Saltwater Intrusion and Flooding: Risks to South Florida’s Agriculture and Potential Management Practices
Introduction
Sea level rise and flooding are significant threats to agriculture in southern Florida, where surface and groundwater resources are hydrologically connected due to the shallow and coarse soils and the highly permeable limestone bedrock. Healthy soils have multifaceted benefits for agriculture. Healthy soils regulate water movement, serve as a source and sink for nutrients, and provide optimal biological and chemical conditions for plant growth and crop productivity (Bhadha et al. 2021). Increased soil salinity due to saltwater intrusion and/or flooding negatively affects soil health and can significantly reduce agricultural productivity. Developing and implementing successful management practices to mitigate the effects of saltwater intrusion and flooding are critical to sustainable crop production. This article highlights the impacts of saltwater intrusion and flooding on the health of agricultural soils. It also discusses management practices to reduce the negative impacts of soil salinity due to saltwater intrusion and/or flooding. This information could be of interest to researchers, students, Extension agents, growers, water and land managers, and policymakers.
Major Causes of Saltwater Intrusion
Major causes of saltwater intrusion include sea level rise, excessive groundwater pumping, king tides, and storm surge. Sea level rise is often associated with global climate change. As ambient temperatures increase, ice caps and glaciers melt, eventually causing a rise in the ocean level. The other major driving force for saltwater intrusion is excessive pumping of groundwater for irrigation and other urban uses (e.g., drinking and cleaning) related to increasing populations (Boman and Stover 2002). Sea level rise and excessive groundwater pumping cause the ocean seawater level to be above the groundwater level, leading saltwater to flow towards the fresh groundwater source (Figure 1).
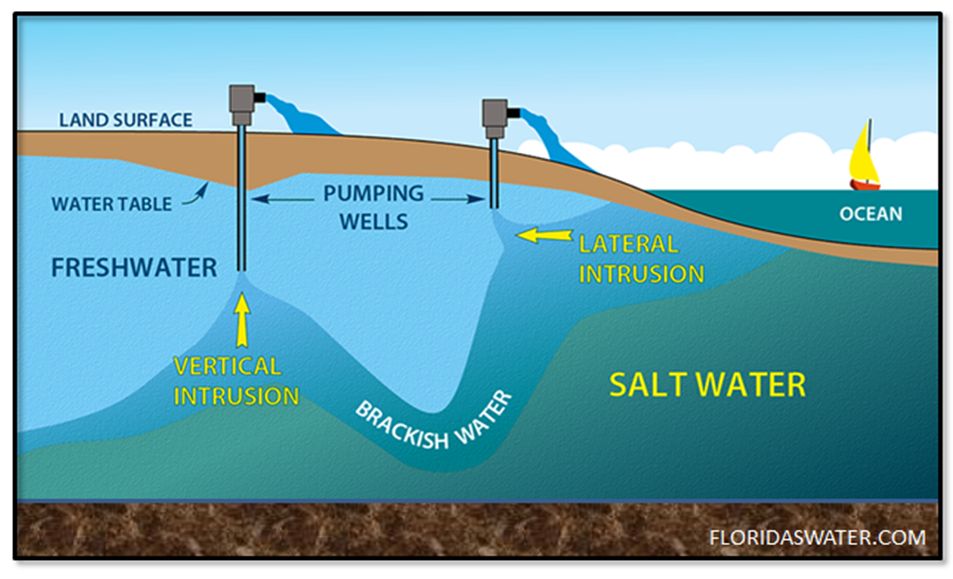
Credit: floridaswater.com
Extent of Saltwater Intrusion in South Florida
Flooding is a common issue in south Florida due to the low land elevation, which is only slightly above sea level. Flooding in the area has a negative impact on a multitude of land uses, including agricultural, residential, and natural areas. Because Florida is a peninsula with proximity to the ocean on three sides, south Florida is very prone to saltwater intrusion (Borisova and Wade 2018). Figure 2 shows a map developed by the United States Geological Survey (USGS 2021) demonstrating the progression of the freshwater-seawater interface in the Homestead, Florida area. As seen from the two lines (red=2011 and green=2018), the seawater is moving further inland towards agricultural areas, posing a threat to agricultural productivity and farmers’ livelihood.
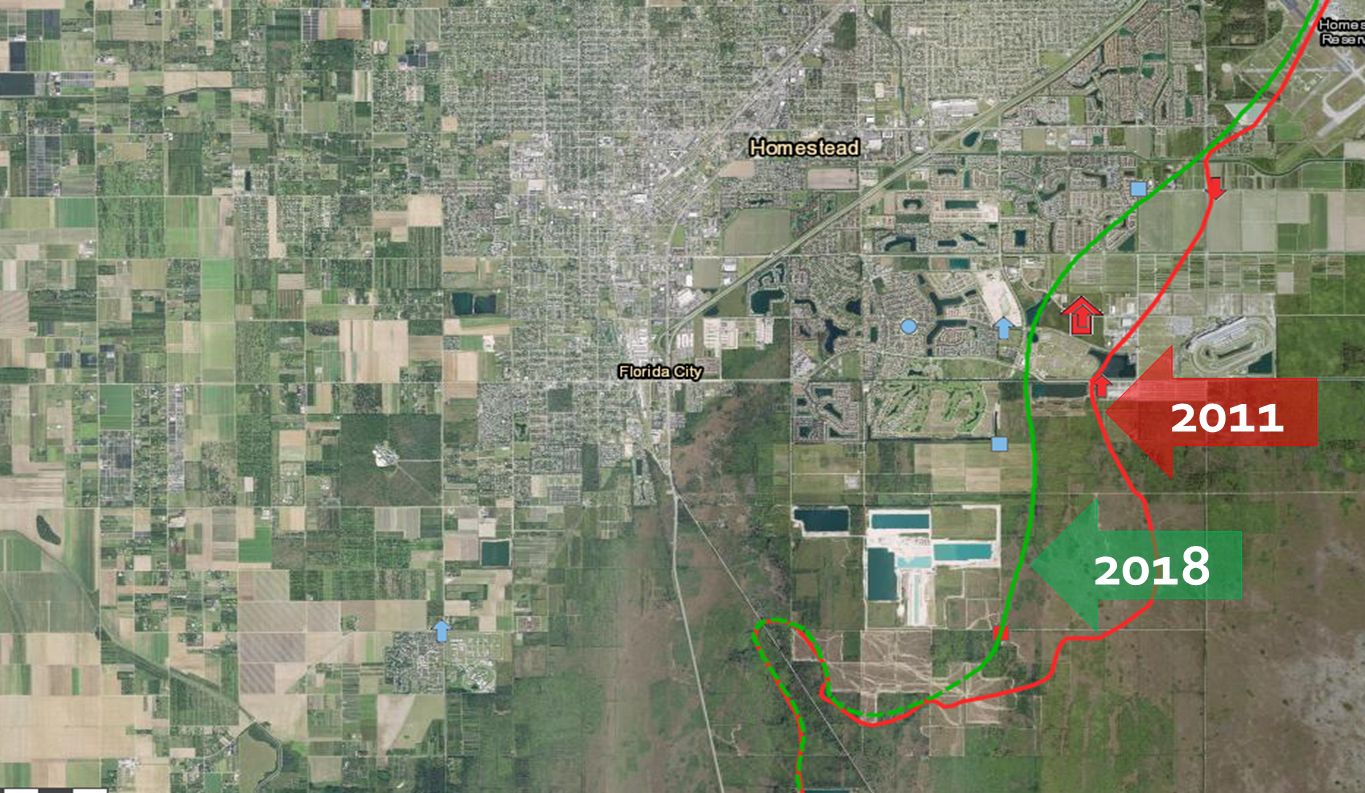
Credit: USGS (https://fl.water.usgs.gov/mapper/)
Impacts of Salinity on Soil Health
Studies show that salt buildup in the soil increases greenhouse gas emissions, contributing to climate change and global warming. Steinmuller and Chambers (2018) found that the application of water with 15 parts per thousand salt concentrations increased CO2 emission by 30% from wetland soils. Higher phosphorous (P) and ammonium (NH4+) concentrations were also reported from pore water samples of soils treated with 15 parts per thousand salt concentrations (Steinmuller and Chambers 2018). Nutrient leaching from salt-affected soils could contribute to the impairments of our freshwater bodies. Saltwater in affected soil also decreases microbial population and diversity and crop yield because few crops can withstand such high salinity. The cumulative impacts of all these factors result in increased cost of agricultural production and decreased agricultural productivity and profit.
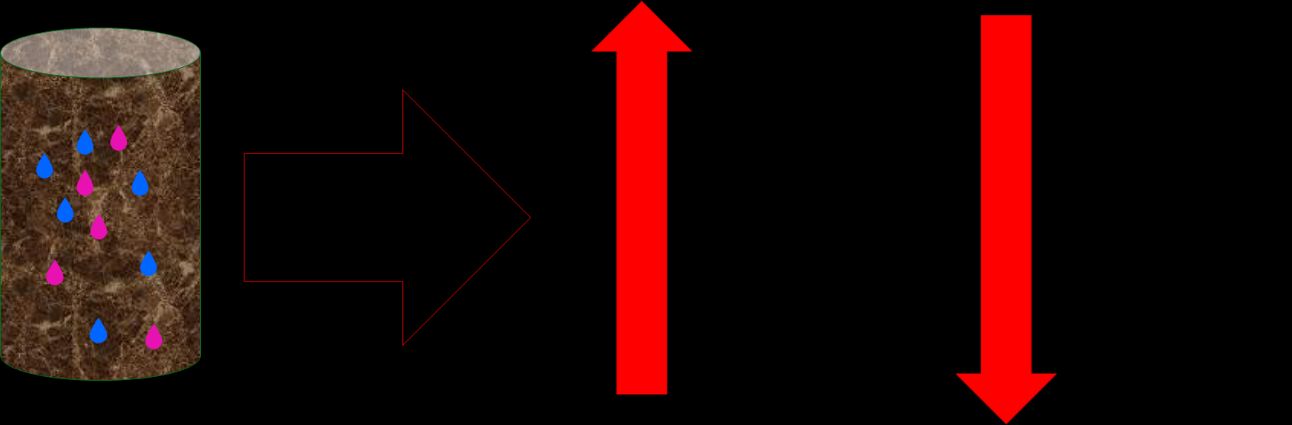
Credit: H. K. Bayabil, UF/IFAS
Impacts of Groundwater Level Rise on Soil Water Storage
Soils in south Florida are highly permeable, with Oolitic limestone bedrock, that hydrologically connects surface and shallow groundwater. Big storm events and storm surges cause the groundwater to rise closer to the soil surface. This rise in groundwater level causes a decrease in the soil’s capacity to store water (Figure 4). Under normal conditions, the groundwater level is several feet below the ground surface, creating an unsaturated zone that stores water during storm events. When the groundwater level is near the soil surface, it causes a significant reduction of the unsaturated storage zone and the capacity of the soil to store rainwater. In addition to the flooding risks to plant roots, reduced soil water storage capacity will also lead to more flooding and runoff.
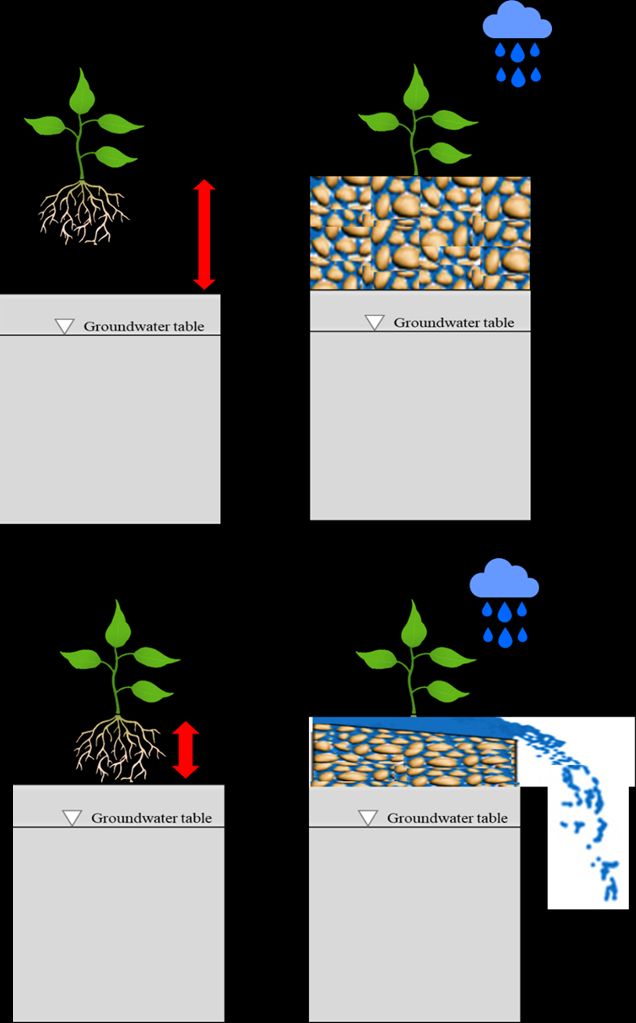
Credit: H. K. Bayabil, UF/IFAS
Impact of Salinity and Flooding on Fruit, Vegetable, and Ornamental Plants
The vast majority of commercially grown tropical fruits and vegetables and most landscape ornamental plants have little to no salinity tolerance (Amacher et al. 2000; Crane et al. 2020; Ebert 2000; García-Caparrós and Lao 2018; Giordano et al. 2021; Ramteke and Sachin 2016; Küçükahmetler 2002; Schaffer et al. 2006; Shannon et al. 1999; Sun et al. 2015). Saline soil and/or salty irrigation water could result in mild to lethal physiological effects, including reduced cell growth and plant organ (e.g., leaf and fruit) expansion, reduced water and nutrient uptake, nutrient imbalances and deficiencies, reduced plant growth and yields, and plant death. Most vegetable crops are intolerant of flooding or constantly saturated soil conditions (Bradford and Hsiao 1982; Heinrich 1970; Li and Rao 2003; Patel et al. 2014). In contrast, while most tropical and subtropical fruit crops have no to very limited flood tolerance (Schaffer et al. 1992), a few tropical fruit crops (e.g., mango, guava, carambola) may tolerate short periods of flooding (Crane et al. 2020; Schaffer et al. 1992; Schaffer et al. 2006).
Potential Management Practices
Management practices to mitigate the negative impacts of salinity due to saltwater intrusion and/or flooding are critical for sustainable and profitable production. Short-term management practices may be easily implemented at farm and field levels and have immediate impacts on production. Long-term management practices such as breeding plants tolerant to flooding and/or salinity have potential, but may take years to develop and require large capital investments in research and development. Cultural practices that involve farm infrastructure modifications often require a prolonged timeline for development and adoption. It takes longer to implement practices that require growers to invest capital and/or change their current practices. Some practices could require new or modified farm equipment. While this article addresses the primary management practices to address saltwater intrusion, it does not cover all the potential management practices.
Short-Term Management Practices
- Flushing salt-affected soils with freshwater: This is the quickest and cheapest method in areas with abundant freshwater resources. This method will be effective only if the soils have good drainage. Its disadvantages include the high demand for freshwater and areas with shallow groundwater, which could lead to contamination of the fresh groundwater. This method will not be feasible if freshwater becomes scarce or freshwater is contaminated by saltwater.
- Solid oxygen fertilizers (SOF): Like humans, plants need oxygen for respiration. However, plants get their oxygen mainly through their roots from soils. Under flooded conditions, plants cannot get enough oxygen. Under salt stress conditions, plants wilt and die (Liu et al. 2019). In addition, when soil salinity is high, plants will have difficulties absorbing oxygen and other nutrients from the soil. Solid oxygen fertilizer provides oxygen to plant roots and improves plants' tolerance to salinity and flooding. Dr. Yuncong Li’s lab at the UF/IFAS Tropical Research and Education Center has been leading the development of SOF and testing the effectiveness of SOF with different crops. Figure 5 shows the effects of SOF on basil and bald cypress plants grown on soil with high salinity levels. The results show that plants with SOF were able to grow well, while plants without SOF did not grow well due to salt stress. There has also been successful use of SOF with some herbaceous tropical fruit crops such as papaya (Thani et al. 2016; Thani et al. 2017), but attempts with woody fruit crops such as avocado have not been successful (Schaffer, unpublished data).
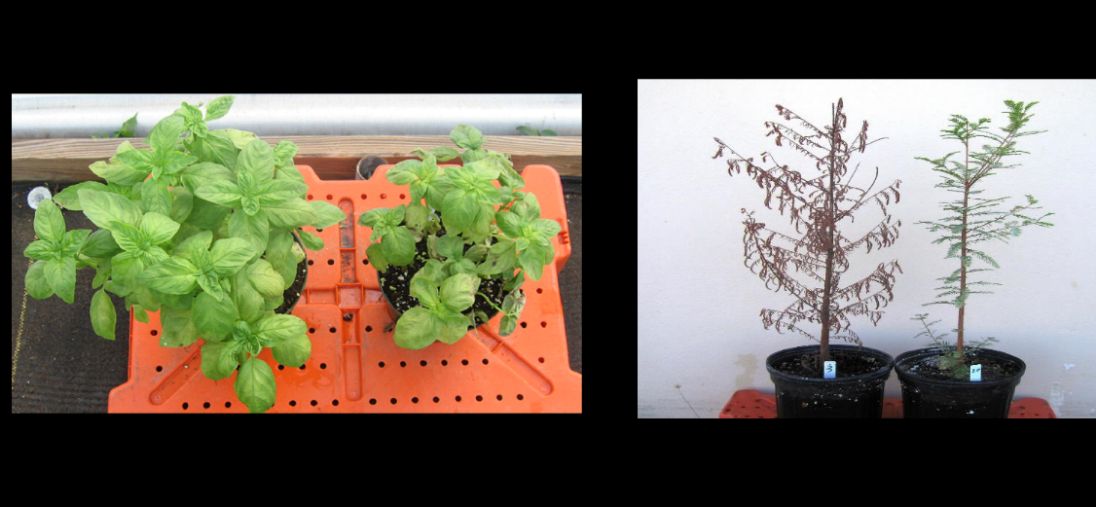
Credit: Guodong Liu, UF/IFAS
3. Soil inorganic amendments—Gypsum: Gypsum contains calcium, which works very well in acidic and clay soils by replacing the sodium in the salt and making it easy for leaching. However, it is less effective in sandy and calcareous soils. Gypsum can be found in garden stores. A study of cotton with and without gypsum found that cotton roots were dense and longer with gypsum compared to the control (Figure 6). Similarly, coffee roots in soil with gypsum were longer and more fibrous than those in soils without gypsum.
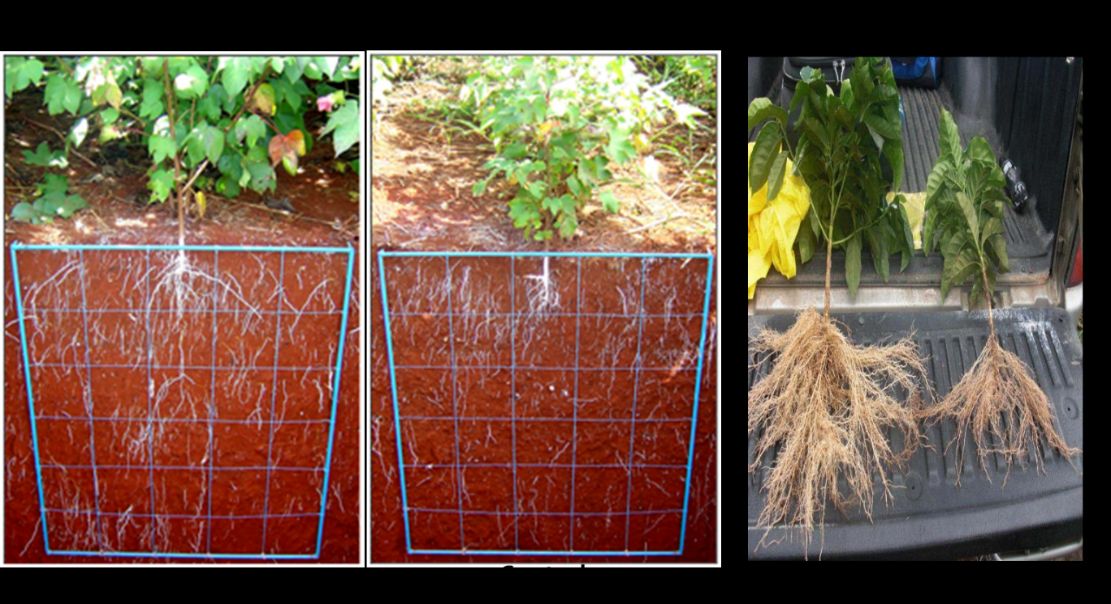
Credit: Malcolm Sumner, University of Georgia
4. Soil organic amendment—Compost: Compost is made of organic material such as yard waste that went through the composting process. Information about compost and composting can be found in Brown (2007). Compost can reduce salinity by improving soil properties and increase plant resistance to salt damage by improving plant health. Figure 7 shows tomato plants grown with and without compost at the UF/IFAS Tropical Research and Education Center. Plants grown in compost grew more and had higher yields compared to plants grown without compost.
5. Soil organic amendment—Biochar: Biochar is reported to reduce plant stress from salinity and improve plant growth and soil health. In addition, modified biochar has been reported to reduce salt accumulation in soil by trapping salts and reducing salt stress on plants (Li, unpublished data).
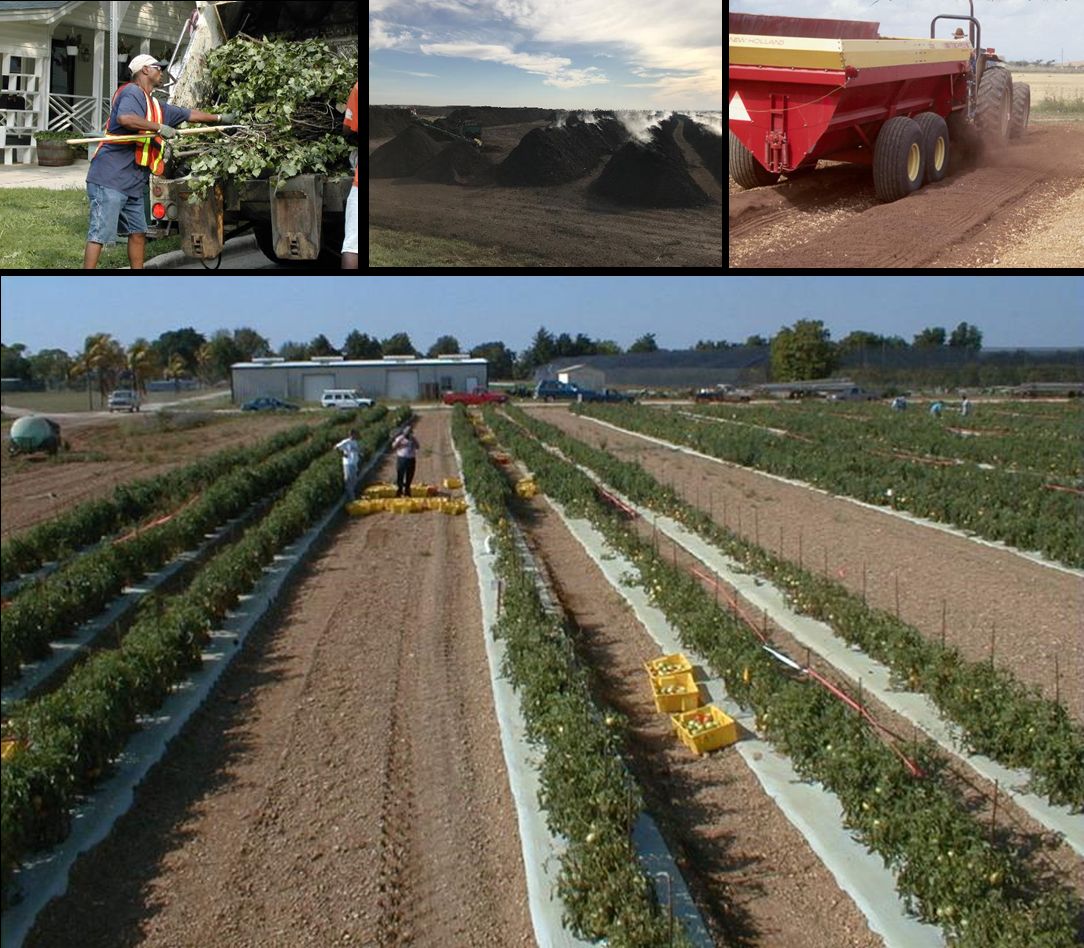
Credit: Yuncong Li, UF/IFAS
6. Cover crops: When incorporated into the soil as a green manure, cover crops can improve soil health and plant growth. As a mulch, they reduce evaporation, which results in less salt accumulation in the root zone (Wright et al. 2018). Sunn hemp is a legume that can fix as much as 300 lb nitrogen per acre (Figure 8). It also generates a lot of biomass, which can be incorporated into the soil to increase organic matter or can be left on the surface to suppress weeds and reduce evapotranspiration.
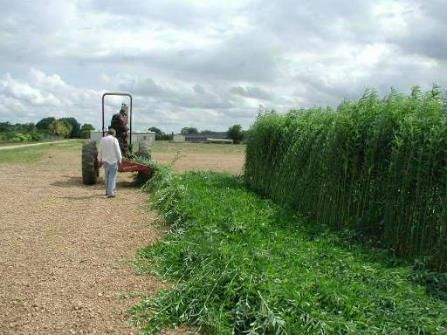
Credit: Yuncong Li, UF/IFAS
7. Plant growth regulators (PGR): Limited research has suggested foliar applications of some plant growth regulators (e.g., glutathione and α-tocopherol), soluble sugars, and plant nutrients may improve flood and salinity tolerance (García-Caparrós and Lao 2018; Li and Rao 2003). Post-flood soil and/or foliar applications of conventional nitrogen fertilizers (e.g., potassium nitrate, urea) and/or plant growth regulators (e.g., synthetic cytokinin) may enhance plant recovery from flooded (low-oxygen) soil (Patel et al. 2014). The use of PGRs and fertilizers needs further study before specific recommendations can be made.
8. Pruning and fruit removal: For fruit crops, such as avocado, removing about 1/3 of the canopy immediately after floodwater subsides increases the root/shoot ratio and helps trees recover from flooding (Sanclemente et al. 2014). If trees have fruit, all fruits should be removed. However, pruning shortly before flooding even in anticipation of a heavy rainfall event can exacerbate flooding stress and tree death (Sanclemente et al. 2013). Therefore, it is important to wait until immediately after floodwaters subside to prune the canopy and remove the fruit of flooded trees.
Long-Term Management Practices
- Plant breeding: Improving the salinity and/or flood tolerance of plants through conventional or molecular approaches for plant breeding is the best method for improving crop performance. However, developing salinity and/or flood-tolerant plants will take a long time and substantial financial resources. The timeline may be five years (for some herbaceous plants) to decades (for some woody fruit and ornamental plants).
- Improving irrigation efficiency: Improving irrigation efficiency will reduce groundwater withdrawal. Salt builds up in soils as salty water evaporates and leaves salt in the soil. In this regard, efficient irrigation practices target reducing evaporation losses and decreasing salt buildup. Another advantage of efficient irrigation systems is that, when irrigation water contains salt, a reduction in the amount of irrigation will result in a proportional decrease in salt load in the soil.
- Growing plants on raised beds: Raised beds could help reduce the damage to plant roots from saltwater or flooding stress. However, this requires modifying farm equipment in order to allow making raised beds.
- Planting salt-tolerant crops: Different plants have different levels of salt tolerance. Table 1 shows the salt tolerance of current cultivars of tropical fruits. However, most agronomic crops are not salt-tolerant.
Table 1. List of salt-tolerant and salt-sensitive cultivars of tropical fruits (Crane et al. 2020).
5. Planting flood-tolerant crops: Plants differ in their flood tolerance level. Food crops such as rice can tolerate floods.
6. Integrated pest management (IPM): Saltwater intrusion and flooding often increase the potential for disease and insect damage. Integrated pest management (IPM) is needed to mitigate the effects of diseases and pests. Furthermore, with climate change, new plant diseases and insects could be more prevalent, and the IPM approach will be key to integrating control of the biotic factors with the abiotic (environmental) effects of salinity and flooding.
Summary
The agricultural industry in south Florida will likely remain vulnerable to salinity due to saltwater intrusion, and flooding. Studies show that soil health is negatively affected by saltwater intrusion and salinity, and damages could be mitigated with the correct management practices. Short- and long-term management practices are needed to reduce salinity and flooding risks to soil health, crop productivity, and the environment. However, there is still an information gap about best management practices and associated costs. More research needs to be done to further understand soil health and environmental risks of salinity due to saltwater intrusion and to identify more effective management practices. Economic research also needs to be conducted to provide growers with guidance in making production decisions. Such research would examine the most cost-effective management strategies to confront saltwater intrusion and determine if the financial returns from these practices outweigh the additional costs. Such research must be complemented with a study of growers’ preferences about these practices, their time and budget constraints, and their willingness to adopt new practices at the field and farm scales. This analysis is necessary to provide insights into which policies would best encourage the adoption of these practices. Flood and salinity control with region-wide water resource management and plans along with government programs and policies will be necessary to assist and maintain agricultural production in south Florida.
Acknowledgments
This material is based upon work that is supported by the National Institute of Food and Agriculture, U.S. Department of Agriculture, under award number 2020-67019-31163 and the Hatch Project No. FLA-TRC-005751. Any opinions, findings, conclusions, or recommendations expressed in this publication are those of the authors and do not necessarily reflect the view of the U.S. Department of Agriculture.
References
Amacher, J. K., R. Koenig, and B. Kitchen. 2000. “Salinity and Plant Tolerance.” All Archived Publications. Paper 43. https://digitalcommons.usu.edu/extension_histall/43
Bhadha, J. H., J. Capasso, R. S. Schindelbeck, and A. R. Bacon. 2021. "Tools for Evaluating Soil Health: SL443/SS657, 1/2021." EDIS 2021(5).
Boman, B. J., and E. W. Stover. 2002. "Managing Salinity in Florida Citrus: Circular 1411/AE171, 7/2002." EDIS 2002(1). https://doi.org/10.32473/edis-ae171-2002
Borisova, T., and T. Wade. 2018. "Florida’s Water Resources." FE757. Gainesville: University of Florida Institute of Food and Agricultural Sciences. https://edis.ifas.ufl.edu/fe757
Bradford, K. J., and T. C. Hsiao. 1982. "Stomatal Behaviour and Water Relations of Water Logged Tomato Plants." Plant Physiol. 70:1508–1513.
Brown, S. P. 2007. "Compost Tips for the Home Gardener: ENH1065/EP323, 8/2007." EDIS 2007. https://doi.org/10.32473/edis-ep323-2007
Crane, J., Y. C. Li, E. Evans, F. Ballen, and J. Wasielewski. 2020. "Planning for a Successful Commercial Subtropical/Tropical Fruit Grove." HS1387. Gainesville: University of Florida Institute of Food and Agricultural Sciences. https://edis.ifas.ufl.edu/hs1387
Ebert, G. 2000. "Salinity Problems in (Sub-) Tropical Fruit Production." Acta Horticulturae 531:99–105.
García-Caparrós, P., and M. T. Lao. 2018. "The Effects of Salt Stress on Ornamental Plants and Integrative Cultivation Practices." Sciencita Horticulturae 240:430–439.
Giordano, M., S. A. Petropoulos, and Y. Rouphael. 2021. "Response and Defence Mechanisms of Vegetable Crops against Drought, Heat and Salinity Stress." Agriculture 11:463. https://doi.org/10.3390/agriculture11050463
Heinrich, D. H. 1970. "Flooding Tolerance of Legumes." Can. J. Plant Sci. 50:435–438.
Holden, P. A., and N. Fierer. 2005. "Vadose Zone | Microbial Ecology." In Encyclopedia of Soils in the Environment, edited by D. Hillel. 216–224. Elsevier, Oxford. https://doi.org/10.1016/B0-12-348530-4/00172-7
Küçükahmetler, Ö. 2002. "The Effects of Salinity on the Yield and Quality of Ornamental Plants and Cut Flowers." Acta Horticulturae 573:407–414.
Li, Y., and R. Rao. 2003. "Management of Flooding Effects on Growth of Vegetable and Selected Field Crops." HortTechnology 13:610–616.
Liu, G., Y. Li, K. Moore, K. Gabel, L. Wu, and R. Muñoz-Carpena. 2019. "How to Chemigate Salinity-Stressed Plants with Hydrogen Peroxide to Increase Survival and Growth Rates." HS1280. Gainesville: University of Florida Institute of Food and Agricultural Sciences. https://edis.ifas.ufl.edu/hs1280
Patel, P. K., A. K. Singh, N. Tripathi, D. Yadav, and A. Hemantaranjan. 2014. “Flooding: Abiotic Constraint Limiting Vegetable Productivity.” Advances in Plants & Agricultural Research 1(3): 00016. http://dx.doi.org/10.15406/apar.2014.01.00016
Ramteke, V., and A. J. Sachin. 2016. “Salinity Influence in Tropical Fruit Crops.” Plant Archives 16(2): 505–509.
Sanclemente, M. A., B. Schaffer, P. M. Gil, A. I. Vargas, and F. S. Davies. 2014. “Pruning after Flooding Hastens Recovery of Flood-Stressed Avocado (Persea americana Mill.) Trees.” Scientia Horticulturae 169:27–35.
Sanclemente, M. A., B. Schaffer, P. M. Gil, J. H. Crane, and F. S. Davies 2013. “Leaf Removal before Flooding Influences Recovery of Avocado (Persea americana Mill.) Trees from Flooding Stress.” Scientia Horticulturae 150:154–163.
Schaffer, B., P. C. Andersen, and R. C. Ploetz. 1992. “Responses of Fruit Crops to Flooding.” Horticultural Reviews 13:257–313.
Schaffer, B., F. S. Davies, and J. H. Crane. 2006. “Responses of Subtropical and Tropical Fruit Trees to Flooding in Calcareous Soil.” HortScience 4:549–555.
Shannon, M. C., and C. M. Grieve. 1999. “Tolerance of Vegetable Crops to Salinity.” Scientia Horticulturae 78:5–38.
Steinmuller, H. E., and L. G. Chambers. 2018. “Can Saltwater Intrusion Accelerate Nutrient Export from Freshwater Wetland Soils? An Experimental Approach.” Soil Science Society of America Journal 82:283. https://doi.org/10.2136/sssaj2017.05.0162
Sun, Y., J. Masabni, and G. Niu. 2015. “Simulated Seawater Flooding Reduces the Growth of Ten Vegetables.” HortScience 50:694–698.
Thani, A. W., B. Schaffer, G. Liu, A. I. Vargas, and J. H. Crane. 2016. “Chemical Oxygen Fertilization Reduces Stress and Increases Recovery and Survival of Flooded Papaya (Carica papaya L.) Plants.” Scientia Horticulturae 202:173–181.
Thani, Q. A., A. I. Vargas, B. Schaffer, G. Liu, and J. H. Crane. 2017. “Physiological and Growth Responses of ‘Red Lady’ Papaya to Flooding and Solid Oxygen Fertilization.” Proceedings of the Florida State Horticultural Society 129:27–34.
USGS. 2021. “Water Level and Salinity Analysis Mapper.” Accessed September 15, 2021. https://fl.water.usgs.gov/mapper/