technologically implementable for the grower, as well as provide a positive environmental impact. In other words, the practice has to be practical, fit with the overall farm management capabilities, and provide the expected environmental effect(s). Use of compost to meet a portion of the crop nutrient requirement is considered a BMP. A list of BMPs designed for vegetable production can be found in the vegetable and row crop BMP manual at the following website: http://www.floridaagwaterpolicy.com/PDF/Bmps/Bmp_VeggieAgroCrops2005.pdf.
Nitrogen Mobility
Nitrogen can change its chemical form in several ways. These changes and resulting N forms are components of the N cycle. For the purpose of this document, the primary forms we considered are explained below.
- Nitrate-N is an oxidized form of N (associated with oxygen), is readily taken up by plants, and is highly mobile in soils, often moving with water in the soil profile. Usually nitrate-N concentration in groundwater is higher than in surface water.
- Ammonium-N is a reduced form of N (associated with hydrogen), is taken up by plants (often in lower amounts than nitrate), is much less mobile than nitrate, and can also be found in surface water and groundwater. Usually ammonium-N moves more slowly in soil compared with nitrate-N.
- Organic N is the fraction of N associated with organic molecules. It is usually not directly plant available, but it can be present in surface and groundwater. Usually organic N moves much more slowly in soil compared with nitrate-N.
- Total N is the sum of all N forms above.
In addition to N form, N mobility is controlled in part by the types of soil in which vegetable production occurs. In Florida, production areas are dominated by sandy soils, such as the sands found in the Ft. Pierce area on the eastern coast of Florida's peninsula. Winter production of vegetables is centered in extreme southern Florida around Homestead, which has calcareous, gravelly soils.
Both sandy and gravelly soils contain large pores, allowing water to move easily vertically and laterally. This soil property also means that nitrate-N can move readily. Since water is the carrier of nitrate-N, controlling its transport away from the field requires an active water management plan. For example, excessive irrigation or a large rainfall can move N below the root zone and into the shallow groundwater, making that N unavailable for the crop and possibly contributing nutrients to adjacent water bodies.
How We Conducted the Tests and What We Measured
While many blends of various feedstocks can be composted together, we elected to use commercially prepared and readily available composts (Table 1) for the tests at both locations. No commercial names are used in this document to avoid recommending one product vs. another. Amendments and fertilizers were roto-tilled to a depth of 4 inches. Most of the amendment was contained in the 10-inch-high beds using a standard, raised-bed polyethylene mulch cultural practice. Seepage irrigation was used at Ft. Pierce (Figure 1), and micro (drip) irrigation was used at Homestead. Irrigation was scheduled based on tensiometer readings to keep optimum soil moisture in the root zone. Rainfall was recorded for both sites to characterize N movement to groundwater.
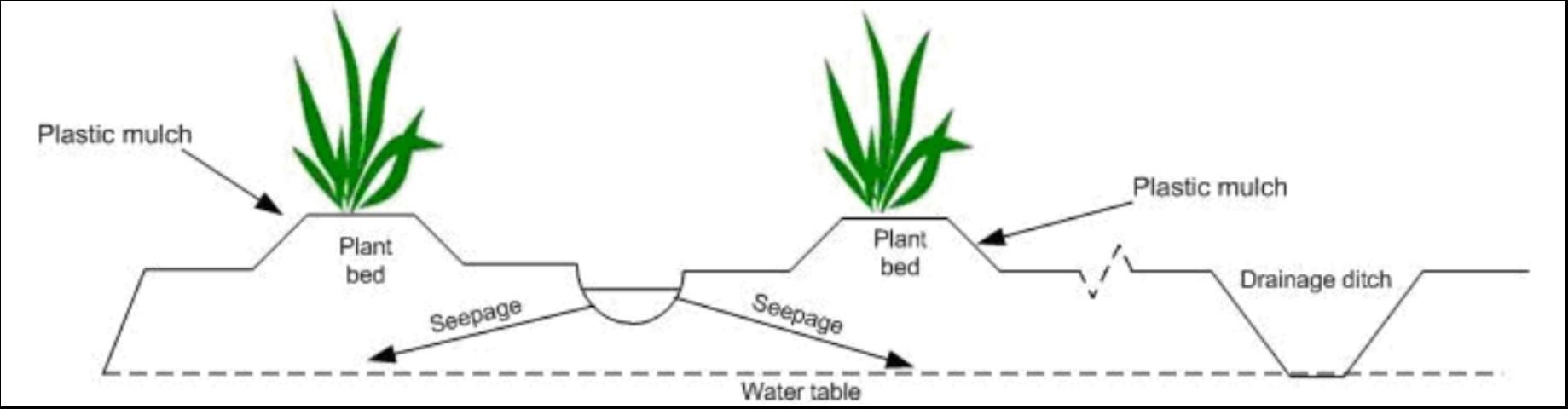
Groundwater Sampling at Ft. Pierce and Homestead
The soil at Ft. Pierce was Oldsmar sand, a typical flatwoods soil with restrictive Bh and Bt horizons (Figure 2). Water percolating through the soil is impeded by the restrictive horizon, creating a perched water table. A small portion of the water reaching the restrictive layer moves vertically through the layer, while the major portion moves laterally toward field drainage ditches. This hydrology necessitated the monitoring of groundwater above and below the restrictive layer. Two types of wells, shallow (above restrictive layer, depth = 3 ft) and deep (below restrictive layer, depth = 11 ft) were installed in each treatment (Figure 2).
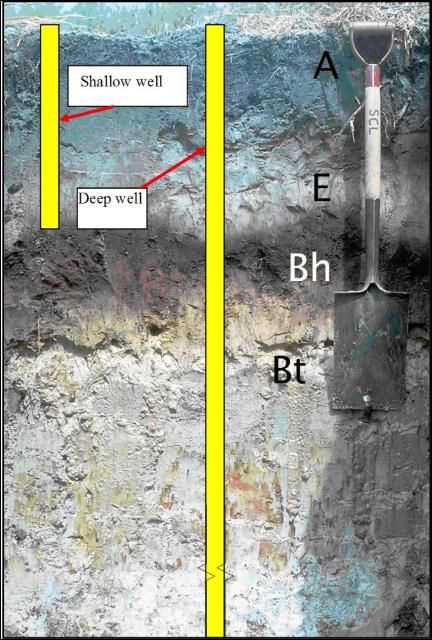
Credit: M. Bauer
The soil profile at Homestead contained no restrictive horizon (Figure 3), so only one well per treatment was used. The soil was Krome, a very gravelly loam, underlain by limestone rock.
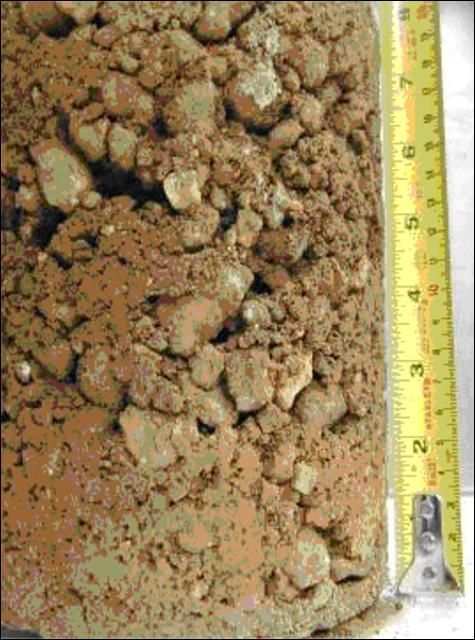
Credit: Y. Li
To evaluate groundwater nutrient inflow and outflow from the study areas, wells were located outside of the areas along the hydraulic gradient found at both sites. A pair of wells was used at Ft. Pierce, and single wells were used at Homestead.
Amendment Rates
The soil amendment rate was calculated to deliver 175 lbs of N per acre (previous UF/IFAS recommendation for tomato), regardless of other nutrients found in the amendment. Soil testing was used to determine the recommended UF/IFAS rates of other nutrients.
The experiments were conducted according to typical grower practices in the two production areas (Table 2). A tomato-squash rotation was used at Ft. Pierce, while a tomato-calabaza rotation was imposed at Homestead. Amendment applications were made twice on the sandy soil and three times on the gravelly soil (Table 2). While additions of organic matter like the composts used in this demonstration project improve soil quality, a measurable increase in soil organic matter is quite slow due to the rapid decay processes given Florida's hot, humid climate. Therefore, any increases in soil organic matter usually occur from repeated additions of organic matter, such as these annual additions.
What We Found
Rainfall
Rainfall amounts at both locations followed typical patterns for peninsular Florida with a distinct dry period during the winter and early spring, followed by a wet season. As expected, Ft. Pierce received 2 inches less rainfall compared with Homestead, which often receives additional moisture from the Gulf of Mexico and Caribbean.
Wells Located Up and Down Slope of the Study Areas
Information from these wells indicated that nutrients were entering the site at Ft. Pierce from adjacent land, but not at Homestead. Concentrations were not a concern at either site. Concentrations were generally lower in water exiting the sites, indicating that the amendments as a group did not have an impact on water quality.
Nitrates
Nitrate-N concentration was less affected by rainfall at the Ft. Pierce site than at the Homestead site. This finding agrees with the relatively slower water movement that occurred in the sandy soil compared with the more rapid water infiltration in the gravelly soil.
At Ft. Pierce, the mean nitrate-N concentration in shallow wells was consistently less than 0.5 mg/L (Figure 4). On only one sampling date did the inorganic fertilizer (Fert) and the biosolid (BS) treatments have higher nitrate-N concentrations than the rest of the treatments (Figure 4). There was no difference in nitrate-N concentrations in deep wells as a result of treatments, which mirrorred shallow well findings and concentrations.
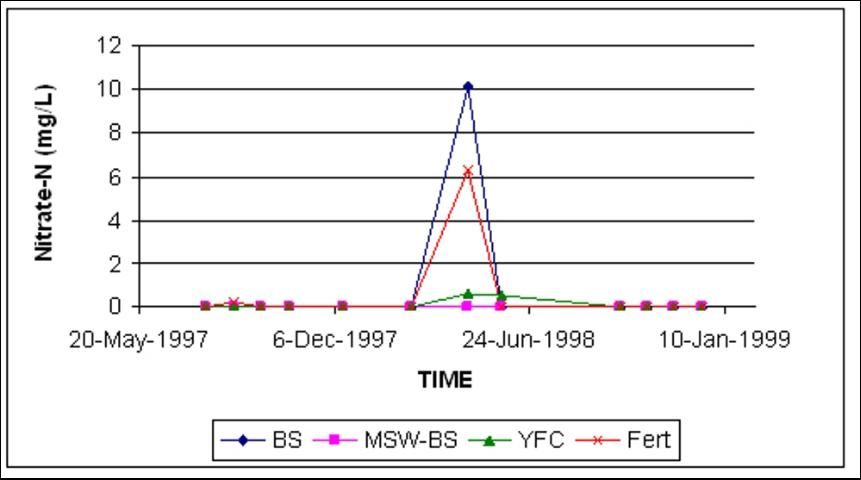
The Homestead site showed more erratic fluctuations in groundwater nitrate-N (Figure 5). This result was expected due to the much higher water movement rate through the gravelly soil. However, concentrations did not exceed the maximum contamination limit of 10 ppm of nitrate-N, except by the MSW-BS treatment at the beginning of the rainy season.
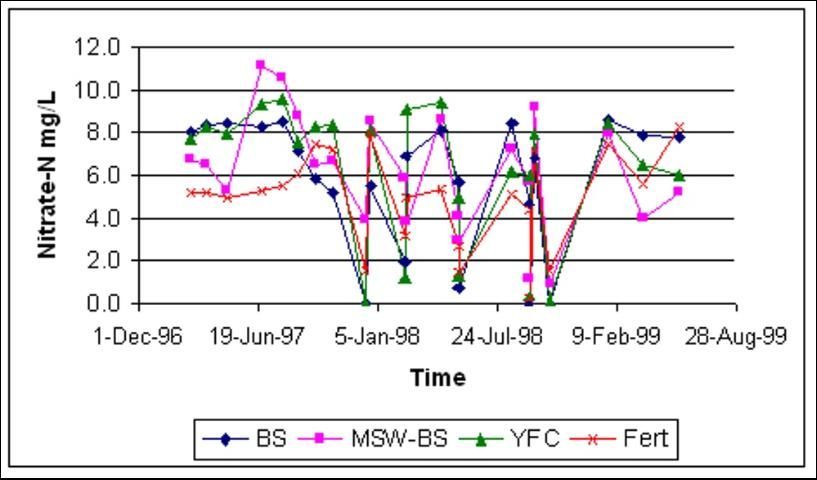
The C:N ratio of these organic amendments varied between 6 and 29 (Table 1). The amendment with the smallest C:N ratio would be expected to contribute more N earlier in the season than amendments with higher ratios. The single, unusually high nitrate-N concentration observed in the FERT and BS treatments at Ft. Pierce (Figure 4) was probably due to the lower C:N ratio of BS.
Ammonium
While there is no set maximum contamination limit for ammonium-N concentration in drinking water, values remained less than 0.5 ppm for all organic amendments. As with nitrate-N, a higher C:N ratio proved to be an indicator of how slowly ammonium-N would be released (larger ratio implies slower release). Both FERT and BS released more ammonium-N soon after application compared with other organic sources.
Ammonium-N, a positively charged ion, can also be held within the soil by charged particles (Cation Exchange Capacity, or CEC). Both soils have relatively low cation exchange capacities, and when coupled with the coarse textures of these soils, little ammonium-N would be retained by this mechanism. As is the case with nitrates, controlling water movement will also contribute greatly to slowing the loss of ammonium from the root zone. No difference in ammonium-N due to amendments was detected in the deep wells at Ft. Pierce, indicating that this N form was either utilized by the plant, converted to nitrate (nitrogen cycle), or moved laterally above the restrictive horizons. The inorganic N source (FERT) had one slightly elevated concentration early in the season, but did not exceed 1.5 ppm ammonium-N.
Total Nitrogen
Total N concentrations in shallow groundwater were similar at both locations, regardless of amendment treatment (Figures 6 and 7). Total N at both sites was dominated by fluctuations of nitrate-N.
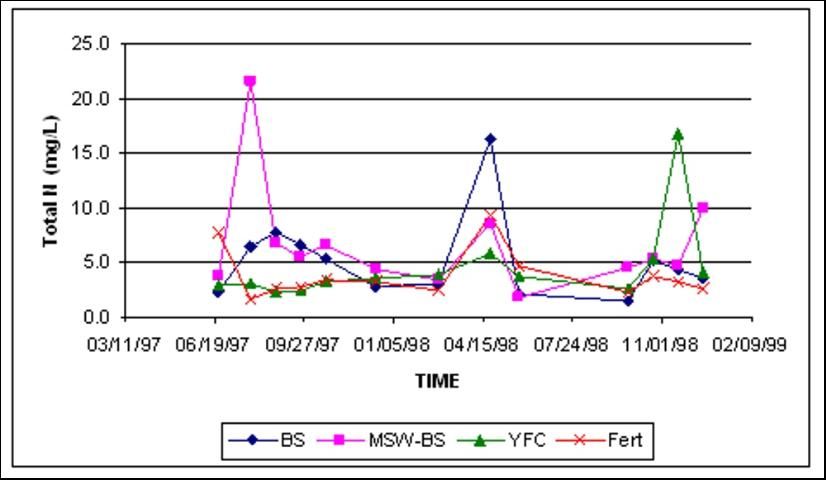
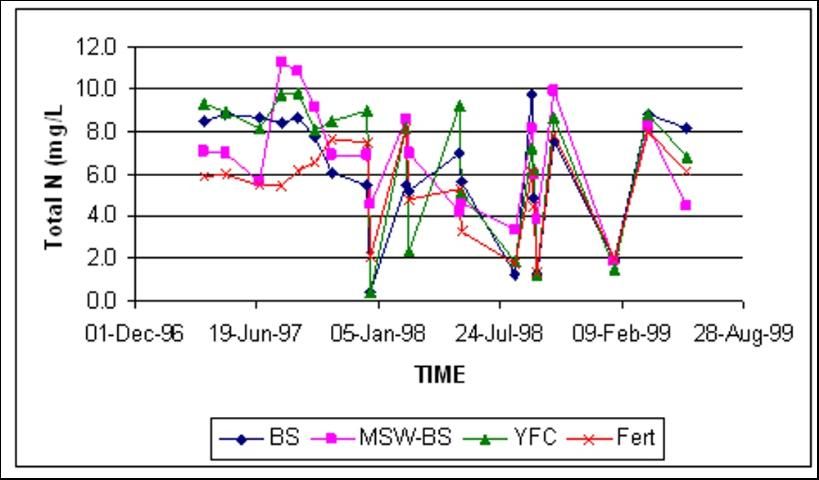
Crop Yields
Vegetable yield data from the sandy soil site showed similar yields of both organic and inorganic nitrogen sources, indicating that either source was effective in providing available N to commercially grown vegetables. Tomato yield data at Ft. Pierce were not collected for the second year of the experiment. The yield data suggested that compost not only resulted in similar or higher yields than the inorganic fertilizer but also did not increase the N concentration in the groundwater compared with the inorganic fertilizer. Yield data from the Homestead site was not available.
Conclusions
- Composts supplied available N at sufficient amounts and rates to satisfy the crop N requirement of tomato and squash at the sandy soil site. While these field trials addressed only two crops, similar yield performance is possible for other commercially produced vegetables.
- Nitrogen was supplied through compost addition without increasing groundwater N concentrations compared with inorganic fertilizers.
- Composts with high C:N ratios supplied N with somewhat decreased N leaching potential compared with composts with a low C:N ratio or inorganic fertilizer sources.
- Using N from composts also recycles other nutrients and provides for an environment-friendly means of waste disposal. At the same time, soil quality can be improved and/or maintained.
How Do We Use This Information
We conducted this study in two of Florida's important vegetable growing regions. From a groundwater N standpoint, we found that use of organic amendments did not adversely impact water quality compared to traditionally used inorganic N fertilizer. From a fertility standpoint, organic amendments at the sandy soil site provided similar crop yields to those obtained from inorganic fertilizer, demonstrating that they are a viable source of nutrition. In certain areas of Florida where P is the main water quality concern, the rate of compost application should be based on the plant requirement of P and not N. The fertilizer P requirement for vegetables can be obtained from UF/IFAS fertilizer recommendations. In addition to satisfying the nutritional requirement of these vegetables, use of organic amendments also has other potential benefits, such as increasing the water-holding capacity of Florida's sandy soil and recycling urban by-products in an environmentally safe manner. Use of organic compost should be expanded for vegetable production in Florida where it is economically feasible.
For More Information
Farming for Clean Water in South Carolina: A Handbook of Conservation Practices—http://www.worldcat.org/title/farming-for-clean-water-in-south-carolina-a-handbook-of-conservation-practices/oclc/37535785 (June 2016)
Cage Layer Manure: An Important Resource for Land Use—http://ufdc.ufl.edu/IR00006164/00001 (June 2016)
Poultry Manure as a Fertilizer—https://edis.ifas.ufl.edu/AA205 (June 2016)
Soil Organic Matter, Green Manures and Cover Crops for Nematode Management—https://edis.ifas.ufl.edu/VH037 (June 2016)
Utilization of Organic Wastes in Florida Agriculture—http://ufdc.ufl.edu/IR00003232/00001 (June 2016)
Soil and Fertilizer Management for Vegetable Production in Florida—https://edis.ifas.ufl.edu/CV101 (June 2016)
UF/IFAS North Florida Research Center—Suwannee Valley—http://nfrec.ifas.ufl.edu/ (June 2016)
Sampling Guide from North Florida Research and Education Center Animal Waste Testing Laboratory—http://soilslab.ifas.ufl.edu/LWTL%20Home.asp (June 2016)
References
Chen, M., L.Q. Ma, W.G. Harris, and A.G. Hornsby. 1999. Background Concentrations of Trace Metals in Florida Surface Soils: Taxonomic and Geographic Distributions of Total-Total and Total-Recoverable Concentrations of Selected Trace Metals. Report #99-7. Florida Center for Solid and Hazardous Waste Management, Gainesville, FL.
Dyer, J.M., and A.S. Razvi. 1987. Assessing risk of solid waste compost. Biocycle 28(3):31–36.
Fernald E.A., and E.D. Purdum, (Eds.). 1998. Water Resources Atlas of Florida. Institute of Science and Public Affairs, Florida State Univ., Tallahassee, FL.
Florida Agricultural Statistics Service. 2003. Vegetables Spring Acreage. USDA-NASS, Washington, DC. http://www.nass.usda.gov/Statistics_by_State/Florida/Publications/Vegetables/spring/vegspr03.pdf (June 2016).
Florida Department of Environmental Protection. 2000. Criteria for the Production and Use of Compost Made of Solid Waste. Chapter 62–709. FDEP, Tallahassee, FL.
Gerke, H.H., M. Arning, and H. Stöpler-Zimmer. 1999. Modeling long-term compost application effects on nitrate leaching. Plant Soil 213:75–92.
Gomez, K.A., and A.A. Gomez. 1984. Statistical Procedures for Agricultural Research. 2nd ed. New York: John Wiley and Sons, Inc.
He, Z.L., A.K. Alva, P. Yan, Y.C. Li, D.V. Calvert, P.J. Stoffella, and D.J. Banks. 2000. Nitrogen mineralization and transformation from composts and biosolids during field incubation in a sandy soil. Soil Sci. 165:161–169.
He, Z.L., D.V. Calvert, A.K. Alva, Y.C. Li, and D.J. Banks. 2002. Clinoptilolite zeolite and cellulose amendments to reduce ammonia volatilization in calcareous sandy soil. Plant Soil 247:253–260.
Henry, C., D. Sullivan, R. Rynk, K. Dorsey, and C. Cogger. 1999. Managing N from Biosolids. Publication # 99-508. NW. Biosolids Mgment. Assoc. and WA. State Dep. of Ecology, Olympia, WA.
Hochmuth, G.J., and E.A. Hanlon. 2000. IFAS Standardized Fertilization Recommendations for Vegetable Crops. CIR 1152. Gainesville: University of Florida Institute of Food and Agricultural Sciences. http://ufdc.ufl.edu/IR00004663/00001 (June 2016).
Hollander, M., and D.A. Wolfe. 1999. Nonparametric Statistical Methods. New York: John Wiley and Sons, Inc.
Insam, H., and P. Merschak. 1997. Nitrogen leaching from forest soil cores after amending organic recycling products and fertilizers. Waste Management & Res. 15:277-292.
Li, Y.C. 1998. What are mineralization rates of composts in south Florida? Vegetarian 98(8):1.
Li, Y.C., P.J. Stoffella, A.K. Alva, D.V. Calvert, and D.A. Graetz. 1997. Leaching of nitrate, ammonium, and phosphate from compost amended soil columns. Compost Sci. & Utilization 5(2): 63–67.
McCutcheon, S.C., J.L. Martin, and T.O. Barnwell, Jr. 1993. Water quality. In D.R. Maidment (Ed.), Handbook of Hydrology. New York: McGraw-Hill, Inc.
McDowell, W.H., W.S. Currie, J.D. Aber, and Y. Yano. 1998. Effects of chronic nitrogen amendment on production of dissolved organic carbon and nitrogen in forest soils. Water Air Soil Pollut. 105:175–182.
McDowell, W.H., and T. Wood. 1984. Podzolization: Soil processes control dissolved organic carbon concentrations in stream water. Soil Sci. 137:23–32.
Nikolaidis, N.P., P. Chheda, J.A. Lackovic, K. Guillard, B. Simpson, and T. Pedersen. 1999. Nitrogen mobility in biosolid-amended glaciated soil. Water Environ. Res. 71(3):368–376.
Obreza, T.A., A. K. Alva, and D.V. Calvert. 1993. Citrus Fertilizer Management on Calcareous Soils. CIR1127. Gainesville: University of Florida Institute of Food and Agricultural Sciences. https://edis.ifas.ufl.edu/CH086 (June 2016).
Ozores-Hampton, M., T.A. Obreza, and G. Hochmuth. 1998. Using composted wastes on Florida vegetable crops. HortTechnology 8(2): 130–137.
Shukla, S., S. Mostaghimi, and J.A. Burger. 2000. Laboratory measurements and modeling N mineralization potential of agricultural and forest soils in Virginia coastal plain. Trans. ASAE 43(2): 315–325.
Sims, J.T. 1995. Organic wastes as alternative nitrogen sources. In P.E. Bacon (Ed.), Nitrogen Fertilization in the Environment. New York: Marcel Dekker, Inc.
Smith, W.H. 1990. Organic waste management in Florida. Biocycle 31(4):52–55.
Stoffella P.J., and B.A. Kahn (Eds.) 1993. Composting Utilization in Horticultural Cropping Systems. Boca Raton, FL: Lewis Publishers.
USDA. 1980. Soil Survey of St. Lucie County Area, Florida. USDA-SCS, Washington DC.
USDA. 1996. Soil Survey of Dade County Area, Florida. USDA-NRCS, Washington DC.
USEPA. 1993. Methods for the Determination of Inorganic Substances in Environmental Samples. EPA/600/R-93/100. Environmental Monitoring Systems Laboratory, Office of Research and Development, U.S. Environmental Protection Agency, Cincinnati, OH.
USEPA. 2002. Current Drinking Water Standards. EPA 816-F-02-013. Office of Water, U.S. Environmental Protection Agency, Washington, DC.