Introduction
This article introduces agricultural and horticultural producers to the role and functions of the newest identified essential plant nutrient, nickel (Ni).
The criteria for essentiality of nutrient elements for plant growth and development were established by Arnon and Scott (1939) and Meyer and Anderson (1939; please also see Bennett 1993). An essential nutrient is defined as follows:
- A given plant must be unable to complete its life cycle in the absence of the nutrient (life cycle = vegetative state, flower, seed production).
- The function of the element must not be replaceable by another element.
- The element must be directly involved in plant metabolism or must be a component of an essential plant constituent (e.g., nitrogen [N] is a constituent of proteins and chlorophyll).
Based on these criteria, Brown and colleagues (1987) determined in the late 1980s that nickel is a nutrient essential for both monocotyledons and dicotyledons. However, American Association of Plant Food Control officials did not recognize Ni as essential until 2004. Nickel is recognized as the seventeenth element essential for plant growth and development (Liu 2001). Plants' Ni requirement is the lowest of all essential elements at < 0.5 mg per kg of dry weight, making it an essential plant micronutrient. Nickel is required for plants at such low concentrations that analytical technologies were not available until the mid-1970s.
Nickel is unique among plant nutrients because its functions in plant growth and development were described in detail before nickel was added to the list of essential elements. Nickel is a key component of selected enzymes (described below) involved in N metabolism and biological N fixation. Plants suffering from Ni deficiency show necrosis initiating from the tip of the leaf (Figures 1–4). This symptom can be reversed or corrected by applying a dilute Ni solution (Figure 5). In 1945, W. A. Roach and C. Barclay obtained the first evidence that Ni significantly increased yields of potato (Roach and Barclay 1946), bean, and wheat grown in the acidic sands found in Romney Marshes of England, which have very low concentrations of manganese (Mn), Ni, and zinc (Zn). Similar soils are prevalent in the southeastern United States, including Florida.
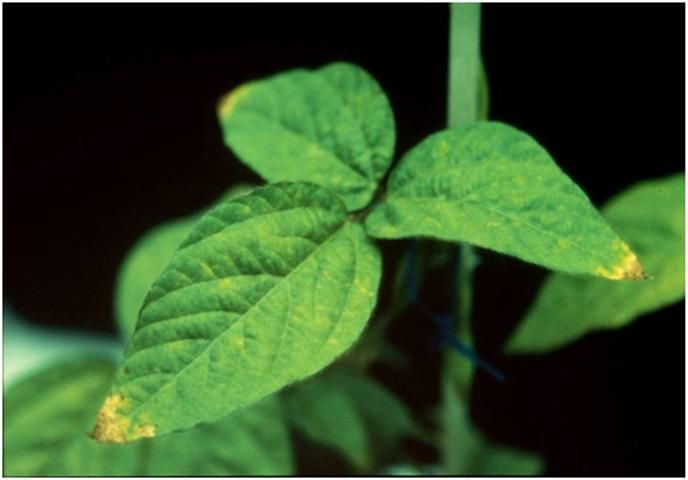
Credit: Patrick Brown, University of California, Davis
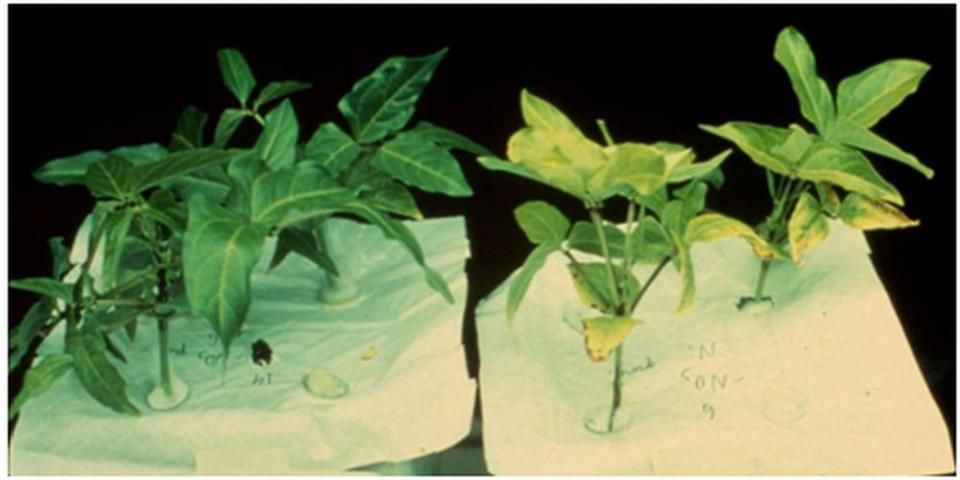
Credit: Patrick Brown, University of California, Davis
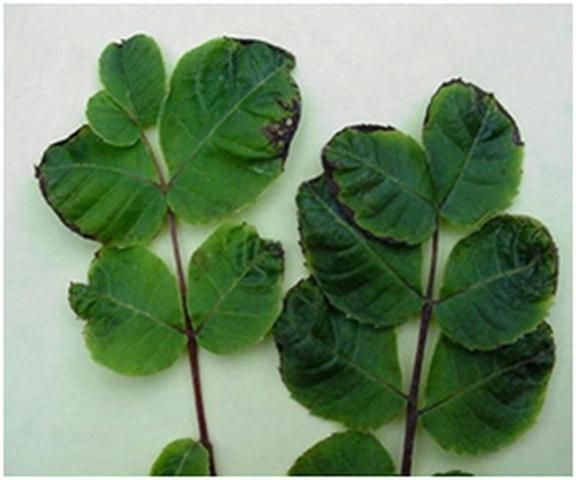
Credit: M. L. Wells, University of Georgia, 2010
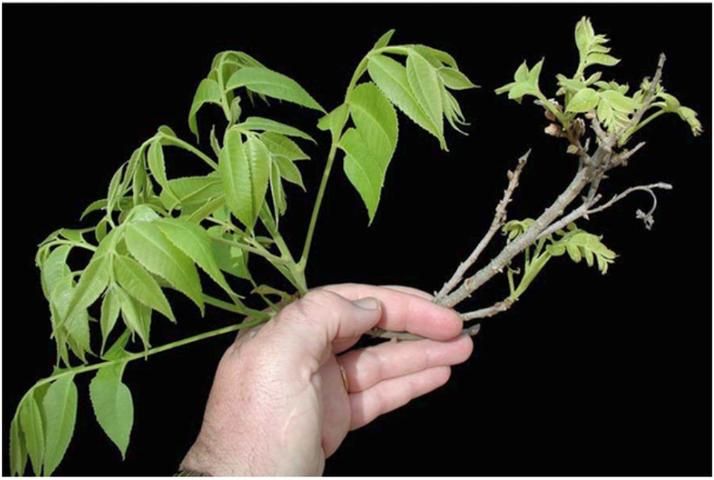
Credit: Bruce Wood, USDA-ARS, Byron, GA
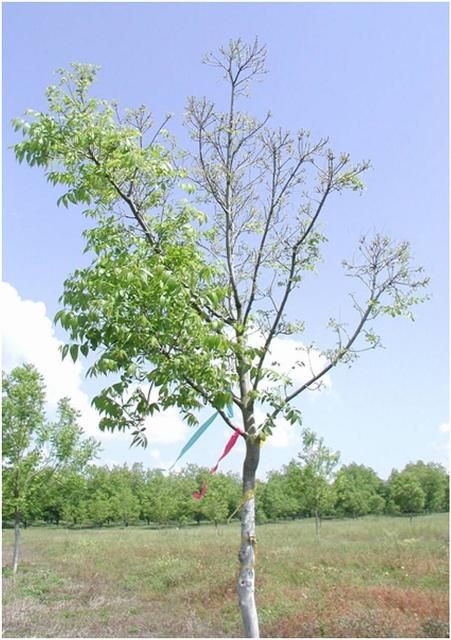
Credit: Bruce Wood, USDA-ARS, Byron, GA
Functions of Nickel in Plants and Symptoms of Nickel Deficiency
Nickel is a functional constituent of eight enzymes, including Glx I (EC 4.4.1.5)l ARD (EC 1.13.11.54), Ni-SOD (EC 1.15.1.1.) Hydrogenase (EC 1.12.98.2), MRC (EC 2.8.4.1), CODH (EC 1.2.99.2), ACS (EC 2.3.1.169), and urease (EC 3.5.1.5) (i.e., urea amidohydrolase), that is extremely important to N metabolism in plants (Ragsdale 2009). As a Ni-metalloenzyme, urease assists in the hydrolysis of urea. Nickel works as a cofactor to enable urease to catalyze the conversion of urea into the ammonium ion, which plants can use as a source of N. Without the presence of Ni, urea conversion is impossible. Nickel is accumulated in plant organs or tissues, such as leaves. In 1983, D. L. Eskew and colleagues reported that legume plants grown in nutrient solutions containing urea as the N source developed necrotic lesions on the leaflet tips (Figure 2). The crop plants accumulated 2.5% urea dry weight in the leaves. The leaf tip necrosis symptom of Ni deficiency is even more frequent and extensive when plants are highly dependent on biological N fixation for their N. This concentrated urea kills leaf cells, resulting in the development of necrotic lesions on the legume leaf tips (Figure 2). Nickel-deficient cowpea develops similar symptoms of leaf chlorosis and leaf tip necrosis (Figure 3). These symptoms suggest that urea is formed during normal N metabolism, regardless of the original N source. Application of Ni increases leaf urease activity and prevents urea accumulation.
Nickel deficiency also results in delayed nodulation and reduced efficiency of N fixation. This finding suggests leguminous plants might have a unique requirement for Ni. Therefore, for leguminous crops such as green bean and cowpea, Ni fertilization might be needed, particularly for those soils with high Zn or copper (Cu) concentrations, or with pH > 6.7 (Brown 2006).
In Ni-deficient pecans, a key morphological symptom of Ni deficiency is the development of "mouse-ear" leaves (Figures 3 and 4). On branches of Ni-deficient pecans, leaf expansion is both delayed and decreased; bud-break is greatly reduced; and leaves present bronzing, chlorosis, resetting, and tip necrosis (Figures 1–4). It seems likely that these symptoms are linked to oxalic and lactic acid toxicity, which accumulates because Ni deficiency disrupts pecan's carbon metabolism. Bai and colleagues (2006) demonstrated that Ni-deficient pecan plants accumulated twice as much organic acid as Ni-sufficient plants. They found that lactic acid increased 3.2-fold and oxalic acid increased 2.4-fold, compared to levels in Ni-sufficient pecans. These accumulations disrupted the conversion of certain organic acids to other metabolites necessary for normal growth and development. This disruption of carbon metabolism might also be associated with diminished plant resistance to certain diseases (Graham et al. 1985).
Additionally, a positive effect of Ni application on disease tolerance has been clearly documented. It is thought that nickel may either exert a direct phyto sanitary effect on pathogens themselves, or that nickel may stimulate plant disease-resistance mechanisms. Although Ni's mode of action in plant protection is unclear, it was shown that direct application of Ni to the roots of cowpea, which contained only 0.03 mg kg-1 Ni dry weight, effectively reduced leaf-fungal infection by 50% (Brown 2006).
Bioavailable Form of Nickel
Human use of Ni has been traced as far back as 3500 BCE. However, it was first isolated and classified as a chemical element from Kupfernickel by the Swedish mineralogist and chemist, A. F. Cronstedt, in 1751. Nickel has five valences: 0, +1, +2, +3, and +4. Among these, only Ni2+ is considered an available form for plants.
Nickel Concentration in Soils
Nickel comprises approximately 3% of the earth's crust composition and is the twenty-fourth most abundant element (Hemantaranjan 2014). Total Ni concentration commonly ranges from 5 to 500 mg kg-1, with an average of 50 mg kg-1 in soils. However, Ni concentrations in dried biosolids (also referred to as treated sewage sludge) or soil near metal refineries are in a range from 24,000 to 53,000 mg kg-1. Soils for crop production contain 3–1,000 mg kg-1. Because Ni2+ is the available form of Ni for plants, total Ni concentration is not a useful measure for Ni bioavailability. Positive 2-valence Ni ion (Ni2+) readily oxidizes and becomes unavailable. Thus, plants grown in high-pH soils are vulnerable to Ni deficiency. Additionally, excessive use of Zn and Cu may induce Ni deficiency in soil because these three elements share a common uptake system. Over liming, which raises pH excessively, also causes soil deficient in plant-available Ni. Thus, in soils that have to be high-pH, either naturally or artificially, Ni fertilization may be needed to ensure good crop quality and yield.
Soil Testing for Nickel
Several soil test methods have been tried for predicting the crop's need for Ni fertilizer. Many extractants have been employed to determine the soil's concentration of exchangeable Ni, including diethyltriaminepentaacetic acid (DTPA, C14H23N3O10), BaCl2, Sr(NO3)2, and ammonium acetate. The DTPA method is the most used and effective for a variety of soils. However, standards for Ni-deficient soils have not been established. Nickel deficiency can occur because of excessive use of competing ions, such as Zn and Cu, unfavorable conditions, such as high pH, or use of intensively oxidized soils for crop production. In Florida, the background level of Ni in agricultural surface soils statewide has been reported as 8 mg/kg, which is much lower than that of agricultural surface soils (17 mg/kg) and soil in general (50 mg/kg) nationwide (Ma et al. 1997). However, there has been no survey for Ni nutrition in crop production. No one knows whether there is a Ni deficiency problem in plant industries because nickel is a new micronutrient. However, pecan plants grown in Georgia have recently shown typical symptoms of Ni deficiency (Wells and Harrison 2010). The information and photos included in this publication may help the Florida plant industries identify and correct this nutrient disorder.
Nickel Uptake and Transport
Plants have two transport systems (Brown 2006): low affinity and high affinity. With the low-affinity transport system, plants can absorb Ni2+ ions at the low concentration of 4.4 parts per billion (ppb), which is approximately 0.6 ounces Ni per million gallons of water. With the high-affinity transport system, plants can take up 1.8 parts per million (ppm) of Ni2+ ions, which is 237.7 ounces per million gallons of water. Nickel is readily re-translocated within the plant, probably as a complex with organic acids, such as citrate, at pH < 5, or with an amino acid, such as histidine, at pH > 6.5. When plants experience Ni deficiency, the symptoms usually show up first on mature leaves (Figures 2 and 3). Also, due to the transportability of Ni2+ ions, up to 70% of Ni in shoots can be transported to seeds (Brown 2006).
Plant Tissue Nickel Content
The Ni concentration in plant leaves ranges from 0.05 to 5 mg kg-1, which is equal to 0.05–5 ppm on a dry weight basis. The critical Ni concentration in plant tissues required for normal shoot growth of urea-fed tomato and zucchini is about 1 ppm. Nickel concentrations = 10 ppm are generally considered to be toxic to sensitive species or cultivars. Table 1 contains a Ni-sufficiency range for several vegetable and fruit crops (Brown 2006).
Nickel Fertilization
As a micronutrient, also called a nano-nutrient, Ni is required by plants at low concentration (Theuer 2009). Most annual plants have a requirement for Ni on the order of 0.5 lb per acre, compared with nitrogen (N) at 80–200 lb per acre. Application of Ni fertilizers (Ni2+) might be needed in the following growth conditions: (1) urea is the primary N source used for the crop production systems; (2) high applications of other metals, including Zn, Cu, Mn, iron (Fe), calcium (Ca), or magnesium (Mg), have been made for many years; or (3) leguminous crops are being grown in soils poor in mineral content or with a pH > 6.7. Soluble salts like nickel sulfate (NiSO4), which contains the Ni2+ ion, are suitable fertilizers to prevent or correct plant Ni deficiency. Applying a foliar spray at a concentration of 0.03–0.06 ppm Ni is sufficient. An application of 0.5 lbs Ni per acre is all that is required (NIPAN LLC 2011). Also, municipal biosolids fertilizer is a good source of Ni. Table 2 summarizes Ni fertilizer sources.
Summary
- Nickel is the most recently discovered micronutrient, also known as a nanonutrient; plants require it in small amounts.
- Leguminous crops like bean and cowpea require more Ni than other crops because nickel plays an important role in nodulation and N fixation.
- If Ni deficiency occurs, it will likely be associated with soils having pH > 6.7 or soils that have received excessive applications of Zn, Cu, Mn, Fe, Ca, or Mg.
- Nickel deficiency appears as leaflet-tip necrosis, or "mouse-ear" leaves.
- Nickel is a challenging plant nutrient with which to work because it readily oxidizes to unavailable forms in the soil.
- The easiest and most effective strategy to correct acute Ni deficiency is foliar spraying with a dilute solution of NiSO4 or other water-soluble Ni fertilizer.
- Municipal biosolids can be effectively used as a Ni fertilizer.
References
Arnon, D. I., and P. R. Scott. 1939. "The Essentiality of Certain Elements in Minute Quantity for Plants with Special Reference to Copper." Plant Physiology 14:371–375.
Bai, C., C. C. Reilly, and B. W. Wood. 2006. "Nickel Deficiency Disrupts Metabolism of Ureides, Amino Acids, and Organic Acids of Young Pecan Foliage." Plant Physiology 140:433–443.
Bennett, W. F. "Plant Nutrient Utilization and Diagnostic Plant Symptoms." In Nutrient Deficiencies and Toxicities in Crop Plants, edited by W. F. Bennett, 1–7. St. Paul, MN: APS Press.
Brown, P. H. 2006. "Nickel." In Handbook of Plant Nutrition, edited by A. V. Barker and D. J. Pilbeam, 395–410. Boca Raton, FL: CRC Press Taylor & Francis Group.
Brown, P. H., R. M. Welch, and E. E. Cary. 1987. "Nickel: A Micronutrient Essential for Higher Plants." Plant Physiology 85:801–803.
Eskew, D. L., R. M. Welch, and E. E. Cary. 1983. "Nickel: An Essential Micronutrient for Legumes and Possibly All Higher Plants." Science 222:622–623.
Eskew, D. L., R. M. Welch, and W. A. Norvell. 1984. "Nickel in Higher Plants: Further Evidence for an Essential Role." Plant Physiology 76:691–693.
Graham, R. D., R. M. Welch, and C. D. Walker. 1985. "A Role of Nickel in the Resistance of Plants to Rust." Proc. 3rd Australian Agron. Conference. Hobart Tasmania, Australia.
Hemantaranjan, A. 2014. Physiology of Nutrition and Environmental Stresses on Crop Productivity. New Delhi: Scientific Publishers. 595.
Liu, G. D. 2001. "A New Essential Mineral Element – Nickel." Plant Nutrition and Fertilizer Science 7(1):101–103.
Ma, L. Q., F. Tan, and W. G. Harris. 1997. "Concentrations and Distributions of 11 Elements in Florida Soils." J. Environ. Qual. 26:769–775
Macnicol, R. D., and P. H. T. Beckett. 1985. "Critical Tissue Concentrations of Potentially Toxic Elements." Plant Soil 85:107–129.
Meyer, B. S., and D. B. Anderson. 1939. Plant Physiology. New York: D. Van Nostrand.
NIPAN LLC. 2011. "Nickel Plus® 5-0-0." http://nickelplus.biz/assets/pdf/Label%20-%20Nickel%20Plus.pdf (accessed on February 6, 2024).
Ragsdale, S. 2009. "Nickel-Based Enzyme Systems." The Journal of Biological Chemistry, 284, 18571–18575.
Roach, W. A., and C. Barclay. 1946. "Nickel and Multiple Trace Deficiencies in Agricultural Crops." Nature 157:696.
Theuer, R. 2009. Petition for Inclusion on the National List of Allowed Substances: Nickel. Micronutrient at §205.601 U)(6)(ii).
Walker, C. D., R. D. Graham, J. T. Madison, E. E. Cary, and R. M. Welch. 1985. "Effects of Nickel Deficiency on Some Nitrogen Metabolites in Cowpeas, Vigna unguiculata." Plant Physiology 79:474–479.
Wells, M. L., and K. A. Harrison. 2010. "Cultural Management of Commercial Pecan Orchards" B 1304. Athens: University of Georgia College of Agricultural & Environmental Sciences. Accessed April 20, 2011.
Wood, B. W., C. C. Reilly, and A. P. Nyczepir. 2004. "Mouse-ear of Pecan: A Nickel Deficiency." HortScience 39 (6): 1238–1242.