Agricultural Soils of Florida
Soils around the world are categorized into twelve Orders according to the USDA taxonomic classification. Seven of these twelve soil Orders can be found in Florida. They include (in order of estimated areal prevalence) Spodosols, Entisols, Ultisols, Alfisols, Histosols, Mollisols, and Inceptisols. The formation of soils involves a complex process of weathering and is a dynamic and combined function of parent material, climate, topography, biology, and time (Jenny 1941). Land disturbance is a major outcome of urbanization and agriculture, including filling, spreading, mixing, and other activities that significantly influence the natural formation of soils. Therefore, it is important to remember that the accuracy of soil maps can be compromised on a local scale because of anthropogenic activities at the time of their creation.
The soils of the southeastern United States mostly developed under forests with abundant rainfall. The abundant rainfall leached base cations, such as potassium (K), calcium (Ca), magnesium (Mg), and sodium (Na), resulting in soils with an acidic nature. The parent materials of Florida's soil are predominantly sandy marine sediments to the north of Lake Okeechobee and organic and carbonatic (mainly calcium carbonate) materials to the south. The subtropical climate combined with year-round rain resulted in high soil temperature and moisture conditions that promoted microbial growth and rapid organic matter mineralization. Thus, soil organic matter levels tend to be lower compared to that of the northern regions of the United States (Mylavarapu et. al. 2014). The varying properties of agricultural soils in Florida should be considered when assessing the required application rates of nutrients and other amendments because a loss of soil can lead to profit loss as well as a potential ecological liability (Harris et al. 2010).
This publication describes the various soil Orders in Florida, how they were developed, their characteristics, coverage in the state, and uses. This information is important for educating land owners, decision-makers, and educators about soils in Florida, leading to better soil management for agricultural and environmental sustainability.
Spodosols
Spodosols are most extensively-occuring soils in Florida, covering approximately 8.4 million acres in the state (Figure 1). Spodosols most often develop in coarse-textured parent materials (sands and loamy sands) under coniferous vegetation in humid regions of the world.These soils are sandy and acidic (acidic parent material) and have an accumulation of organic matter, aluminum (Al), and iron (Fe) (spodic horizon) in the subsoil, usually with a greater abundance of Al than Fe. The majority of the spodosols in Florida are poorly- or very-poorly-drained and are widespread on flatwoods landscapes. Spodosols include the Myakka (the Florida State Soil), Leon, Immokalee, Pomona, and Smyrna soils. These soils are characterized with low but intense leaching, augmented by organic-acid-induced mobilization of Al, results in vertical translocation of minerals and organically-complexed Al. These translocated components accumulate at some depth during the formation of a black to reddish-brown spodic (Bh) horizon. The Bh is far more reactive than overlying horizons, but access to the Bh by plant roots is often interupted by seasonally-high water tables. Spodosols are easily identified by their white or light gray E horizon, an immediate subsurface layer where intense leaching of minerals, organic, iron, and aluminum compounds has exposed clean quartz sand grain surfaces, imparting a very light color to the horizon.
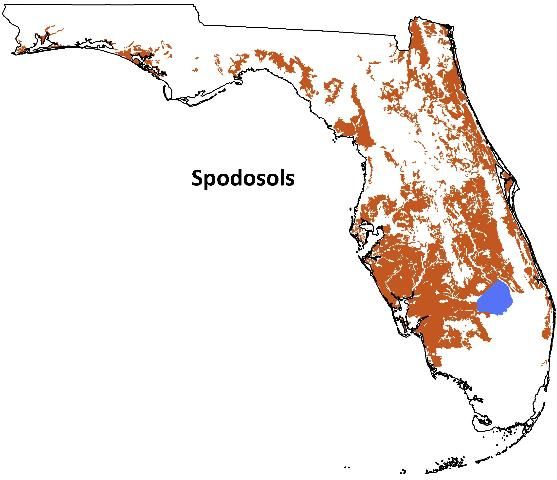
Credit: Greg Means, UF/IFAS
Heavy application of fertilizers and lime has enabled cultivation of grains, potatoes, tomatoes, peppers, berries, and silage corn (maize) in spodosols. However, high water tables and sandy textures mean that the application of soil amendments in Spodosols must be thoughtfully executed to provide sufficient nutrients while minimizing the loss of nutrients. In Florida, most Spodosols belong within the suborder Aquods, which are poorly drained Spodosols with a water table at or near the surface for much of the year. Therefore, without drainage management, nutrient retention in Spodosols can prove difficult. Additionally, the E horizon that is available for most plant rooting depths has a minimal area of reactive surface, thereby providing essentially no phosphorus (P) retention capacity (Harris et al. 1996) and reducing P availability to plants while increasing P loss to the environment. Water table depth in relation to E-horizon thickness affects the availability of applied P to crops and the potential for lateral transport of P via subsurface flow (Harris et al. 2010). Artificial drainage to increase water movement from the site and lower the water table to allow infiltration to reach the B horizon can reduce P mobility (Harris et al. 2010). The poorly drained conditions of Spodosols can also lead to N losses via denitrification; this pathway for N loss should be considered in determining optimal N fertilization rates (Harris et al. 2010).
Entisols
Entisols are soils that, within 2 meters depth, lack most diagnostic horizons defined under USDA soil taxonomy. In most areas of the world, they are associated with young surfaces and minimal soil development. However, a high proportion of Florida Entisols occur on older geomorphic surfaces in association with Ultisols, have sandy textures, and are indeed intensely weathered. These sandy Entisols range from excessively to poorly-drained. Some commonly-occuring, sandy Entisols include the Astatula, Candler, Kershaw, Ortega, Chipley, Basinger, and St. Lucie soils. Non-sandy but coarse and silt-textured Entisols also occur in Florida. These latter Entisols occur on the younger, poorly-drained geomorphic surfaces of the south Florida peninsula, where they are underlain at shallow depth by limestone and form in carbonate mud. Perrine and Pennsuco are two common soil series that form in the carbonate-rich parent materials of the region. Entisols cover approximately 7.5 million acres; they occur in every region of the state (Figure 2).
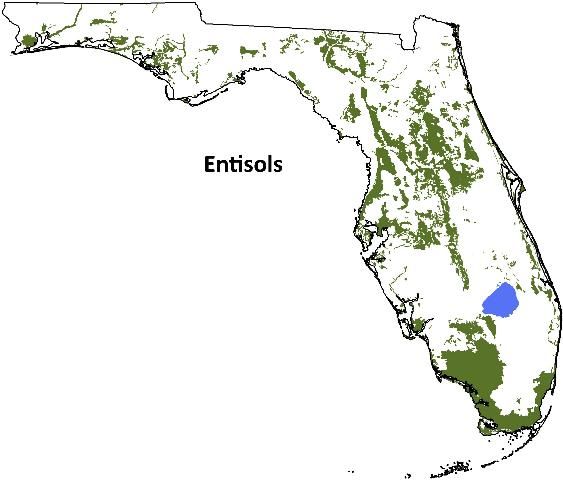
Credit: Greg Means, UF/IFAS
While citrus is predominantly produced on sandy Entisols, a variety of other agricultural crops, including vegetables and fruit crops, are cultivated. Entisols warm up fast in the spring for early vegetable crops, are easy to till, and can be very productive if irrigation and fertilizers are provided.
The sandy Entisols of Florida (Psamments) are acidic, typically composed of more than 98% sand, and have a low CEC and base saturation. They tend to have sand grains that are coated with Fe or Al oxides to varying degrees, particularly in upper horizons (Bw or E) (Harris et al. 2010). These coated sands allow for some retention of P, and they have a finite CEC for retaining Ca, Mg, K, and ammonium ions (Harris et al. 2010). Nonetheless, there is significant risk for loss of these nutrients via leaching. Also, Entisol soil components are minimally reactive with nitrate ions, elevating the risk of nitrate movement to groundwater via vertical flow. Therefore, nitrogen should be applied at rates that do not exceed crop nutrient requirements, and the effects of long-term P application should be reviewed to ensure crop nutrient requirements are not exceeded for long periods of time.
The Entisols forming in the carbonate-dominated parent materials of south Florida (Evergaldes and Big Cypress regions) are commonly very wet and silt textured. Exceptions are better drained areas where nearly bare limestone bedrock has been rock plowed to enable cultivation. The alkaline pH and dominance of Ca create plant fertility challenges (addressed below under "Calcareous Soils of South Florida") that are quite different from those of sandy Entisols. Though a challenge to manage, these calcareous Entisols of extreme southern Florida are used economically to produce tropical fruits and winter vegetables, along with significant tropical nursery crops.
Ultisols
Ultisols are soils that have an argillic or kandic horizon (layer in the sub soil with higher clay content) and a low level of bases due to leaching. Ultisols range from well-drained soils on upland ridges, rises and knolls to very-poorly-drained soils. Some of the more extensive Ultisols in Florida include the Apopka, Arredondo, Blanton, Millhopper, Orangeburg, and Troup soils. Ultisols cover approximately 6.9 million acres of the state (Figure 3).
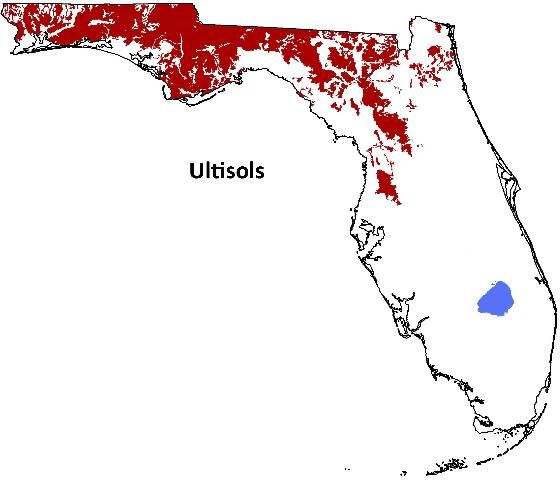
Credit: Greg Means, UF/IFAS
Due to intensive weathering, the clay fraction of Ultisols tends to be dominated by minerals of relatively low reactivity, such as kaolinite and hydroxy-interlayered vermiculite with a low base saturation. Typically, Ultisols are acidic, have enough moisture for crops in most years, and can be highly productive if managed properly (Collins 1985), especially through liming and fertilizer that will increase the CEC and water holding capacity. Important agricultural crops on Ultisols include peanut, cotton, corn, forages and hay, and pastures.
Alfisols
Alfisols are soils that have an ochric epipedon (relatively thin surface layer), an argillic horizon (subsoil with a higher clay content than the layers above), and a moderate to high level of bases. These soils make up one of the most predominant soils in the world, where major agronomic and horticultural crops are grown. Alfisols range from well drained soils on ridges and knolls to very poorly-drained soils on flats, depressions, and floodplains. Aqualfs, the most common Alfisols in Florida, are wet for extended periods during most years (Harris and Hurt 1999). For most agricultural crops to be successful, Aqualfs must be artificially drained. Some of the more extensive Alfisols in Florida include the Boca, Felda, Malabar, Meggett, Pineda, and Riviera soils. Alfisols cover approximately 4.6 million acres (Figure 4). In Florida, Alfisols occur in both the peninsular and panhandle regions.
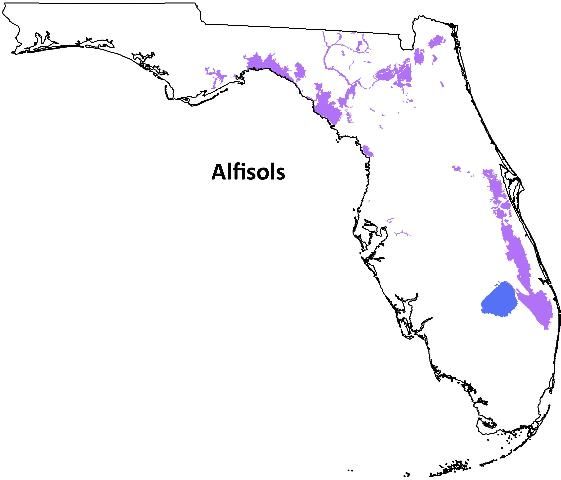
Credit: Greg Means, UF/IFAS
Major vegetable and fruit crops produced on Alfisols include tomatoes, potatoes, cucumbers, water melons, peanuts, and corn. The thin, dark A horizon of Alfisols is rich in organic matter and nutrients, contributing to moderate to high native fertility. Additionally, these soils have higher nutrient and water holding capacities because of the clay-enriched layer (B horizon) and are, therefore, intensively cropped. The presence of the argillic B horizon in Alfisols also favors the retention of P and micronutrients. In Florida, Alfisols are exposed to leaching from frequent rainfall, which can make the soil more acidic than the Alfisols in drier regions. While Alfisols have undergone some weathering, their relatively high base saturation and cation exchange capacity (CEC) enable cultivation with less frequent fertilization compared to other more intensively weathered soils. To maintain fertility, especially during dry seasons, cover crop rotation with row crops.
Histosols
More common names for Histosols are peats and mucks. Histosols are organic-rich and have at least 12% organic carbon content to a depth of = 40 cm. Histosols of Florida typically have an organic matter content of greater than 80%. The underlying materials are sandy to loamy mineral soils or limestone bedrock. Most Florida histosols are formed from highly decomposed wetland plant remains and material that accumulated in water. Histosols are found all over the state, covering approximately 4.0 million acres, and include the Lauderhill, Pamlico, Torry, Okeelanta, Terra Cela, Pahokee, Gator, and Samsula soils (Figure 5).
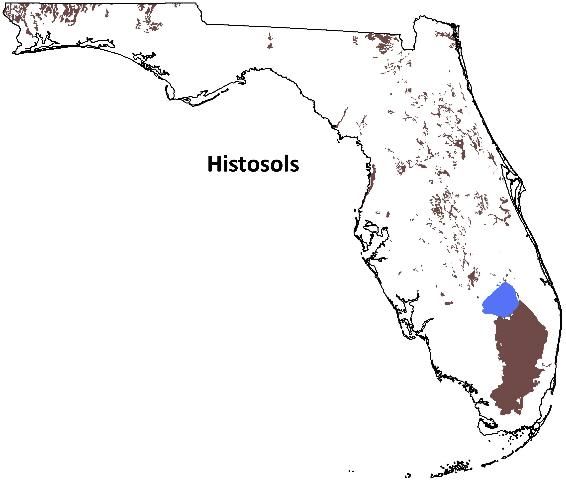
Credit: Greg Means, UF/IFAS
Histosols require drainage to enable survival of most horticultural crops because of the naturally high water tables that limit plant-available water and nutrient retention (Harris et al. 2010). Drained Histosols retain cationic nutrients and maintain their plant availability, although alkalinity can limit the availability of some nutrients (Harris et al. 2010). Histosols lack P-retaining minerals. This, coupled with the landscape location (drained areas that are otherwise flooded; for the most part, low lying and bottoms) of Histosols in Florida, means that P application rates should not be in excess of crop nutrient requirements. The Everglades Agricultural Area is a Histosol-based agricultural area producing much of the nation's late winter sweet corn, celery, leafy vegetables, and snap beans.
Mollisols
Mollisols are considered some of the the most fertile soils in the world and have a thick, dark-colored surface layer with a high level of organic matter and bases. Many Mollisols have argillic horizons, where the illuvial accumulation of clays has taken place through leaching (eluviation) of horizons above. Mollisols in Florida are generally very poorly-drained soils in depressions and floodplains. The more extensive Mollisols include Anclote, Buccaneer, Chobee, Delray, Yulee, and Floridana soils. Mollisols cover approximately 1.1 million acres in the state (Figure 6).
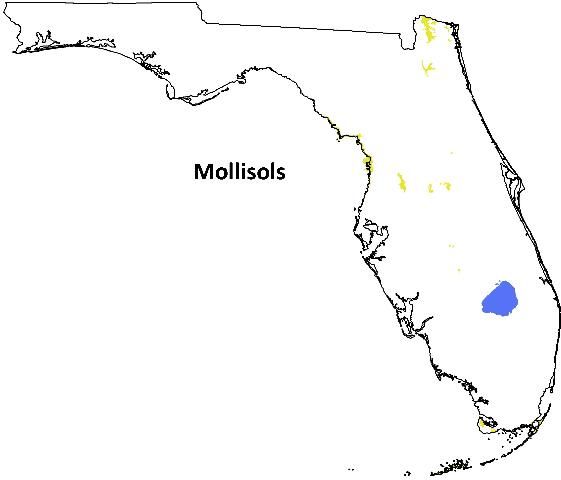
Credit: Greg Means, UF/IFAS
The Ca-rich chemistry of Mollisols allows for the accumulation of stable humic compounds at great depths (Smeck and Burras 2007). The accumulation of organic matter-rich material in the mollic epipedon results in a reasonable reserve of basic nutrients (Ca, Mg, and K) and sometimes elemental N. Mollisols can be managed without much fertilization because of higher humus content combined with limited leaching. Soils can be returned to their highly productive conditions by addition of lime to help correct the pH if the soils are acidic.
Almost all Mollisols in Florida are Aquolls, soils that are saturated seasonally and typically found within wetlands. These conditions can contribute to the build-up of a highly fertile histic (organic-rich) epipedon overlying the mollic epipedon. In areas where there is not a natural outlet, tile and drainage ditches may be required. The Mollisol soil structure allows for movement of air and water when the soil is not saturated (Collins 1985). The native fertility, favorable chemical properties, and strong soil structure make Mollisols one of the most important and productive agricultural soils in drier regions. However, in Florida, their agricultural use is significantly hampered by wetness, and they commonly remain under native vegetation. Where drained, they can be cultivated for row crops and pasture. Where row crops are grown, cover crops should be grown in rotation and left to fallow to maintain fertility.
Inceptisols
Inceptisols are soils with minimally developed B horizons of textures finer than loamy sand, or surface horizons too dark and thick for classification as Entisols.
They also do not meet the profile base saturation requirements for Mollisols. Physical, chemical, and morphological properties range widely. Drainage can range from well- to very-poorly-drained. Examples of sandy Inceptisols in Florida include the Fort Meade (well drained), Florahome (moderately-well drained), and Placid (very-poorly-drained) soil series. Inceptisols cover approximately 1.0 million acres in Florida (Figure 7).
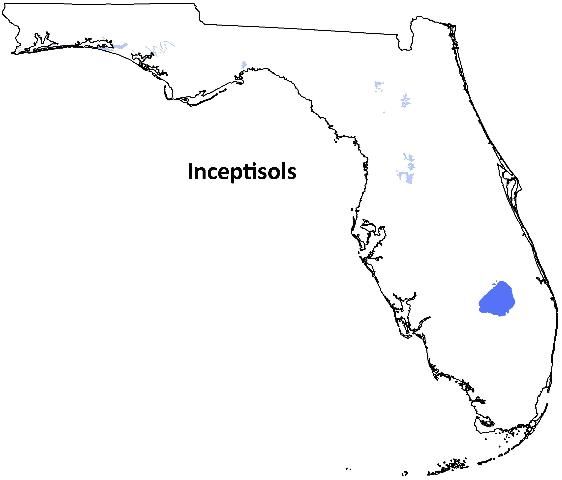
Credit: Greg Means, UF/IFAS
Managing Soils for Agriculture across Florida
In the context of predictive and diagnostic soil testing, the soil orders found in Florida can be grouped into three categories based on their predominant physical and chemical properties: acidic soils, organic soils, and calcareous soils. The predominant physical and chemical properties of a soil are used to determine appropriate soil test methods, which require different calibrations and interpretations. These three classifications are useful for determining appropriate management techniques based on the interpretation of soil test results using University Extension field research on soil tests and crop response. In Florida, there are different interpretations for organic soils compared to mineral soils, and a different soil test method for calcareous soils (Mylavarapu et. al. 2014). Soil Testing Programs serve to interpret test results and categorize soils into levels of adequacy based on the crop nutrient requirement. Recommendations based on soil testing are generally made for P and other nutrients (when applicable by test). Where calibration for other nutrients is not possible, nutrient recommendations are based on successful crop performance and yields. Recommendation for N application is based on crop response to applied N fertilizer because there is no reliable soil test method for N in a humid climate with leachable soils.
Acid-Mineral Soils
Soils in the southeastern United States are naturally acidic because of the high rainfall and temperatures that result in the leaching of basic nutrients from the rootzone (Mylavarapu et al. 2014). In Florida, acid-mineral soils typically include Ultisols, Spodosols, and sandy Entisols. Since these soils require extremely intense lime, fertilizer, and water management for cultivation, appropriate soil test methods are important to optimize economic yields of crops (Mylavarapu et al. 2014). The Mehlich-3 extraction procedure has been found to best mimic the extraction of nutrients from the soil by plant roots in soils with higher CEC and also has the ability to extract micronutrients (Mylavarapu et. al. 2014). Nutrients extracted by Mehlich-3 include P, K, Ca, Mg, copper (Cu), zinc (Zn), and manganese (Mn).The concentration index for interpreting Mehlich-3 test results ranges from low to high; this index can then be converted to a recommended fertilizer application rate for P, K, and Mg. A sufficiency chart is also provided for micronutrients as a guidance. Annual soil testing is recommended, since the buildup of nutrients is less likely to happen in these sandy, low CEC soils (Hochmuth et al. 2014).
Liming requirements for crops grown in acidic soils depends on the target pH of the crop. While some crops have a target pH that requires no liming, other crops may have a target pH higher than 6. In these instances, the Adams-Evans buffer is used to determine the amount of lime required to increase the soil pH. This buffer was developed specifically for soils with lower CEC, pH buffer capacity, and lime requirement (Mylavarapu et al. 2014). If the soil's pH is higher than the target, an Adams-Evans buffer pH is not applicable.
Organic Soils
Since organic soils retain P poorly, P application is often required for successful crop cultivation. Soil testing and interpretation is essential in these soils in order to balance crop nutrient requirements with environmental stewardship. Extraction methods for organic soils in Florida depend on the crop. Where sugarcane is grown, Mehlich-3 is used for extraction to determine P levels. For all other crops, water extraction is used for P application. A water extract removes dissolved forms of P that are available to crops grown in these soils. Acetic acid is used to determine micronutrient levels of K, Mg, silicon, and Na (Mylavarapu et. al. 2014).
Note that low soil test results for P are typical for these soils because they have essentially no P retention capacity. The application rate for P should be based on crop nutrient requirements and not on soil fertilization targets because these soils do not have the capacity to build up and maintain P levels due to their lack of P-retaining minerals. To reduce environmental impacts, the form of applied P to organic soils should be bound. This is because the risk for off-site movement is dictated by the solubility of the P form rather than its concentration. Therefore, Fe- and Al- stabilized biosolids that contain P in a bound form are a better option than manures or some other biosolids with soluble P (Harris et al. 2010).
Calcareous Soils of South Florida
The south Florida calcareous soils are important for the production of vegetables, fruits, and ornamentals, with over 85% of Florida's tropical fruits grown on these soils. These crops are grown in the area because of favorable winter temperatures, rather than favorable soil characteristics. Careful management of nutrients is critically important to the successful production of crops on calcareous soils (Mylavarapu et. al. 2014).
The marl (calcium carbonate "mud") and rocky calcareous soils in Miami-Dade County in Florida usually contain from 30% to 94% calcium carbonate. As such, pH values of these soils are greater than 7 and usually range from 7.4 to 8.4. Textures of calcareous soils can be sandy, loamy, or gravelly in south Florida (Mylavarapu et. al. 2014). The dominance of calcium carbonate results in the fixation of P, thereby reducing plant availability (Harris et al. 2010). Calcareous soils can also suppress Mg uptake and create Fe, Zn, and Mn deficiencies (Harris et al. 2010). Application of these micronutrients, often foliarly, is used to overcome deficiencies where they occur.
On calcareous soils, only P can be interpreted through soil testing because no calibrations exist for the other nutrients. In Florida, ammonium bicarbonate-DTPA solution is used as a soil extractant. Since P accumulates in the soil, it is at least partially available because it is converted to less soluble compounds with time.In areas that have been managed long-term for intensive agriculture, soil P values generally exceed "high" levels for the DTPA interpretation and do not require regular, frequent P fertilization.
Due to the high pH of calcareous soils, N, Mg, K, Zn, Mn, and Fe should be applied with several considerations in mind. If rainfall is not expected, mechanical incorporation or irrigation should be used following the application of dry fertilizer containing ammoniacal N to prevent losses by volatilization. Many crops in this area are grown with drip or micro-irrigation, and nutrients can be applied through the irrigation system, resulting in accurate placement near the root zone for improved plant use. High Ca levels suppress Mg and K plant uptake through competition with Ca ions, which may necessitate above normal levels of Mg and K fertilizer for some crops. If soil-applied fertilizer is ineffective due to tremendous quantities of both exchangeable and nonexchangeable Ca in the soil, foliar application of water-soluble Mg or micronutrient fertilizers may be required. Due to the formation of low-solubility compounds in high pH calcareous soils, soil applications of Zn and Mn fertilizers are generally ineffective, but deficiencies can be corrected through the use of foliar sprays (Obreza et al. 2012).
References
Collins, M. E. 1985. Key to Soil Orders in Florida. SL43. Gainesville: University of Florida Institute of Food and Agricultural Sciences. http://ufdc.ufl.edu/IR00003421/00001
Harris, W. G., M. Chrysostome, T. A. Obreza, and V. D. Nair. 2010. "Soil Properties Pertinent to Horticulture in Florida." HortTechnology 20:10–18.
Harris, W. G. and W. G. Hurt. 1999. "Introduction to Soils of Subtropical Florida." In: K. R. Reddy, G. A. O'Connor, C. L. Schelske (ed.) Phosphorus Biogeochemistry in Subtropical Ecosystems.
Harris, W. G., R. D. Rhue, G. Kidder, R. B. Brown, and R. Littell. 1996. "Phosphorus retention as related to morphology and taxonomy of sandy coastal plain soil materials." Soil Sci. Soc. Am. J. 60:1513–1521.
Hochmuth, G., R. Mylavarapu, and E. Hanlon. 2014. Soil Testing for Plant-Available Nutrients—What Is It and Why Do We Use It? SL408. Gainesville: University of Florida Institute of Food and Agricultural Sciences. https://edis.ifas.ufl.edu/ss621
Jenny, H. 1941. Factors of soil formation: A system of quantitative pedology. McGraw-Hill, New York.
Mylavarapu, R., C. Mitchell, and H. J. Savoy. 2014. "Soils of the Southeastern US." In: F. J. Sikora, K. P. Moore (ed.) Soil Test Methods from the Southeastern United States. Southern Coop Series Bulletin No. 419. Southern Extension and Research Activity Information Exchange Group - 6.
Smeck, N. E., C. L. Burras. 2007. "Soils in Time and Space." In: R. Lal (ed.) Encyclopedia of Soil Science, Second Edition.