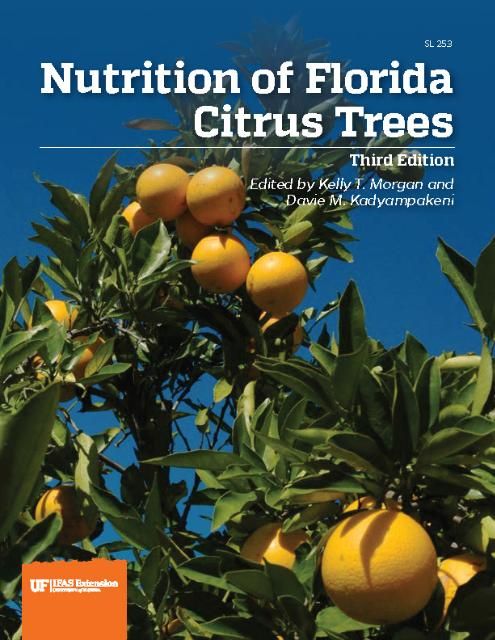
This publication is part of SL253, Nutrition of Florida Citrus Trees, 3rd Edition. For references, a glossary, and appendices, please refer to the full document at https://edis.ifas.ufl.edu/ss478.
Information in the box below applies to citrus trees affected by HLB. Other information in this chapter is valid for healthy citrus trees and trees with HLB.
Recommendations for HLB-affected trees:
Citrus under Protective Screen (CUPS) Production System for HLB-free Trees—Rhuanito Ferrarezi and Arnold Schumann
Completely enclosed screen houses can physically exclude contact between the Asian citrus psyllid (ACP, Diaphorina citri) and young citrus trees, preventing huanglongbing (HLB) disease development. The benefits of eliminating HLB include rapid tree growth, little fruit drop, and higher yields with premium-quality fruit. One of CUPS' main advantages is the reduced frequency of insecticide sprays to control psyllids.
This new production system is designed for the fresh-fruit market due to its high installation and maintenance cost. It relies on precision irrigation, hydroponic systems, electronic soil water sensing, fertigation, and canopy management to maximize plant growth and yield. Structural engineering is also important for screen houses to cope with Florida's weather conditions. Several CUPS practices, such as pest and disease robotic scouting, automated pesticide spraying, fertilizer application through the irrigation system, canopy hedging, and topping, require different techniques, tools, and approaches because CUPS operations are more intensive or different from open-air citrus operations. For example, the fertigation system can be automated using computer controllers, soil moisture sensors, and electrical conductivity sensors to provide the trees with water and fertilizers on demand. Nutrients can be delivered in real time to match crop requirements and maximize high-value fruit production. Most other technologies that could impact citrus production are no different in CUPS than in open-air groves, including soil and leaf sampling, liquid fertilizers for fertigation, controlled-release fertilizer (CRF) or soluble dry fertilizers, irrigation emitters, freeze protection requirements, etc.
There are several important differences between CUPS and conventional open-air groves (see below). Such differences may affect the interpretation of information presented in this edition of Nutrition of Florida Citrus Trees or require modification of the recommendations.
CUPS compared to conventional open-air groves:
- Potential evapotranspiration, ETo, is 23% lower in CUPS compared to open-air groves (Ferrarezi et al. 2017a), and therefore using the FAWN irrigation scheduling is not advised; automated digital soil moisture sensors are the best choice.
- Growth rates are nearly twice as high in CUPS than outside (Ferrarezi et al. 2017b), so young tree fertilizer rates and timings recommendations should be modified for CUPS.
- Increased plant growth rates induce vigorous flushing, and canopy needs to be frequently managed by mechanical or hand pruning to control plant growth and increase fruit yield.
- Liquid fertilizers applied daily or weekly or CRF dry fertilizer blends are recommended to maximize growth and production.
- Vegetative growth of trees in CUPS is promoted by the unique light (23% lower shortwave radiation), wind gusts (4× lower), and temperature (11% higher) environment, which also affect tree photosynthetic rates. Fertilizer N-P-K nutrient ratios may need adjustment in a CUPS environment.
- Leaf and soil nutrient sampling and thresholds are the same for CUPS as for open-air groves but should emphasize nutrition of healthy trees grown for fresh-fruit production.
- Due to the high temperature and humidity induced by the screen-house covering, higher populations of thrips, rust and spider mites, and other secondary pests such as snow scales must be managed to avoid fruit damage and quality reduction. Greasy spot disease becomes a major issue due to high humidity and temperature in CUPS and has to be controlled, especially by fungicide sprays and by removing debris and fallen leaves to reduce the inoculum pressure.
- Due to the presence of poles and trellises, special machinery for spraying, hedging, topping, loading fertilizer, hauling fruit, and repairing the screen and poles are necessary in CUPS, particularly in higher-density plantings. Attention to machinery movement between adjacent screen houses is required to avoid disease spreading.
Details about how to implement those exceptions can be obtained from Schumann et al. 2019 (EDIS article CMG19, available at https://edis.ifas.ufl.edu/hs1304). A comprehensive Quick Start Guide will soon be released on the UF/IFAS EDIS website.
References
Ferrarezi, R. S., B. R. Wright, B. J. Boman, A. W. Schumann, F. G. Gmitter, and J. W. Grosser. 2017a. "Protected Fresh Grapefruit Cultivation Systems: Antipsyllid Screen Effects on Environmental Variables inside Enclosures." HortTechnology 27(5): 675–681.
Ferrarezi, R. S., A. L. Wright, B. J. Boman, A. W. Schumann, F. G. Gmitter, and J. W. Grosser. 2017b. "Protected Fresh Grapefruit Cultivation Systems: Antipsyllid Screen Effects on Plant Growth and Leaf Transpiration, Vapor Pressure De?cit, and Nutrition." HortTechnology 27(5): 666–674.
Schumann, A. W., A. Singerman, A. L. Wright, and R. S. Ferrarezi. 2019. Citrus under Protective Screen (CUPS) Production Systems. CMG19. Gainesville: University of Florida Institute of Food and Agricultural Sciences. https://edis.ifas.ufl.edu/hs1304.
Scions
Orlando Tangelo
While the nutritional requirements of oranges and grapefruit are well known, the requirements of many commercially grown hybrids are obscure. Orlando tangelos may require the highest N and K rates recommended in Chapter 8, with emphasis on fall application to prevent yellowing and defoliation. Transient yellowing of foliage in the winter should not be the sole reason for increasing the fertilizer rate.
Honey Tangerine
Mature honey tangerines (Murcotts) may require up to 300 lb/acre of N and K2O each year to reduce the incidence of tree collapse during heavy crop years. At least one-third of the annual fertilizer rate should be applied in the fall to coincide with greater tree stress from crop load. Some studies have indicated that heavy fruit set leads to root starvation and death, resulting in reduced mineral uptake. Therefore, the fruit crop should be mechanically or chemically thinned in heavy crop years, with reduced rates of fertilization in light crop years.
Navel Orange
There is no evidence that Florida navel oranges require different fertilizer formulations or additional nutritional or foliar N applications compared with other sweet orange cultivars. It is prudent to maintain leaf nutrient concentrations within guidelines established for sweet oranges without excessive fertilization. Optimum but not excessive nutrition will ensure sufficient tree vigor without compromising fruit yield and quality.
Rootstock/Nutrition Interaction
Citrus rootstocks influence nutrient uptake and can make the difference between a productive and nonproductive grove. Best known is the poor performance that can occur when trees budded on trifoliate and trifoliate-hybrid rootstocks, like Carrizo citrange and Swingle citrumelo, are planted on soils with pH above 7. These trees typically exhibit Zn, Mn, Fe, and Mg deficiency symptoms. Soils with high concentrations of shell, limestone rock, and/or subsoil clay are particularly unsuitable for Swingle and Carrizo rootstocks.
The widespread planting of trees on Swingle citrumelo rootstock has brought the important influence of rootstock on citrus tree nutrition to the forefront. Trees on Swingle rootstock often grow relatively poorly with chlorotic foliage when planted on alkaline soils. Iron deficiency has become more prominent with the increased use of Swingle rootstock. Trees on Swingle also sometimes show general starvation symptoms (of N and other elements) during the winter, even though soil temperatures may not attain the critical 55°F threshold associated with reduced root function. This "winter chlorosis" occurs in spite of sufficient soil nutrient supply and supplemental winter fertilizer applications.
While rootstock-related nutrient deficiencies can be overcome by appropriate fertilizer applications (although at high cost), the only way to alleviate toxicities is through reduced input of phytotoxic elements or the use of less-sensitive rootstocks. For example, C. macrophylla is highly B tolerant, and trees on Rangpur lime are Cl tolerant.
Cleopatra mandarin is the most Cl-tolerant rootstock. Sun Chu Sha is efficient in taking up Mg when soil Mg availability is low. Trifoliate orange, and to a lesser extent citranges and citrumelos, are susceptible to Fe chlorosis on high pH (>7) soils. The above rootstocks also accumulate B and Cl and as a result are not tolerant to saline soils.
Soils High in Copper
Repeated use of Cu both as a soil application and as foliar fungicide sprays has caused Cu to accumulate to toxic concentrations in some older citrus-grove soils with acidic soil pH. Trees experiencing Cu toxicity may exhibit Fe chlorosis on the foliage. For most Florida soils under citrus production, the Mehlich 1 soil test Cu interpretations in Chapter 4, Table 4 can be used as a rough estimate of potential Cu toxicity.
Although useful as a guide, soil test Cu may not predict Cu toxicity well in many cases. Cu is more toxic when soil pH is less than 6.0. However, if soil pH is maintained above 6.0 by liming, Cu bioavailability is negligible. When the soil is found to contain high Cu, the following steps should be taken:
- Discontinue using Cu except where it is required to control fruit and foliage fungal diseases where no alternative control exists.
- Lime the soil to pH 6.5 and maintain it there.
- Avoid using Swingle citrumelo rootstock, which is Cu-sensitive.
As Cu is present in the soil solution at low concentration and accumulates in feeder roots with time, its extraction from roots may be used to diagnose Cu toxicity. Field and greenhouse studies have indicated that both total feeder root Cu and Cu extracted with 1M hydrochloric acid are correlated with extractable Cu in sandy soils. In high Cu soils, root Cu concentration is a better indicator of Cu toxicity than leaf Cu. While a critical root Cu concentration is not well defined, toxicity in mature groves has been associated with feeder root Cu in the 350 to 800 mg/kg range.
Calcareous Soils
Calcareous soils are alkaline (pH > 7) due to the presence of excess calcium carbonate (CaCO3). These soils can contain from 1% to more than 25% CaCO3 by weight, with pH in the range of 7.6 to 8.4. In a Florida calcareous soil, the pH is not usually higher than 8.4 regardless of CaCO3 concentration.
Many Florida Flatwoods soils contain one or more horizons (layers) that are calcareous. A typical characteristic is an alkaline, loamy horizon less than 40 inches deep that can be brought to the surface during land preparation for citrus planting. Increased nutritional management intensity is often required to successfully grow citrus on calcareous soils. Some sites (e.g., ditch banks) are comprised of soils containing considerable amounts of lime rock or shell. It may not be economically justifiable to plant these sites with certain rootstocks considering the management problems and costs involved.
Citrus fertilizer management on calcareous soils differs from that on noncalcareous soils because of the effect of soil pH on soil nutrient availability and chemical reactions that affect the loss or fixation of some nutrients. The presence of CaCO3 directly or indirectly affects plant availability of N, Mg, K, Mn, Zn, Fe, and Cu. The behaviors of Fe, Zn, and Mn in high-pH soil and recommendations for alleviating their deficiency have already been discussed in chapter 8. The remaining discussion deals with N, Mg, and K. The availability of soil Cu is also affected but is not discussed here because the citrus Cu requirement is normally satisfied through Cu fungicide foliar sprays.
Management of Fertilizer Nutrients
Soil pH affects biological and chemical reactions involving nitrogen and can influence plant N use efficiency. Nitrification (the conversion of NH4+ to NO3- by soil bacteria) is more rapid in soils with pH between 7 and 8. Volatilization of ammoniacal-N fertilizer can be significant if the pH of the soil surface is greater than 7 where the fertilizer is applied. This condition occurs in calcareous soils or where the breakdown of the N fertilizer material produces alkaline conditions (e.g., urea decomposition).
Nitrogen fertilizer should be managed to minimize ammonia volatilization. If rainfall is not imminent following application of ammoniacal-N to the surface of a calcareous soil, the fertilizer should be immediately moved into the soil with irrigation water. Urea applied to the surface of any soil should also be irrigated in. Fertigation using either of these sources is a suitable application method because the N immediately enters the soil.
Low leaf-potassium concentrations are common in groves planted on calcareous soils. If low yield, small fruit, fruit splitting, or creasing is observed, application of additional K fertilizer is justified. One approach is to increase the N:K2O fertilizer rate ratio to 1:1¼, that is, to apply 25% more K2O than normal.
If the trees do not respond to soil application, an alternative approach to increasing leaf K is foliar sprays of KNO3 or KH2PO4 (Chapter 8). Applications of 20 lb KNO3 per 100 gal of water sprayed to foliar runoff (roughly 20 to 30 lb KNO3 per acre) have effectively increased leaf K, especially if applied more than once during the year. Precautions should be taken to avoid foliar burns from high spray concentrations. The N applied in the spray is as equally available as soil-applied N, so the rate of N applied as KNO3 should be taken into account when determining the annual N fertilizer rate for the citrus grove.
Low leaf-magnesium concentration in groves on calcareous soils can be addressed by applying foliar Mg sprays using Mg(NO3)2.
Acidification to Reduce Soil pH
Soil acidification can improve nutrient availability in calcareous soils by decreasing soil pH. The rate of a soil acidifier required to cause a plant response depends on the amount of CaCO3 in the soil. The chance of a positive plant response to broadcast applications of an acidifier is near zero if lime rock or shell is visible in the root zone. In contrast, it is feasible to acidify soils with lower CaCO3 content (e.g., from overliming) or those that have become alkaline from repeated application of high-bicarbonate irrigation water.
Soil acidifiers include elemental S and ammonium or potassium thiosulfate [(NH4)2S2O3, K2S2O3]. The sulfur in these compounds converts to sulfuric acid in the soil, which neutralizes CaCO3 (Table 1) and decreases soil pH. Ammonium sulfate [(NH4)2SO4] acidifies the soil through nitrification, which releases H+ as NH4+ converts to NO3-.
Elemental S is the most effective soil acidifier. The powder form can be difficult to handle due to dustiness and fire hazard, and it can cause severe root burn if not applied properly. To overcome these problems, some S products have been formulated into porous pellet-like particles that are much easier to handle and apply.
Ammonium thiosulfate and potassium thiosulfate are clear liquid fertilizers containing S2O32-. They can be blended with N, P, and K solutions to form a wide variety of N-P-K-S formulations. Thiosulfates are noncorrosive and not hazardous to handle, and they are well adapted to the methods used to apply fertilizer solutions. Application of ammonium thiosulfate to calcareous soils has been shown to increase the amount of extractable Fe in the soil.
The soil within the wetted pattern of a microirrigation emitter often becomes alkaline when the water source contains bicarbonate, while the surrounding soil may be neutral or acidic. Lowering the soil pH in this situation requires an application of acid or acidifying fertilizer to the wetted pattern only. Application of acid or thiosulfate fertilizer through the irrigation system can be effective in treating this problem.
Saline Soils and Water
All natural waters and soil solutions contain soluble salts. Salt concentration is reported several ways:
- Milligrams per liter (mg/L), or parts per million (ppm) of total dissolved solids (TDS). The units mg/L and ppm are interchangeable.
- Electrical conductivity (EC), expressed as deci-Siemens per meter (dS/m), millimhos per centimeter (mmhos/cm), or micromhos per centimeter (μmhos/cm). The units dS/m and mmhos/cm are interchangeable, and μmhos/cm = mmhos/cm × 1000.
Salts in solution exist as ions that can conduct an electric current, so EC increases as dissolved salt concentration increases. The EC of Florida waters usually ranges between 0 and 5 dS/m.
The conversion from EC to TDS depends on the kind of salts present in the solution. TDS (in ppm) can be estimated by multiplying EC (in dS/m or mmhos/cm) by 700. This conversion factor is an average value appropriate for converting the EC of Florida soil extracts and irrigation waters to TDS. Many conductivity meters that provide a direct salinity reading in ppm have a built-in conversion factor in the range of 630 to 640. Care must be taken to ensure that measurements made by different conductivity meters are comparable.
Vast amounts of salts can be deposited on the soil by long-term irrigation with high-salinity water. For example, 100 gallons of water at 3000 ppm TDS contains about 2.5 lb of salt. Because the weekly irrigation requirement of a bearing citrus tree can exceed three times this amount, soil salts can quickly accumulate. Even 1000 ppm TDS water (containing 0.8 lb salt in 100 gallons) can create salt stress.
Because soil salt concentration depends on soil water content, soil salinity is often related to a standard saturation extract (ECe). The ECe standardizes soil salt concentration to the saturation soil water content. Thus, salinity around tree roots may be several times greater than ECe when soil moisture is at field capacity or less. In sandy soils, where salts are easily leached, management decisions based solely on ECe measurement are not advised. ECe of these soils only indicates soil salinity at the time of measurement and can change rapidly following irrigation or rainfall.
The main citrus tree response to excess salts in soil and irrigation water is growth reduction. Injury symptoms caused by saline irrigation water are not usually permanent, but affected trees may remain stunted compared with trees not receiving salty water, especially if they are young.
Salts in solution exert an osmotic effect that reduces water availability through both chemical and physical processes. Roots are not able to extract as much water from a solution high in salts compared with one low in salts. In effect, the trees must expend more energy to move water through them, which reduces root growth followed by reductions in shoot growth and yield.
The critical salinity concentration will vary with the buffering capacity of the soil (that increases with clay and organic matter content), the climate, the rootstock used, and the soil moisture status. Salinity-induced symptoms like reduced root growth, decreased flowering, smaller leaf size, and impaired shoot growth are often difficult to assess but occur prior to ion toxicity symptoms in the leaves. Chloride toxicity, which appears as burned necrotic or dry-appearing edges on leaves, is one of the most common visible salt injury symptoms. Sodium toxicity symptoms are seldom seen in Florida, but sometimes high Na may cause an overall leaf "bronzing" accompanied by reductions in growth. As with Cl, high leaf Na can cause nutrient imbalances at much lower concentrations than those required to produce visible symptoms. Because Na and Cl are highly soluble in soil water, evaluating salinity stress by measuring their concentration in the soil has little diagnostic value.
Common citrus rootstocks tolerate soil salinity differently. In general, the ranking of rootstocks from most tolerant to least tolerant of salinity is: 1. Cleopatra mandarin; 2. sour orange; 3. sweet orange; 4. Swingle citrumelo; 5. Carrizo citrange; 6. rough lemon. Grapefruit trees tend to be less salt tolerant than orange trees.
Fertilizer application frequency directly affects soil solution TDS concentration. A fertilization program that frequently applies low rates of soluble salts will normally result in less salinity stress than programs applying only two or three high-rate doses per year. Use of controlled-release fertilizers or fertigation can minimize salt stress if high-salinity irrigation water must be used. Growers using salty irrigation water usually observe a marked improvement in water quality when summer rains begin.
Selecting nutrient sources that have a relatively small osmotic effect on the soil solution can help reduce salt stress. The osmotic effect of a fertilizer is defined as its salt index relative to sodium nitrate, which arbitrarily has a salt index of 100. Phosphorus sources have a low salt index and present little problem. Conversely, N and K sources can have a high salt effect (Appendix C). The salt indexes of inorganic and natural organic fertilizers are low compared with commonly used soluble fertilizers. High-analysis fertilizers may have a lower salt index per unit of plant nutrient than lower-analysis fertilizers because they may be made with lower salt-index materials. Thus, at a given fertilization rate, a high-analysis formulation will likely produce less salt injury.
Selecting nutrient sources that do not add a potentially harmful ion to already high concentrations in irrigation water can reduce the likelihood of a salinity problem. The Cl- in KCl or Na+ in NaNO3 add potentially harmful salts to the soil solution. High application rates of fertilizer salts can raise soil pH and decrease soil nutrient availability. Specific ions can also aggravate nutrient imbalances in soil and trees. For example, Na+ displaces K+, and to a lesser extent Ca2+, in soil solutions. Displacement of K+ by Na+ can lead to K deficiency and in some cases even Ca2+ deficiency in leaves when repeatedly irrigating with water high in Na+. Nutrient deficiencies compound the effects of salinity stress. Problems can be minimized if sufficient nutrition is maintained through either soil or foliar fertilizer application.
Nutrient Management with Saline Irrigation Water
- Routinely evaluate irrigation water salinity with an EC meter. TDS below 1000 ppm is excellent. A salt problem may become evident as TDS increases from 1000 to 2000 ppm and is highly likely if TDS exceeds 2000 ppm.
- If excess salts accumulate in the soil, keep the soil moist so they are less concentrated.
- Fine-textured soils and areas of compacted soils or poor drainage may need special management to flush excess salts from the root zone.
- Do not allow salty water to contact leaves, especially when evaporation demand is high.
- Use nighttime irrigation whenever possible to minimize evaporation and salt deposition.
- Choose fertilizer formulations with the lowest salt index per unit of plant nutrients.
- Increase fertilization frequency, which will help reduce the salt content of each application, and will aid in preventing excess salt accumulation in the root zone.
- Maintain optimum but not excessive nutrient concentrations in the leaves.
- Base fertilization rates on the long-term production of the grove. Decrease fertilizer rates applied to trees irrigated with salty water compared with trees irrigated with good-quality water, because production is probably lower.
- Use leaf tissue analysis to detect excessive leaf Na or Cl or deficiencies of other elements caused by salt-induced nutrient imbalance.
Using Reclaimed Water for Irrigation
Reclaimed municipal effluent is an excellent citrus irrigation water source as long as it is produced under strict quality control. As Florida's population continues to grow, treated wastewater will become increasingly important for irrigation.
Long-term use of large quantities of reclaimed water can increase soil pH and soil test P and Ca. Leaf analysis may sometimes show increased Na, Cl, and B concentrations with no observed tree injury. Differences in uptake of various elements in the water (Table 2) can be expected to occur among varieties and rootstocks.
Essential elements in reclaimed water contribute to citrus tree nutrition, so it may be possible to reduce fertilizer rates if reclaimed water is the sole source of irrigation. For example, applying 50 acre-inches/year of reclaimed water containing 10 ppm N would supply 113 lb N/acre. It has been determined that P and B inputs could be reduced or eliminated when using large quantities of reclaimed water. Leaf Na, Cl, and B should be routinely monitored to avoid their reaching toxic levels under reclaimed water irrigation.
Limited studies have shown that it is feasible to grow citrus using citrus processing effluent as an irrigation source. However, certain variables must be considered in the design and management of irrigation systems for use of this water. Daily flow from the processing plant, weekly loading depth to the land, and the storage capacity of the soil should all be considered when determining the needed land area. As with other reclaimed water sources, effluent monitoring procedures at the processing facility are needed to ensure that acceptable quality is maintained.
Nutrient concentrations in citrus processing wastewater are too low to sustain tree growth (Table 11.3), so supplemental fertilization is necessary. Rootstocks and scion varieties should be selected for their tolerance to excess Na contained in processing effluent.
Fertilization Strategies for Damaged Trees
Wind Damage
Strong sustained winds from tropical storms or hurricanes can damage citrus trees by removing canopy and fruit. Root damage may also occur if a storm produces flooding rains. The main nutritional factors related to severe canopy thinning are loss of leaf N and K reserves and interruption of the natural nutrient recycling that occurs as tree residues decompose and mineralize in root zone soil. As a result, subsequent vegetative growth may deplete remaining tree and soil reserves of N and K. Additional fertilization beyond the normal program is justified to aid grove recovery in this situation as long as the root system is not significantly damaged.
To determine how much additional N and K2O to apply, start with the assumption that every 10% loss of leaf canopy from an average grove represents a loss of about 10 lb N/acre, then estimate a target fertilizer rate based on the relative amount of leaf canopy that the grove has lost and the efficiency of the fertilizer application method to be used. Keep the following considerations in mind when attempting to replace lost canopy N and K with additional fertilizer as the grove recovers:
- Reduce the fertilizer rate for smaller trees.
- It will take time to rebuild a full, healthy canopy.
- Distribute additional N and K fertilizer throughout the following growing season as normal. Do not front-load all of it in the spring.
- Consider foliar application in place of soil application.
- Remember that bloom and fruit set in the following year will depend more on environmental conditions and less on fertilizer rates.
When root systems are extensively damaged from prolonged flooding, the tree canopy will recover more slowly. In this case, fertilization should be reduced until the root system can rejuvenate. Surviving roots are more likely to be close to the soil surface, with the lower ones damaged or killed. Addition of fertilizer at rates normally applied to vigorous trees may further damage roots.
Freeze Damage
Trees should not be fertilized or irrigated following a severe freeze until the extent of damage is determined and regrowth is evident. Stored nutrients in bearing trees on a regular fertilizer program can mobilize to new shoots and leaves, especially after severe wood damage. No more than 50% of the recommended N rate should be applied to severely damaged citrus trees that will not produce a crop the following year, provided that optimum tree water status is maintained. It may be necessary to apply only N and foliar micronutrients. There is strong evidence that soil-applied fertilizer may not even be necessary for bearing trees in the first postfreeze season if they have received sufficient fertilization in the years prior to the freeze. Reduced fertilizer rates may be applied to 2-to-4-year-old trees with moderate freeze damage provided no crop is set following the freeze.
Nutritional Deficiencies Enhanced by Environmental or Pathological Factors
Zinc deficiency patterns can be enhanced by citrus blight disease. When trees have blight, leaf symptoms will look the same as Zn deficiency. Leaves are reduced in size and off-color, and small blotches of yellow between green-colored veins in the leaf will appear. In many cases, the leaf Zn deficiency pattern may not be evenly distributed within the tree canopy.
Iron deficiency can result from flooding injury to the citrus root system. Root damage can occur if the root zone soil is flooded for several days in the summer but may take weeks to occur in the cooler winter months. The pattern will first appear on young, expanding leaves. The leaf turns light green, while the veins and midrib remain darker green.
Nitrogen deficiency, or "winter chlorosis," can occur in late winter or early spring when rapid tree growth begins and the soil temperature is too cool for normal root function and nutrient uptake. When this occurs, the midrib will begin to yellow while the remaining portion of the leaf remains darker green.
Phytophthora-induced N deficiency occurs when the roots or tree trunk become infected, resulting in partial or complete girdling that causes the tree to decline. Leaves express visual symptoms of yellowing of the veins, typical of N deficiency. The deficiency pattern may be associated with individual limbs in the case of foot rot or could involve the entire tree in the case of root damage. The deficiency appears as the ability of the roots or trunk to transport N upward into the tree canopy is reduced.
Organic Citrus Production
General Information
The exclusive use of certified organic nutrient sources in an organic production program may not be a viable alternative for large-scale Florida commercial citrus production mostly because of insufficient supply of nonsynthetic fertilizers (manures, composts, etc.), sometimes slow availability of nutrients with time from these materials, and the logistics of their transport, storage, and application. For the small producer, organic citrus production may be feasible depending on the availability and quality of acceptable sources, customer acceptance of the product, and expected returns on investment. Yields, production costs, and market returns for organic citrus production have not been clearly defined in Florida.
Certified organic groves must have distinct, defined boundaries between fields managed organically and those managed conventionally. Storage facilities and records for certified organic fields must be maintained separately from noncertified fields. A production unit may be certified as organic only if harvest occurs at least 36 months after the most recent use of prohibited pesticides or fertilizers. Records of all fertilizer and soil amendment use must be kept for at least 3 years prior to certification.
Organic fertilization programs for citrus emphasize methods to improve soil fertility and health through the use of organic fertilizers and soil amendments. Soil management includes increasing soil organic matter (humus) content by mowing, grazing, growing green manure and N-fixing cover crops in row middles, and applying manures, composts, and natural fertilizers. Annual nutrient application rates for organically grown young trees and bearing trees should be similar to those recommended for conventionally grown trees.
Citrus production operations applying for initial or ongoing organic certification must comply with regulations established under the National Organic Program (NOP) and applicable organic production regulations. Information about various aspects of the NOP is available on the internet at http://www.ams.usda.gov/NOP/NOPhome.html. This web page provides links to several other web pages where additional information can be found.
The USDA does not provide organic certification but instead accredits state, private, and foreign organizations, groups, or persons to become "certifying agents." FDACS has not assumed this role, so growers must choose a private USDA-accredited organic certification agency.
Soil Fertility and Crop Nutrient Management Guidelines
=These guidelines are summarized from Section 205.203 of the NOP final rule.)
- Producers should implement tillage and cultivation practices to maintain or improve the physical, chemical, and biological condition of soil and minimize soil erosion.
- Producers should manage crop nutrients and soil fertility through rotations, cover crops, and application of plant and animal materials.
- Producers should manage plants and animal materials to maintain or improve soil organic matter in a manner that does not contribute to contamination of crops, soil, or water by plant nutrients, pathogenic organisms, heavy metals, or residues of prohibited substances. Animal and plant materials include:
- raw animal manure (feces, urine, other excrement, and bedding produced by livestock that has not been composted):
- can be applied to land used for a crop not intended for human consumption;
- must be incorporated into the soil not less than 120 days before harvesting a product that comes into contact with the soil surface or soil particles; and
- must be incorporated into the soil not less than 90 days before harvesting a product that does not come into contact with the soil surface or soil particles.
- composted plant and animal materials produced through a process that:
- established an initial carbon-to-nitrogen ratio between 25:1 and 40:1; and
- maintained temperature between 131°F and 170°F for 3 days using an in-vessel or static aerated pile system, or
- maintained temperature between 131°F and 170°F for 15 days using a windrow composting system, turning the materials at least five times.
- noncomposted plant materials.
- raw animal manure (feces, urine, other excrement, and bedding produced by livestock that has not been composted):
A producer may apply:
- crop nutrients or soil amendments allowed for use in organic production;
- mined substances of low solubility;
- mined substances of high solubility, provided that it is used in compliance with the conditions established on the national list of nonsynthetic materials prohibited for crop production;
- ash obtained from the burning of a plant or animal material, provided it has not been treated or combined with a prohibited substance or the ash is not included on the National List of nonsynthetic substances prohibited for use in organic crop production; and
- plant or animal material that has been chemically altered by a manufacturing process, as long as it is included on the national list of synthetic substances allowed for use in organic crop production.
A producer may not use:
- any fertilizer or composted plant and animal material that contains a synthetic substance not included on the national list of synthetic substances allowed for use in organic crop production;
- biosolids; or
- burning as a means of disposal for crop residues produced on the operation. (Exception: burning may be used to suppress the spread of disease.)