Introduction
In most US milk marketing orders, milk pricing is based on a multiple component formula that takes into account milk fat, true protein, and associated premiums for somatic cell count and microbiological quality. In the Southeast, milk is priced based on fat and skim milk value, with additional bonuses for somatic cell and bacterial counts. In recent years, butterfat has become more valuable; in many instances, the fat content in milk may represent more than half of the price paid for milk to the producer (USDA, Agriculture Marketing Service, Dairy Programs 2018). Numerous animal, dietary, and environmental factors influence milk fat content and yield in dairy cows. Because of the current pricing system, it is critical to reevaluate some of these factors and consider the economic implications of manipulating milk fat content with the ultimate goal of increasing milk fat yield in a profitable manner. This document discusses some of the history behind the changes in milk fat consumption, management factors that influence milk fat content and yield by dairy cattle, and economic implications of changing fat content through the diet in two case scenarios.
Paradigm Shift
In June 2014, Time magazine's cover page read, "Eat Butter. Scientists labeled fat the enemy. Why they were wrong." The history of margarine and butter consumption in the United States is a peculiar and cyclic one. Starting in the mid- to late 1930s, per capita butter consumption began to decline at the same time that the vegetable oil industry accelerated production and marketing of margarine. The reduced price for margarine, abundant supply of vegetable oils, expanding oil seed production, and need for protein meals for animal diets favored the adoption of margarine as a staple in US households and diets. Concurrently, a wave of research mistakenly suggested that animal fats were associated with increased risk of cardiovascular disease, whereas vegetable oils were expected to reduce the risk of cardiovascular disease. Government guidelines suggested reducing fat intake and replacing fat calories with calories from other nutrients (USDA HHS 1980). From the early 1940s to its peak in the early 1970s, US per capita consumption of margarine increased from 2.5 lb/year to 12 lb/year, while that of butter plummeted from more than 16 lb/year to only 4 lb/year (Figure 1). We have learned that hydrogenated oils present in margarine contain undesirable trans fatty acids that increase the melting point of the fat but are associated with increased risk of chronic diseases in humans, particularly coronary heart disease. On the other hand, research has demonstrated that saturated fatty acids present in dairy products are not linked to cardiovascular disease or metabolic syndrome (Drehmer et al. 2016; Otto et al. 2018). Extensive reviews of the literature have shown that dairy foods have repeatedly been found to have either no effect or a beneficial effect on cardiovascular disease despite their high content of saturated fatty acids. The recent Cardiovascular Health Study, a population-based prospective observational study, investigated risk factors for coronary heart disease in adult individuals of at least 65 years of age in the US (Otto et al. 2018). The investigators found no link between the consumption of whole-fat dairy products and cardiovascular disease. They found no association between fatty acids found primarily in milk fat (pentadecanoic, heptadecanoic, and trans-palmitoleic acids) and coronary heart disease, but showed that high blood concentrations of heptadecanoic acid, also known as margaric acid, were inversely associated with cardiovascular disease and stroke mortality (i.e., increased concentration of margaric acid in blood resulted in reduced risk of cardiovascular disease). These results suggest some potential benefits of this particular fatty acid to human cardiovascular health. It is important to note that dairy fats contain a small amount of naturally synthesized trans fatty acids (Lock and Bauman 2004). These ruminant-derived trans fatty acids have powerful anti-diabetogenic and anti-carcinogenic effects (Parodi 1999), as opposed to hydrogenated vegetable oils that contain unhealthy trans fatty acids.
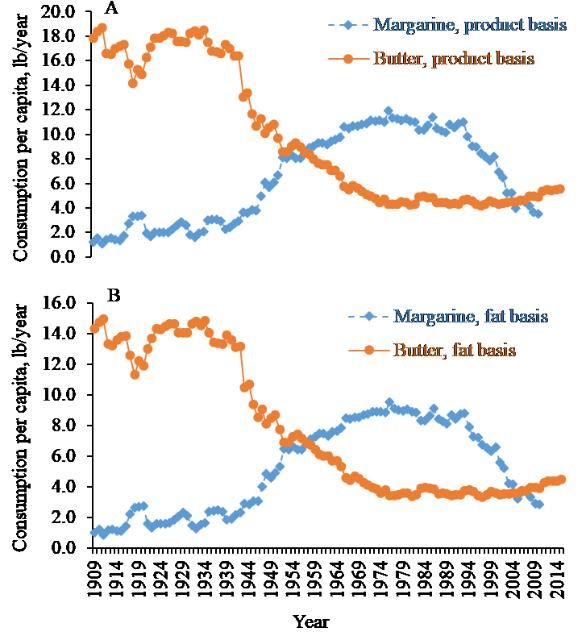
Credit: United States Department of Agriculture, Economic Research Service
Synthesis of Milk Fat by the Mammary Gland
Fatty acids are the main building blocks of milk fat. The mammary gland of a dairy cow synthesizes milk fat by two pathways. One is de novo synthesis of fatty acid, in which the mammary cells use 2- to 4-carbon fatty acids present in blood originated from ruminal digestion of carbohydrates and combine them to make fatty acids with up to 16 carbons. The second pathway is a process called incorporation of preformed fatty acids, which involves transferring fatty acids with 16 or more carbons from blood into the mammary cells and then packaging them to be secreted into milk. Thus, fatty acids with 16 carbons can be derived from de novo synthesis or incorporation of preformed fatty acids from blood. In a well-fed cow past the first month of lactation, approximately 25 to 30% of the fatty acids will be formed solely through de novo synthesis (up to 14 carbons); 30% will be fatty acids with 16 carbons (mixed origin). Approximately 40% will be strictly preformed fatty acids containing primarily 18 carbons (Palmquist et al. 1993). When cows lose large amounts of body weight, which is common in early lactation or during disease, the concentration of fat in milk increases because of incorporation of more preformed fatty acids (fatty acids with more than 16 carbons) that are in circulation from body fat loss. On the other hand, when cows undergo diet-induced milk fat depression, the concentration and yield of fat in milk decrease because the mammary gland is less capable of producing fatty acids through de novo synthesis (fatty acids with fewer than 16 carbons).
Factors Affecting Milk Fat
Genetics
Breed has a major impact on milk fat synthesis. Jersey cows synthesize milk with an average of 5.0% milk fat, whereas Holstein cows typically average 3.70% milk fat. Nevertheless, a large genetic variability exists within breed, and milk fat content (%) is one of the traits that is controlled by a few sets of genes. Because of this, heritability of milk fat content is usually high, approximately 0.32 for Holsteins (Soyeurt et al. 2007). Milk fat yield (lb) also has a relatively high heritability; for Holsteins, the Animal Improvement Program of the USDA considers 0.20 (https://www.aipl.arsusda.gov/reference/nmcalc-2017.htm). Heritability is the estimate of variation in the phenotype in a population that is caused by the genetic variation between individuals in the same population. High heritability usually means high probability of genetic gain from one generation to the next if selection emphasizes that particular trait. In other words, if producers select sires that have high predicted transmitting ability (PTA) for milk fat yield, the genetic gain by the next generation will be appreciable. Producers in milk markets such as those in the Southeast, in which milk volume still has a value and no penalty other than hauling cost applies, should favor selection of increased milk fat yield rather than concentration.
Environment
Environmental factors have marked effects on milk fat content and yield in dairy cows. In particular, high ambient temperature associated with high relative humidity causes dairy cows to suffer from heat stress, which leads to increased body temperature. When ambient temperature remains below approximately 75oF, cows are able to thermoregulate. They dissipate heat by radiation, conduction, convection, and evaporation. As the ambient temperature rises above that threshold, the differential between the cow's skin temperature and air temperature becomes smaller, which makes it difficult to lose heat by conduction and convection. In such scenarios, the cow becomes more dependent on evaporation. However, areas with high humidity such as the southeastern United States limit evaporation and reduce the cow's ability to maintain body temperature. Hyperthermia can occur as a result.
A clear seasonality in milk fat content is present in the US, especially in the Florida milk market (Figure 2; Florida milk market data obtained from the Agricultural Marketing Service agency of the US Department of Agriculture, Washington, DC). Concentration of fat in milk is typically greatest during January and February and smallest in July and August. This seasonality is in part mediated by heat stress in the summer months and it is present in all milk market regions in the US (Salfer et al. 2019). The exact mechanisms by which heat stress reduces milk fat synthesis are not completely understood, but they are likely related to changes in ruminal metabolism with reduced rumen pH that favors accumulation of products that inhibit synthesis of fatty acids by the mammary gland. Cows in hyperthermia have reduced rumen motility and increased panting, which is part of an attempt to better thermoregulate. Panting results in hyperventilation and loss of CO2, which is thought to reduce the flow of salivary buffers to the rumen. The combined effect likely explains the reduced rumen pH observed in cows with heat stress. It is also possible that elevated body temperature negatively affects the ability of mammary cells to synthesize milk components, including milk fatty acids. Therefore, dairy producers who want to produce more milk as well as milk with increased components such as fat must make an effort to minimize heat stress in dairy cows.
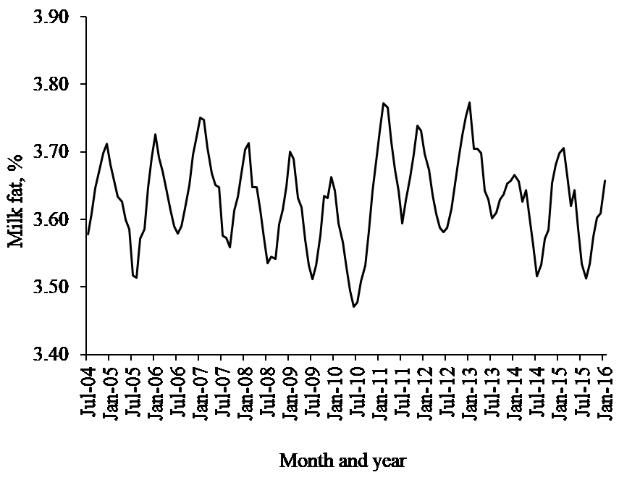
Credit: USDA Agricultural Marketing Service
Stage of Lactation
Cows in the first two to six weeks of lactation usually have elevated milk fat content because of the typical loss of body weight that occurs with the onset of lactation. This elevation can be extended if a cow develops diseases in early lactation. The typical lactation curve shows an inverse relationship between milk yield and milk fat content (Figure 3). In very early lactation, production of milk is low at the same time that the concentration of fat is high. As cows pass peak production, milk yield steadily declines while concentration of fat in milk increases.
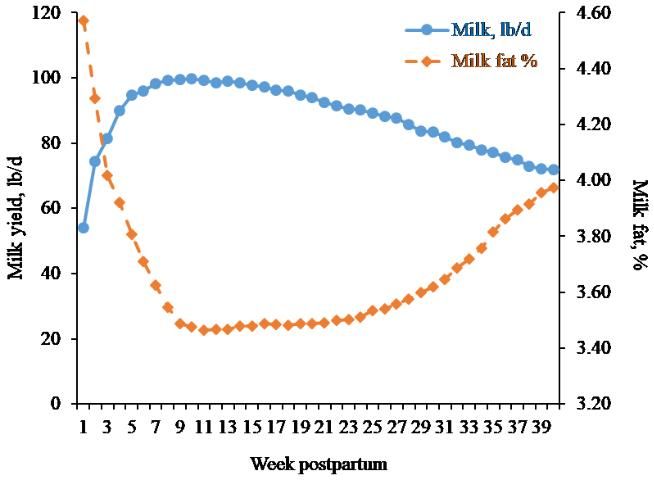
Credit: UF/IFAS
Nutrition and Nutritional Management
Dietary formulation and feeding management can greatly influence milk composition, particularly milk fat content, which can ultimately affect milk fat yield. Keep in mind that if a dietary change increases the concentration of fat in milk but results in less milk yield, then the total yield of fat might not increase at the same time that milk volume suffers. Because diet changes on farms are made without a control group, producers and nutritionists have to rely on comparing results before and after the change. In general, bulk tank values are more sensitive to changes in milk composition than to changes in yield per cow. For instance, producers are more likely to perceive a change in milk fat content than they are to appreciate a change in daily fat yield per cow. Results for fat content in milk are generated for each truckload of milk sold, but fat yield per cow is not available unless producers calculate those values. For instance, it is common for milk yield in bulk tanks to vary 3 to 4 lb/day, which might represent 3 to 5% of the daily yield of milk. Such fluctuations in milk yield are expected, particularly in herds that use bovine somatotropin or have a less regular feeding program or milking procedures. These fluctuations will influence the yield of fat regardless of the content of milk fat. Nevertheless, concentration of fat in bulk tank milk tends to be more stable and fluctuations are gradual, allowing producers to easily identify marked changes.
Diet-induced milk fat depression is characterized by a sudden drop in milk fat content that occurs after a change in diet and results in reduced milk fat yield. Small day-to-day variation in content and yield of milk fat is expected within cows or within a herd, but such daily variability is only between 0.05 and 0.10 percentage units of fat content. When cows experience diet-induced milk fat depression, the changes in milk fat content are usually greater than 0.20 percentage units in milk fat (e.g., from 3.70% to less than 3.50%), resulting in appreciable loss of milk fat yield. Altered ruminal fermentation with production of specific trans fatty acids that suppress de novo synthesis of fatty acids acts as the mechanism by which diet can induce milk fat depression. In most forages and diets, 50 to 60% of all fatty acids consumed by cows are polyunsaturated, meaning that they have two or more double bonds between carbons. Microbes in the rumen have the ability to add hydrogen ions to carbons when they are chemically linked by a double bond in an unsaturated fatty acid. Small quantities of trans fatty acids are produced during the hydrogenation of unsaturated fatty acids by rumen microbes. These trans fatty acids can leave the rumen and be absorbed into the cow's bloodstream. Some specific trans fatty acids isomers have the ability to inhibit de novo fat synthesis in the mammary gland. Therefore, when diets favor the accumulation of these specific trans fatty acids, milk fat content typically decreases, which can lead to reduced fat yield.
Among the most common dietary factors that increase the risk of diet-induced milk fat depression are:
- diets with low forage content (less than 40% forage);
- diets with low forage fiber content (less than 18% neutral detergent fiber from forage);
- diets with high content of highly fermentable carbohydrates (more than 40% nonfibrous carbohydrates);
- diets with high starch content from extensively processed sources (more than 28% starch);
- diets whose primary forage is corn silage with small particle size;
- no supplemental dietary buffers;
- diets with high ionophore content (more than 16 mg of monensin/kg diet);
- diets low in degradable protein;
- diets with large concentrations of polyunsaturated fatty acids (more than 2%);
- diets with supplemented fatty acids from marine or fish products (more than 0.4%);
- slug feeding;
- overmixing of diets resulting in forages with very small particle size; and
- inadequate mixing of forages with concentrates resulting in selective sorting by cows.
Values within parentheses represent threshold values for complete diets on a dry matter basis that might induce milk fat depression.
To increase milk fat yield, you must first minimize the dietary and management factors that increase the risk of milk fat depression. Once that is accomplished, focus on two important components: the dietary forage and the supplemental fats.
Make sure that diets contain enough forage fiber to maintain rumen health. Ruminal acidosis favors accumulation of trans fatty acids in the rumen which suppress milk fat synthesis. One of the risk factors for rumen acidosis is inadequate dietary forage, specifically neutral detergent fiber (NDF) from forage. For diets to minimize the risk of rumen disturbances, the concentration of forage NDF and the particle size of the forage are important. Forages stimulate rumen contractions, which are critical for absorption of the volatile fatty acids produced during digestion of carbohydrates and proteins in the rumen. Forages are also needed to stimulate rumination, which results in continuous chewing of feed particles that favors copious secretion of saliva. The increased amount of saliva buffers the acids produced during digestion in the rumen. Complete diets for lactating cows typically contain 40 to 60% forage and 18 to 24% NDF from forage on a dry matter basis. In general, the greater the forage and the forage NDF fed to cows, the greater the concentration of fat in milk. However, if forage intake limits total dry matter or energy intake, then yield of milk and milk protein can decrease. It is important to pay attention to forage quality. Better quality forages allow for increased inclusion in the diet, which increases total forage intake without compromising energy intake, and often benefits yields of milk and milk fat. Use the Penn State Particle Size Separator to monitor the particle size of forages and total mixed rations. Information on how to use the particle size separator and specific recommendations for minimum particle size are available at https://extension.psu.edu/penn-state-particle-separator.
Dietary fats can increase, decrease, or have no effect on milk fat content. The variable impact of dietary fat on milk fat content and yield is largely affected by the type of fat fed. In most cases, supplementing diets with fat will increase milk fat yield because of the stimulatory effect on milk yield, but it can also increase milk fat content. When diets of dairy cows are supplemented with 1.0 to 1.5% of the dry matter with dietary fatty acids, the expected response in milk yield is typically an increase of 3 to 4 lb/day. In addition, dietary fatty acids also can increase milk fat content. When the goal of supplementing fat is to increase milk fat yield, then the profile of fatty acids of the supplemental fat source is important. In general, fat sources rich in unsaturated fatty acids increase milk yield but tend to reduce milk fat content, which results in minor effects on milk fat yield. On the other hand, fat sources rich in palmitic acid (fatty acid with 16 carbons, also called C16) stimulate yields of fat and fat-corrected milk (milk yield adjusted with a constant fat concentration). Research has shown that supplementing diets of dairy cows with 1.0 to 1.5% of the diet dry matter with fatty acids from sources rich in palmitic acid can increase milk fat yield by as much as 0.4 to 0.5 lb/day (de Souza and Lock 2018), depending on the level of production of the herd.
Economics of Manipulating Milk Fat Through the Diet
Let us look at the economics of two hypothetical cases that affect the content of fat in milk. Case 1 is a dairy feeding a high-forage diet and producing 80 lb of milk with 3.80% milk fat. The producer and the nutritionist decided to change the diet in an attempt to increase volume, but they believed milk fat content would drop a little. The expectation was that the increase in the volume of milk would make up for the loss in butterfat percentage. They agreed to replace a portion of the corn silage in the diet (4 lb of dry matter) with corn grain (4 lb of dry matter) to increase the energy content of the ration so cows would make more milk. Feed intake was assumed to remain the same at 50 lb of dry matter per cow per day. Daily feed cost of the old diet was $5.52 per cow per day; with the new diet, it was $5.66. Milk was priced at $8.504 per 100 lb (cwt) skim milk and $2.569 per lb of butterfat. A premium of $0.65 per cwt of milk was provided based on somatic cell count and bacteriology, both of which were assumed to remain constant with the dietary manipulation imposed.
After the change in diet, milk increased from 80 lb to 84 lb, and milk fat decreased from 3.80% to 3.50%. The 3.5% fat-corrected milk yield remained the same at 84 lb. Was this dietary change a good decision? The milk price received depended on the concentration of fat, as shown in Figure 4. With the old diet, the milk price was $18.59 per cwt, or $14.87 for a cow making 80 lb. With the new diet, the milk price decreased to $17.85 per cwt, or $14.99 for a cow making 84 lb. Income minus feed cost with the old diet was $14.87 - $5.52 = $9.35 per cow per day. With the new diet, this was $14.99 - $5.66 = $9.33 per cow per day. This amounted to a loss of $0.02 per cow per day with the new diet. In this case, the change in diet was not a good decision.
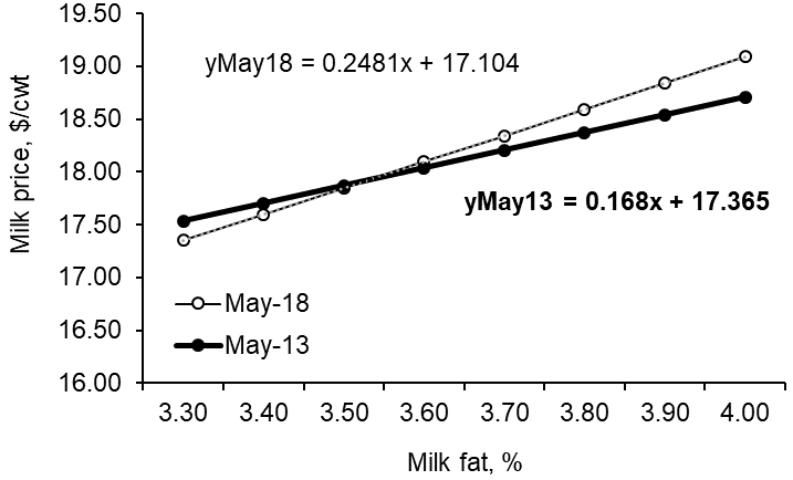
Credit: UF/IFAS
In 2013, butterfat was worth less compared to butterfat today (Figure 4). If we used the 2013 fat and skim milk prices, then the new diet would have a positive income minus feed cost of +$0.17 per cow per day.
Case 2 is a hypothetical dairy feeding a high-forage diet and producing 80 lb of milk with 3.65% fat. The producer and nutritionist decided to add 1.5% supplemental fat to the diet in an attempt to increase the energy density and stimulate more milk and fat yield. The nutritionist replaced 1.5% corn grain with a 1.5% high-palmitic acid fat product on a dry matter basis. This fat product was expensive, valued at $1,450 per ton, so the producer wondered if the dairy could afford this expensive ingredient. The dry matter intake was 52 lb per cow per day. They assumed it would not change.
After the change in the diet, milk yield increased from 80 to 83 lb, and milk fat increased from 3.65% to 3.80%. Dry matter intake did not change. Daily feed cost was $5.608 per cow per day with the old diet and $6.137 with the new diet; therefore, feed cost increased by $0.71 per cow per day. The value of more milk and increased fat compensated for this increase in feed cost. With the old diet, the milk price per cwt was $18.22, and at 80 lb, milk sales were $14.58 per cow per day. Income minus feed cost was $8.97 with the old diet. With the new diet, milk yield, butterfat, and the milk price per cwt increased. Milk price increased to $18.59, which resulted in milk sales of $15.43 per cow per day. With the $6.317 daily feed cost, income minus feed cost was $9.11. This was an increase of $0.14 per cow per day compared to the old diet. Adding the supplemental fat was a good decision in this case.
Increased milk volume means that more milk needs to be shipped. Notice that we did not include an adjustment for hauling cost. If milk increases from 80 to 83 lb, and hauling costs are $1.00 per cwt, that would add a cost of $0.03 per cow per day. This would become evident by more loads of milk that need to be shipped in a month with increased milk yield. Including hauling costs in the calculations implies that increasing butterfat content is worth even more compared with increasing milk volume.
Fat in milk is worth a lot today. With the milk prices as low as they are, it is even more important to consider the fat content in your milk. Check with your nutritionist to see if you have the right fat content and milk volume.
Conclusion
Milk fat has become valuable in the US dairy market, representing as much as or more than 50% of the price of milk paid to producers in recent years. The concentration of fat in the milk of dairy cows is variable and influenced by numerous factors, such as genetics of the cow, environment, and diet. Producers should consider selecting sires with superior breeding value for milk fat yield as part of their genetic improvement program. Minimizing heat stress, by implementing heat abatement strategies, benefits yields of milk and milk fat. Work with the nutritionist of the herd to formulate diets that minimize the risk of milk fat depression and consider dietary strategies that enhance milk fat yield. Consider the economics of dietary interventions to improve milk fat yield because, in many cases, the additional income is expected to offset the investment in supplements or better dietary ingredients.
References
Drehmer, M., M. A. Pereira, M. I. Schmidt, S. Alvim, P. A. Lotufo, V. C. Luft, and B. B. Duncan. 2016. "Total and full-fat, but not low-fat, dairy product intakes are inversely associated with metabolic syndrome in adults." J. Nutr. 146: 81–89. doi: 10.3945/jn.115.220699.
Lock, A. L. and D. E. Bauman. 2004. "Modifying milk fat composition of dairy cows to enhance fatty acids beneficial to human health." Lipids 39: 1197–1206. doi.org/10.1007/s11745-004-1348-6
Otto, M. C. O., N. L. Rozenn, X. Song, I. B. King, D. S. Siscovick, and D. Mozaffarian. 2018. "Serial measures of circulating biomarkers of dairy fat and total and cause-specific mortality in older adults: the Cardiovascular Health Study." Am. J. Clin. Nutr. 108: 1–9. doi/10.1093/ajcn/nqy117/5052139
Palmquist, D. L., A. D. Beaulieu, and D. M. Barbano. 1993. "Feed and animal factors influencing milk fat composition." J. Dairy Sci. 76: 1753–1771. doi: 10.3168/jds.S0022-0302(93)77508-6
Parodi, P. W. 1999. "Conjugated linoleic acid and other anticarcinogenic agents of bovine milk fat." J. Dairy Sci. 82: 1339–1349. doi: 10.3168/jds.S0022-0302(99)75358-0.
Salfer, I. J., C. D. Dechow, and K. J. Harvatine. 2019. "Annual rhythms of milk and milk fat and protein production in dairy cattle in the United States." J. Dairy Sci. accepted in press doi: 10.3168/jds.2018-15040.
Soyeurt, H., A. Gillon, S. Vanderick, P. Mayeres, C. Bertozzi, and N. Gengler. 2007. "Estimation of heritability and genetic correlations for the major fatty acids in bovine milk." J. Dairy Sci. 90: 4435–4442. doi: 10.3168/jds.2007-0054
de Souza, J., and A. L. Lock. 2018. "Long-term palmitic acid supplementation interacts with parity in lactating dairy cows: Production responses, nutrient digestibility, and energy partitioning." J. Dairy Sci. 101: 3044–3056. doi: 10.3168/jds.2017-13946.
United States Department of Agriculture, Economic Research Service. n.d. Food Availability (Per Capita) Data System. Accessed on July 15, 2018. https://data.nal.usda.gov/dataset/food-availability-capita-data-system/resource/6195a96b-c4c6-4593-b18e-0a5d6869601f
United States Department of Agriculture, Agriculture Marketing Service Dairy Program. 2018. Florida and Southeast Marketing Areas: Federal Milk Marketing Orders 6 and 7. Accessed on July 15, 2018. http://www.fmmatlanta.com/PriceAnnouncements/currentprice.pdf
USDA HHS. 1980. Nutrition and Your Health: Dietary Guidelines for Americans. Health.gov.