The purpose of this publication is to review and summarize the historical Florida research literature on nitrogen (N), phosphorus (P), and potassium (K) fertilization of potato. The research has provided the scientific basis for the Extension standardized fertilization recommendations that further form the basis for potato best management practices (BMPs). This publication is an updated version of a previous research literature review (Hochmuth and Cordasco, 2000). This updated review includes reports of research conducted since 1996 and expands on the role of irrigation in managing fertilizer for crop nutrient efficiency and environmental protection. The audience for this publication includes educators, such as Extension specialists and locla UF/IFAS Extension faculty members, private consultants, commercial potato producers, and governmental agencies involved in environmental regulations.
Potato is an important crop in Florida's agricultural economy. Potato production in Florida in 2007 represented 9.8% of the $1.65 billion state vegetable industry (http://www.florida-agriculture.com/fass_activities.htm). Eighty percent of potatoes grown in the state are harvested between May and June during what is considered the spring growing season. Of this amount, 85% are harvested from the Hastings or "Tri-county" area in northeastern Florida, including Flagler, Putnam, and St. Johns Counties, and the remaining potatoes are harvested from other areas across northern Florida, such as Suwannee County. Potatoes, are harvested in January through April from the state's winter planting areas in southeast (Dade County), southwest (Collier, Manatee, and Lee Counties), and also Okeechobee and Brevard Counties, Florida.
Potatoes are grown on sandy soils in most production areas, and fertilizer is required for economical crop production. The current nutrient target recommendations for potatoes are 200, 120, and 140 lb/acre N, P2O5, and K2O (Hochmuth and Hanlon, 2000; Hutchinson et al., 2008). The research prior to the late 1990s was summarized by Hochmuth and Cordasco (2000). Before that time the potato nitrogen (N) fertilizer recommendation was 175 lb/acre N (Stall and Sherman, 1984), with additional N from supplemental applications following leaching rain. Following the research review in 2000, the target N fertilizer recommendation was increased from 175 to 200 lb/acre. The studies through the late 1990s suggested 200 lb/acre of N as part of BMP for potato (Hochmuth and Cordasco, 2000). The potassium recommendation was uncoupled from the Mehlich-1 soil test because soil testing research failed to accurately and consistently predict crop response to K fertilization on the sandy soils. In other words, the research showed that accurate predictions of K needs were not consistent enough to warrant a recommendation to use a soil test for K fertilizer recommendations (Hochmuth et al., 1993a; 1993b). An "across-the-board" recommendation of 140 lb/acre K2O was instituted for any soil-test result. The recommended rate of applied P for soils testing low in Mehlich-1 P is 120 lb/acre P2O5. This amount is reduced to 60 lb/acre P2O5 when potato is grown on soils having medium extractable soil-test values of this nutrient, and 0 lb/acre when high or very high Mehlich-1 soil P values are present. Potato growers' fertilization rate practices in the late 1980s were about 270-100-270 lb/acre (N-P2O5-K2O) (Hochmuth et al., 1993a; 1993b; Kidder et al., 1992; Weingartner et al., 1999). A recent reference to "average grower N practices" by Simonne and Hutchinson (2005) indicated that grower N rates remained about the same in the mid 2000s.
The early research focused largely on potato agronomic yield and tuber quality (yield grade and tuber specific gravity) in response to fertilization. These attributes were important criteria for potatoes destined for the processing (chipping) markets. Since the early research was conducted and the first literature review, there have been increasingly strong interests in the incorporation of environmental impacts (water quality) into the research. In the Tri-County Agricultural Area (Livingston-Way, 2000), and for the state (FDACS, 2005), there has been more formalizing of BMPs and more interest in encouraging growers to implement BMPs. The definition of a BMP includes consideration of economics and environmental components, and that a BMP should embody the best available science. The establishment of a Total Maximum Daily Load (TMDL) (Magley and Joyner, 2008) and a basin management action plan (BMAP) (FDEP, 2009) for the Lower St. Johns River will mean continued efforts to encourage potato growers to adopt BMPs and to increase the effort on the part of IFAS to conduct needed education programs to improve the adoption and effectiveness of BMPs.
Potato fertilization research has been conducted in Florida for more than sixty years by numerous scientists. During this time, changes have occurred in potato production practices, including new cultivars, refinements in fertilizer application timing, use of fertilizer recommendations based on soil nutrient analysis, use of nematicides, and improved weed and pest management practices. The purpose of this publication is to review and summarize potato fertilization research in Florida, including new research since the late 1990s when the last summary (Hochmuth and Cordasco, 2000) was written. This research summary should form the basis for analyses of UF/IFAS Extension recommendations for potato fertilization. Almost all potato research has been conducted using subsurface irrigation, but this summary will identify those studies conducted with other irrigation methods, such as sprinkler irrigation.
Data Summary Method
Evaluation of potato yield responses to varying rates of applied fertilizer required a standardized method of summarizing statewide yields, which were expressed variably as kg/ha, Mg/ha, tons per acre, or cwt/acre. In addition, potato yields varied depending on season, weather conditions, cultivar, and location in the state. Relative yield (RY), a calculated percentage, was chosen as the unit to express potato yield responses to fertilization. Relative yield is an accepted scientific method for summarizing and presenting data across wide sources and reports (Brown, 1987).
In his book, C. A. Black (1992) summarizes the advantages and disadvantages of the RY approach. There are valid statistical limitations about RY, but he concludes the RY approach can be useful in displaying general relationships when applied properly and cautiously. Black demonstrates examples in his book where RY yield is helpful and where it was not. We chose the RY approach because we wanted to display the historical data without making biased decisions about what to include in the presentation. Plotting absolute yield data in original units across many studies obviously would result in a scatter of data rendering any general interpretation impossible. Black (1992) points out that decisions can always be improved with further research, but the data on hand are the best we have at the time. The highest yield for each fertilizer experiment was assigned a 100% value, and other yields were expressed as a percentage of the highest yield. The actual yield corresponding to 100% RY was presented as the number of cwt/acre. The RYs were plotted against rates of nutrient to determine how potato yields responded to fertilizer in Florida. The RY presentation allowed data from a variety of experiments to be included in the graphical summary of yield responses. For most studies, actual yields corresponding to RYs from 90 to 100% were not significantly different. The usefulness of the RY approach is further illustrated with potato data later in this review paper near the end of the section on N fertilization.
Sometimes the argument is made that growers have expectations of greater yields than those obtained in research projects or that yields in research plots are not as great as expected commercial yields. It is interesting to note in this regard there are reports of research yields in excess of 350 cwt/acre even as far back as 1955 (Mccubbin, 1956; Myhre et al., 1955). Realistic, regularly obtainable yields are more reliable than expected yields or "yield goals." Research on this subject (using corn) has documented that 20% of growers actually reached their yield goals and only 50% reached 80% of their yield goals (Schepers et al., 1986). Therefore, growers rarely achieve their stated yield goal, which means that fertilizer rates should not be set on unrealistic expectations, but rather on realistic documented goals based on research. This practice of using yield goals to set fertilizer rates is not recommended in Florida, so the effect of over-fertilization has been avoided by grounding expectations in measurement of observed yields. In effect, the current Florida fertilizer recommendation includes a component that addresses growers' concerns about yield expectation versus actual production capability. Throughout the years, scientists have conducted many replicated demonstration studies in growers' fields, with true commercial production practices, and those studies are included in this review. Excessive fertilizer was justified in times past when fertilizer was viewed as inexpensive insurance against yield loss, before potential impacts to the environment were clearly understood. Research with numerous crops, including potato, has shown that nutrient-use efficiency declines as nutrient rate, especially N, increases. Even given the best of production systems, N-use efficiency rarely exceeds 70% of the applied N. Concepts and practices for managing nutrients in vegetable production were summarized by Hochmuth (1992a, b; 2000). While fertilizer rate is reported in pounds per acre (lb/acre), the reader should keep in mind that the bed system used in each field may vary and require adjustments to the fertilizer rate. To address this issue, the linear bed foot concept can be used to convert among the various bed designs with and without spray rows or ditches (Hanlon and Hochmuth, 1989; Hochmuth and Hanlon, 2009). Additional cultural practices for commercial potato production can be found in Chapter 2 of the Vegetable Production Guide (Simonne and Hochmuth, 2010).
The fertilization recommendation addresses commercial yield and quality, the economics of crop production, and protection of the environment. Equally important is to have a mechanism available to the grower to adjust fertilization practices during the season due to leaching rains and extension of the growing season for additional harvests when market conditions are favorable. To address all of these concerns, UF/IFAS vegetable recommendations are given as a single target fertilizer rate that is projected to be sufficient for meeting the crop fertilizer needs for most growing seasons. This single target fertilizer rate is a recommended starting point and has been used historically in all vegetable fertilizer recommendations in Florida, as well as around the country. The target recommendation approach with footnotes was used by Montelaro (1978) and confirmed by Hochmuth and Hanlon (1995; 2000). The target value was derived from numerous fertilizer studies and represents a reasonable fertilizer rate that reflects the average maximum crop responses from all of the fertilizer research, not the extremes in responses. However, the recommendation process recognizes some growing seasons are different due to more leaching rains or increases in crop value that can lead to prolonged harvest windows. Hence, the target fertilizer amount is accompanied by a series of footnotes that explain the addition of supplemental fertilizer during the growing season, which includes addressing leaching rains and additional harvests near the end of the season. It is logical to select a single target rate based on research that avoids excessive fertilizer applications that often reduce crop nutrient efficiency and increase the potential for environmental degradation. Keeping nutrients in the soil profile and available for potato plant uptake requires proper irrigation management. Information is regularly updated because of the critical nature of irrigation and drainage management with respect to nutrient uptake by the plant and possible loss of nutrients to the environment (Simonne et al., 2010).
Another tool for measuring nutrients that contributes to appropriate management of fertilizer includes plant tissue and/or plant sap sampling. For interpretation of plant and petiole sap values, see Hochmuth (1994 a, b) and Hochmuth et al. (1991b; 2009).
Nitrogen Mixed-Fertilizer Trials
Mixed N-P-K fertilizers were used in potato trials from 1947 through 1967 to establish crop nutrient requirements and evaluate fertilizer application methods (Figure 1). Attributing crop response to a single nutrient, however, could not be done with certainty from a blended N-P-K material. Since N was likely the most limiting nutrient in sandy soils, yield responses in mixed-fertilizer studies are considered here as most likely responses to N and therefore yield is plotted against the N content in the fertilizer material. All trials discussed in this section were conducted in Hastings, Florida, unless otherwise indicated.
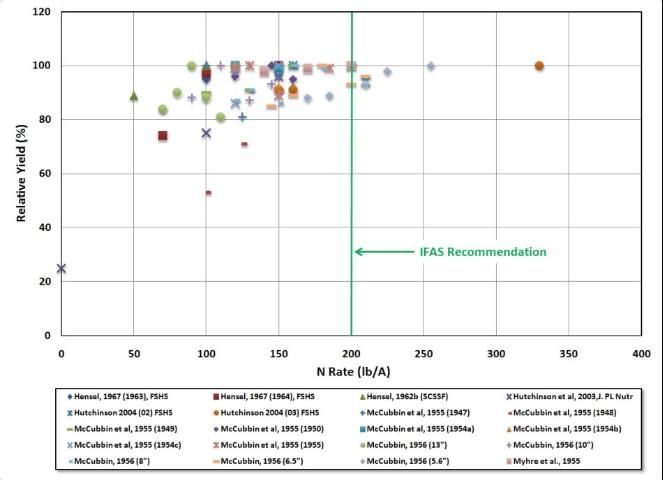
Side-dress applications of fertilizer from a 5-7-5, 5-7-6, or 6-8-8 (N-P2O5-K2O) fertilizer were used to evaluate the effects of N-P-K on potato yield in experiments from 1947 through 1955 (McCubbin et al., 1955). Fertilizer applied at planting was banded on either side of the plant row to supply 100 lb/acre N in 1947 and 1948, and 110 and 120 lb/acre N in 1949 and 1950, respectively. Because of a wet season in 1947, additional fertilizer was applied to the sides of the row 70 days after planting. Rainfall continued after the last fertilizer side-dressing. Side-dressing resulted in similar potato yields as potatoes that had received no side-dressed fertilizer. Average yield during the 1947 season was very low due to the weather conditions. In the other seasons, additional fertilizer was side-dressed before blossom time or 45 to 60 days from planting. Leaching rainfall occurred before fertilizer was side-dressed in 1948, and a significant yield response to side-dressed fertilizer resulted. Potatoes that received 100 lb/acre side-dressed N (200 lb/acre total N) from mixed fertilizer yielded 141 cwt/acre compared to 74 cwt/acre from potatoes that received no fertilizer side-dressing (100 lb/acre total N). Though rainfall was below normal in 1949 and 1950, rain occurred in sufficient amounts after fertilizer side-dressing for acceptable yields. Yield responses to high side-dressed N rates were minimal. Optimum yield occurred with 160 lb/acre total N (226 cwt/acre, 100% RY) in 1949, and with 145 lb/acre total N (152 cwt/acre) in 1950. Yields in all experimental seasons with higher N rates were not significantly different from yields with lower N rates.
Evaluations of yield responses to side-dressed fertilizer continued in 1954 and 1955, with side-dress mixed-fertilizer applications made in addition to a base (at planting) application of 120 lb/acre N. In 1954, 30 lb/acre N was side-dress applied in experiments on Leon, Bladen, and Rutledge fine sand soils. Yield on the nutrient-poor, Leon fine sand soil was greater with the 30 lb/acre side-dress application, 150 lb/acre total N (187 cwt/acre, 100% RY), than with the base fertilizer application only (86% RY). Similar yields resulted with or without the side-dress fertilizer application on the heavier Bladen and Rutledge fine sand soils. High yields occurred each year with the base-mixed-fertilizer application supplying 120 lb/acre N, resulting in 285 cwt/acre and 257 cwt/acre, respectively. Yields in 1955 on Bladen fine sand soils were also unaffected by additional side-dressed fertilizer. High yield resulted this season with 15 lb/acre of side-dressed N, 135 lb/acre total N (191 cwt/acre, 100% RY) and did not increase with 30 or 60 lb/acre side-dressed N to 180 lb/acre total N. Researchers concluded that side-dressed fertilizer had little yield effect on dark sand soils, but when applied to light sand soils, yields were significantly improved. The soil organic matter concentration, not measured in these experiments, was described as moderate for both the Bladen and Rutledge fine sand soils.
Soil moisture content, seeding rate, and fertilization were factors managed by growers to increase potato yields (Myhre et al., 1955). Researchers conducted a factorial experiment using sixteen soil moisture levels, four N rates, and two seeding rates on Bladen loamy fine sand soil, considered an "old" potato production soil (soil that had been in potato production for many years). Soil moisture concentrations were achieved by running water through every other furrow. The water height in the "wet" furrow was maintained at 4 inches below the bed top. All plants received 6N-8P2O5-8K2O as 100 lb/acre N at planting and an additional 40 lb/acre N, 40 days from planting. Total applied N treatments were 140, 170, 200, and 230 lb/acre from additional side-dress applications of 30 lb/acre N from NaNO3, 58 days after planting (treatment 2), 60 lb/acre N from 6N-8P2O5-8K2O, 18 days before planting (treatments 3 and 4), and 30 lb/acre N from NaNO3, 58 days after planting (treatment 4). The greatest yields occurred with the seeding rate of 2,450 lb/acre or seeds spaced 8 inches apart.
Soil moisture concentrations were 12, 10, and 8%, respectively considered wet, center-bed moist, and dry, as estimated on an oven-dry basis. The highest potato yields resulted from moist soil-water conditions, lower yields from dry field conditions, and lowest yields from wet soils where root knot nematodes and pink rot disease reduced yields. Total marketable yields were nearly double the local average yields of 160 cwt/acre in 1955. Marketable yields were similar with all N rates, with 100% RY resulting from 200 lb/acre N (307 cwt/acre). Marketable yields with 140, 175, and 235 lb/acre N were 98%, 99%, and 96% RY, respectively. Researchers noted no yield advantage from application of 60 lb/acre N three weeks before planting, compared to 0 preplant N, but suggested additional studies were needed to confirm the findings of this trial.
Experimentation to increase yield continued as researchers evaluated the profitability of potato yields from plants grown 13 inches apart to plants grown 10, 8, 6.5, or 5.6 inches apart (McCubbin, 1956). Experiments were conducted on leveled land with furrows for subsurface irrigation. Yield results were presented for each seeding rate. Fertilizer from 6N-8P2O5-8K2O was banded on each side of the plant row before planting to supply from 70 lb/acre to 255 lb/acre N, with the higher N rates applied with the closer seed spacing rates.
In 1943 to 1953, the optimum seeding rate for potato was determined to be 2,450 lb seed/acre or seeds spaced 8 inches apart in rows 40 inches apart. After leveling the field and improving the irrigation system, researchers in 1956 again found the 8-inch seed spacing to be the most profitable for potato production based on the net profit after sale of US 1A and US 1B grade potatoes. Optimum yields occurred with this seed spacing with 165 lb/acre N (355 cwt/acre, 100% RY). Yields of 383 cwt/acre resulted from potatoes spaced 5.6 inches apart and fertilized with 255 lb/acre N, but profit was reduced 6% by the higher costs associated with the closer seed spacing.
Attempts at finding material to enrich the organic matter composition of Florida sand soils were marginally successful with application of eight- to ten-year-old pine sawdust applied at 0, 5, 10, 15, or 25 tons/acre (Locascio et al., 1961). Experiments were conducted on Kanapaha fine sand soils near Gainesville. Applied sawdust was disked into the upper 6 inches of soil with N at 0, 50, or 100 lb/acre (from 6N-8P2O5-8K2O) applied broadcast. Additional fertilizer from 6N-8P2O5-6K2O was banded in the row at 120 lb/acre N in 1957 and at 180 lb/acre N in 1958. Yield responses were averaged over four rates of applied sawdust for each rate of applied N in potato experiments conducted in 1957 and 1958. Marketable US No. 1 and 2 potato yields were not affected in either season by the rate of applied N or by the rate of applied sawdust. High yields occurred with 120 lb/acre N (187 cwt/acre, 100% RY) and with 180 lb/acre N (178 cwt/acre, 100% RY), in 1957 and 1958, respectively. Soil organic matter, tested in 1958 with the Walkley-Black method, had not changed one year after application of 0 tons/acre of sawdust, 1.81% organic matter, compared with 25 tons/acre of sawdust, 1.85% organic matter.
Black polyethylene mulch had a positive effect on potato yield and quality (increased yield of size grade A potatoes) in 1961 and 1962 experiments (Hensel, 1962b), but had a negative effect on yield and potato quality (specific gravity) when the planting season was delayed one month to February 14 in a 1964 experiment (Hensel, 1967). All black polyethylene mulch treatments in 1965 resulted in higher yields than unmulched treatments with three planting dates, January 13, 27, or February 10, and two harvest dates, April or May (Hensel, 1968). As the planting date was delayed in 1965, yields of polyethylene mulched and unmulched plants increased. In 1966, only January-planted, polyethylene, or aluminum-foil mulched potatoes resulted in higher yield than unmulched potato plants. February-planted potatoes yielded similarly this season whether mulched or unmulched. The 1961 and 1962 experiments were conducted on Ona fine sand, while the remaining experiments were conducted on Rutledge fine sand.
No interaction occurred between mulch and fertilizer rates in 1962 through 1964. Yield responses to increasing N rates from 6N-8P2O5-8K2O fertilizer were averaged over mulched and unmulched treatments. In 1962, yield responses to N rates 55, 110, or 160 lb/acre were optimized with 110 lb/acre N (147 cwt/acre, 100% RY) (Hensel, 1962b). In 1963 and 1964 (Hensel, 1967), yields responded to N rates 75, 115, or 150 lb/acre and were optimized with 150 lb/acre N in both seasons (213 and 193 cwt/acre, respectively, 100% RYs).
Older research mostly with mixed N-P-K fertilizers supported the previous fertilizer recommendations (Montelaro, 1978) for irrigated potato production on mineral soils. These older recommendations were based on levels of mixed fertilizers, typically using 6-8-8 (N-P2O5-K2O) mixture. The recommendations were 150N-120P2O5-200K2O with 1 to 3 supplemental applications of 30-0-30 (lb/acre), depending on length and intensity of rainfall.
Nitrogen-Single Nutrient Experiments
Potatoes are produced in Florida with several irrigation methods, most with the subsurface (seepage) irrigation method. The early research largely conducted in the northeastern part of the state was done with the commonly used subsurface irrigation system. This system is used in southwest Florida but no research reports could be found that were conducted in that part of the state. However, potatoes in Dade County and some areas in northern Florida are grown with sprinkler systems. Nitrogen research results are presented by irrigation system, with sprinkler irrigation in Figure 2, subsurface irrigation in Figure 3, and combined in Figure 4. This separation was done to determine if there might be differences in fertilizer needs of crops grown with different irrigation systems. Based on limited data with sprinkler-irrigated potato presented below there appears to be no difference between potato nitrogen fertilizer needs based on irrigation system. This conclusion is logical since the goal of a fertilizer program is to fertilize the plant and should not be dependent on the irrigation method per se. If there were variations in fertilizer needs based on irrigation system, then there might be certain inefficiencies associated with a particular irrigation method or with the management of the system. Seepage irrigation can supply the entire crop water requirement similar to a sprinkler system; however, seepage is a relatively inefficient system and ~80% of the applied water is lost (Mylavarapu, et al. 2008 report to SJRWMD).
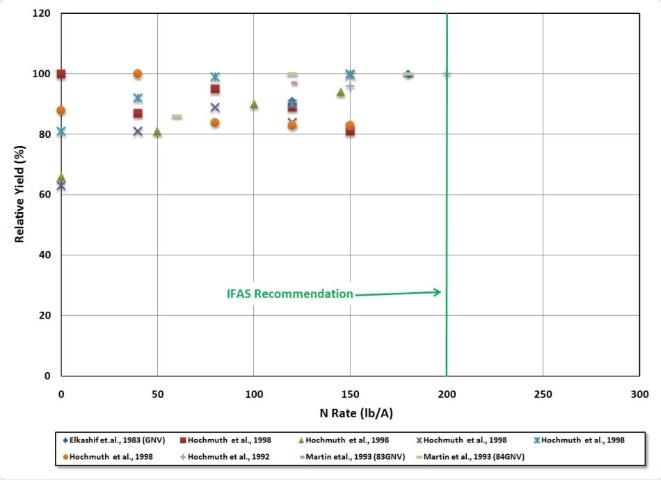
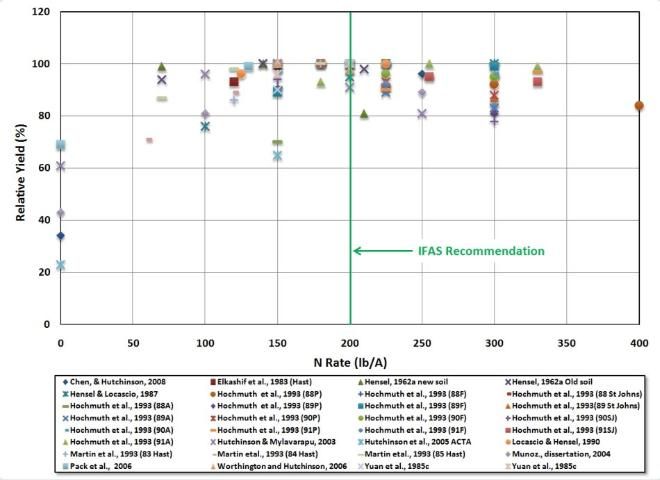
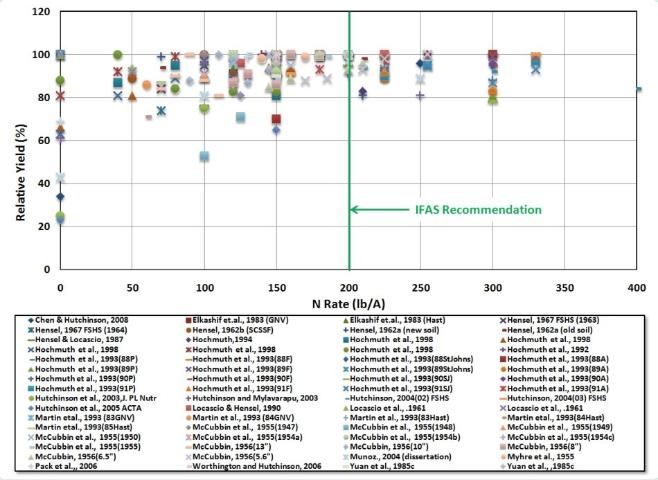
As of the late 1990s, fertilizer application recommendations for potato specified no fertilizer application before planting (Kidder et al., 1992). This recommendation was made to minimize the chance of N leaching or runoff before the plants emerged with a root system that could take up the fertilizer, and was supported by earlier research (Myhre et al., 1955). Nonetheless, growers typically do apply preplant fertilizer containing some N and P, and large amounts of K. Band-applied fertilizer is recommended by IFAS Extension at planting or at crop emergence for all applied P2O5, 70 lbs/acre K2O, and 60 to 90 lb/acre N with side-dress application of the remaining N and K fertilizers 35 to 40 days later.
Potato yield (sprinkler irrigation in Live Oak) was unaffected by waiting until plant emergence to make the first application of N (Hochmuth and Jones, 2004). This report is the latest research to support a recommendation of waiting until planting or plant emergence before making the first application of N. An earlier study (Myer et al., 1955) also showed that pre-plant fertilizer application did not result in an increase in yield. Split-application of N in this manner is a safeguard against leaching with subsurface or overhead irrigation for potato production. Further, waiting until the tubers (seed pieces) have sprouted to make the initial N application protects against the risk of leaching from early-season rainfall. A study in Hastings confirmed this hypothesis of increased N losses associated with pre-plant N applications. From the patterns of nitrate concentrations in the perched water table beneath a potato crop, it was possible to conclude that much of the leached N came from the pre-plant application of N to the crop (Munoz-Arboleda et al., 2008). The combined previous and recent research supports a recommendation of waiting until cracking (plant emergence) to make the first application of N fertilizer. This recommendation should have positive implications for water quality protection and potato economics while not reducing yield.
Comparisons of yield responses to factorial combinations of three each N and P rates were conducted on long-term potato ("old") soils and newly cleared soils in Hastings (Hensel, 1962a). Fertilizer, including 240 lb/acre K2O, was placed preplant in bands beneath and to the side of each plant row. On old cultivated soils, potato yields were not different with increasing N (70, 140, or 210 lb/acre N) optimizing with 140 lb/acre N (146 cwt/acre, 100% RY). On newly cultivated soils, potato yields responded quadratically to increasing N rates (70, 140, or 210 lb/acre). Researchers suspected a drier season limited yields with higher N rates when high yields resulted with 70 lb/acre N (178 cwt/acre, 97% RY).
Researchers conducted early trials in Gainesville and Hastings to study reducing fertilizer leaching losses through the use of controlled-release fertilizers (CRF)-N (Elkashif et al., 1983). Fertilizer treatments included 100% preplant applications of isobutylidene diurea (IBDU), sulfur-coated-urea (SCU), or NH4NO3, or split N applications, 67% of N applied preplant as IBDU (coarse or fine grain), or SCU followed by side-dress application of NH4NO3 four weeks after plant emergence. Preplant fertilizers were placed 4 inches below and 4 inches to each side of the seed tuber. Side-dress fertilizer applications were banded 9.8 inches from the plant. Seed tubers were planted in mid-February at each site, and the crops received above-average rainfall through March. Very dry weather in April and May resulted in the use of sprinkler irrigation in Gainesville (1.0 inch/week) and subsurface irrigation in Hastings (3 inches/week). Applied fertilizer rates were 100 or 180 lb/acre N, 230 lb/acre P2O5 from concentrated superphosphate (applied preplant), and 240 lb/acre K2O from K2SO4 (33% side-dressed).
In Gainesville, marketable yields were not significantly affected by N source (average yield, 237 cwt/acre) or by N rate (average yield, 237 cwt/acre). Marketable yields were 7% higher with split-N applications (average yield, 245 cwt/acre) than when all fertilizers were applied preplant (average yield, 230 cwt/acre). Researchers indicated that with overhead irrigation, split-applied N reduced fertilizer leaching losses and resulted in higher yields (Fig. 2, sprinkler irrigation). With subsurface irrigation in Hastings, however, marketable yields were similar with 100% preplant NH4NO3, or split-N applications of IBDU (coarse grain), IBDU (fine grain), or SCU with NH4NO3 side-dressed (average yield, 270 cwt/acre). Researchers cited better nutrient retention in the root zone with subsurface irrigation compared to overhead irrigation as the basis for the equal response to 100% preplant NH4NO3 and the split-N application method. Yields were lower with 100% preplant CRF-N sources IBDU (coarse) or SCU (average yield, 219 cwt/acre) due to reduced N release from these sources with cooler winter to spring soil temperatures. Significantly higher yields resulted at Hastings with 180 lb/acre N (266 cwt/acre, 100% RY) than with 120 lb/acre N (91% RY).
Measurable changes in leaf-tissue concentrations of N, P, and K occurred for leaf samples taken between four and eight weeks after plant emergence. Leaf tissue N, P, and K concentrations fell 31%, 40%, and 35%, respectively, during this period when nutrients are largely translocated from the leaves and stems to the developing tubers. Leaf-tissue concentrations of Mg and Ca increased by 50% at eight weeks after planting compared to the concentrations at four weeks.
The heat requirement of some CRF fertilizer sources for the release of nutrients can lead to reduced efficiency of these fertilizers for cool season crops, such as potato. In the Hastings experiment described above, split application of SCU or IBDU with NH4NO3 performed as well as a single preplant application of NH4NO3. Researchers working with a hydrophilic gelling agent incorporated into liquid urea-ammonium-nitrate (UAN) in the spring of 1990, hoped to develop an efficient, leach-resistant, single application N-fertilizer source that was not dependent upon heat for release (Locascio and Hensel, 1990). As with all CRF-N sources, the full benefits of these fertilizers were realized only during seasons of excessive rainfall. In this study, average to below average rainfall minimized the effect of the gelled fertilizer.
Two N rates, 125 and 225 lb/acre, were applied from four liquid N treatments, with or without the gelling agent. A fifth dry fertilizer treatment was included. Liquid urea-ammonium-nitrate (UAN) treatments included UAN combined with a non-ionic gel, with an anionic gel, with a cationic gel, and without a gel. A dry UAN fertilizer treatment was also applied. All treatments were applied after planting, February 7, 1990, using a liquid N-fertilizer applicator. The experiment was conducted near Hastings, Florida, on Ellzey fine sandy soil to which subsurface irrigation was applied at 3 inches/week.
An interaction occurred between gel treatment and fertilizer rate. Marketable yields were greater when potato plants were fertilized with liquid or dry UAN and liquid UAN with cationic gel or anionic gel than those fertilized with liquid UAN with nonionic gel. Marketable yields with all N treatments were similar with 225 lb/acre N and generally higher than with 125 lb/acre N, except with the liquid UAN where higher yield resulted with 125 lb/acre N (300 cwt/acre, 100% RY), and 90% RY with 225 lb/acre N.
Experiments were conducted in 1983 and 1984 on Ca and P rich Ellzey sands (Yuan et al., 1985c). Researchers planned to balance the high Ca and P concentrations in these soils and increase yields by raising the soil N and K fertility. Yield responses were evaluated for N rates of 150 or 200 lb/acre N (50 lb/acre side-dressed five weeks from planting). Three K rates, 0, 140, and 200 lb/acre K2O, were applied with each N rate at planting, and an additional 50 lb/acre K2O was side-dressed at five weeks. The effect of N rate on marketable yields (averaged over all K rates) was not significant in either year. Marketable yields with 200 lb/acre N each season were 202 and 194 cwt/acre (100% RYs) and with 150 lb/acre N were 90 and 96% RY each year. More N and K were removed from the soil with harvest than with any other nutrient. Nitrogen removed from the soil with the harvested potato was between 70 to 80 lb/acre and did not change between plants fertilized with 150 or 200 lb/acre N in either experiment year. Researchers concluded that application of N to 200 lb/acre provided no yield advantage compared with yields at 150 lb/acre N on soils high in Ca and P. Vegetative tissues sampled the day before harvest each year were within the sufficiency range of 2 to 3% N each year. As with yields for these experiment seasons, tissue N concentrations were similar with both N rates, averaging 2.8% in 1983 and 2.5% in 1984.
The N-fertilizer rate recommendation remained at 150 lb/acre (base application with supplemental N to replace leached N) through 1984. The N rate was updated to 175 lb/acre in 1984 (Stall and Sherman, 1984). In 1992, experiments were conducted in three counties in northeast Florida from 1988 to 1991 to field test IFAS Extension fertilizer recommendations (Hochmuth et al., 1993b). Marketable potato yield and potato quality were evaluated following application of 150 to 400 lb/acre N (NH4NO3) at experiment sites in Putnam, Flagler, and St. Johns Counties, and the University of Florida Agricultural Research and Education Center (AREC) in Hastings. Yields with P and K rates near the recommended amounts based on Mehlich-1 (pre-fertilization) soil testing (Kidder et al., 1989) were compared to commercial grower yields with grower N, P, and K rates, which were above the recommended rate at each location. In 1988, N and K fertilizers were banded at planting (near the tuber), two weeks after emergence, and 40 to 50 days after planting. In 1989, 1990, and 1991, N and K were applied once at planting and again 40 to 45 days after planting.
Yields of marketable (size A) potatoes were higher with the commercial fertilizer program than with the experimental fertilizer program in 1988 (1% probability). The authors attributed the lower yields to the second side-dressing of N, which was made too late in 1988 to benefit yields with the experimental program. The commercial fertilization program had a single side-dress N application. Highest yields among the experimental fertilizer treatments occurred with 200 lb/acre N in Putnam (156 cwt/acre, 100% RY), 225 lb/acre N in Flagler (218 cwt/acre, 99% RY), and 225 lb/acre N in St. Johns (293 cwt/acre, 100% RY). Yields increased quadratically with increasing N rates at the AREC location this season. Highest yields occurred with 300 lb/acre N (240 cwt/acre, 100% RY) and 92% RY with 225 lb/acre N. Yields were significantly higher (1% probability) with the commercial N-P-K fertilization program at each location with the exception of the AREC experiment where yield with the commercial rate was lower. Corresponding yields with commercial N rates from 212 to 360 lb/acre were 259, 267, 321, and 231 cwt/acre in Putnam, Flagler, St. Johns, and the AREC. Leaf-tissue N concentrations sampled at full bloom at Flagler and AREC were above adequate (> 4.0%) and not affected by increasing rates of applied N including the commercial N rate. Leaf samples taken at early bloom in St. Johns County were also above the sufficiency range (> 4.0%) with significantly higher N concentrations with the commercial fertilizer application.
A single side-dress fertilization at 40 to 45 days after planting was used in 1989-1991, compared to two side-dress fertilizer applications in 1988. Marketable size-A potato yields were higher in 1989 with 150 lb/acre N at Putnam County resulting in 262 cwt/acre (100% RY) compared to 157 cwt/acre yield with the commercial fertilizer N rate of 250 lb/acre (Hochmuth et al., 1993b). Leaf-tissue N concentration at full bloom was also significantly lower with the commercial fertilizer rate compared to experimental rates, though all tissue N concentrations were above adequate (> 4%). Yields decreased linearly with N rates from 150 to 300 lb/acre at St. Johns County and the AREC. Marketable yields were optimized at both locations with 150 lb/acre N (335 and 212 cwt/acre, respectively, 100% RY). Yields with the commercial rate were significantly lower, 260 and 189 cwt/acre with 240 and 228 lb/acre N at each St. Johns County and the AREC, respectively. Leaf-tissue N concentrations with the commercial N rate were significantly lower at St. Johns County and not different from N concentrations of plants fertilized with experimental rates at AREC. High yield occurred with 225 lb/acre N at Flagler County (238 cwt/acre, 100% RY), which was greater than 226 cwt/acre with the 215 lb/acre N commercial grower rate.
Marketable yields were similar with experimental and commercially applied N, P, and K rates in 1990 experiments at Putnam and St. Johns Counties (average yields, 205 and 85 cwt/acre, at each site). Marketable yields differed at 5% (Flagler) and 1% (AREC) probability with all applied fertilizer rates at these sites (Hochmuth et al., 1993b). Optimum, 100% RYs with experimental fertilizer rates resulted with 150 lb/acre N (Flagler, 302 cwt/acre) and with 225 lb/acre N (AREC, 227 cwt/acre). Yields fell with 300 lb/acre N to 95% and 96% RY at these sites, respectively. Leaf-tissue N concentrations measured at pre-, early-, or full-bloom were above the sufficiency ranges for all locations. Significant differences in leaf-tissue N concentrations between the experimental and commercial fertilization programs occurred at St. Johns County, though yields did not differ, and at the AREC where yields were different (5% probability) between fertilization programs.
In 1991, yields were lower overall and responded little to fertilization practices (Hochmuth et al., 1993b). Yields with some experimental rates were the same or higher than yields with the commercial grower fertilizer rates. From the four years of work, researchers concluded that 150 to 175 lb/acre N was sufficient for high potato yields and that N rates exceeding 250 lb/acre often reduced potato yields. Effective side-dress N application occurred between 35 and 40 days from planting. Band application of 60 to 90 lb/acre N was recommended at planting and at side-dress. These preliminary revised recommendations were tested against the commercial fertilization program in commercial field demonstration plots one acre and larger in 1992 and 1993 (Hochmuth et al., 1993). Use of the revised fertilization rates and timing resulted in yields equivalent to the commercially fertilized plots and reduced N use by 100 lb/acre. Application of less than the recommended N rate reduced yields in Flagler (1992) where 140 lb/acre N resulted in 374 cwt/acre compared to 403 cwt/acre with the commercial N rate of 210 lb/acre. Delaying the initial fertilization from at planting to four weeks after planting also reduced yields in St. Johns County (1992) to 238 cwt/acre compared to 323 cwt/acre with the commercial fertilization applied near planting and at two weeks after emergence (cracking). Nitrogen deficiency symptoms or smaller plant size resulted in St. Johns County (1993) when the side-dress fertilization was delayed to 56 days after planting. The specific gravity of harvested potatoes was unaffected by N rate in these studies.
Further on-farm demonstration studies were conducted in 1999 as a follow-up to the on-farm studies reported above (Weingartner et al., 1999). Four commercial farm sites were used in addition to one site at the University of Florida Hastings Research and Education Center. In these studies, comparisons were made between a large plot (several acres in size) of potato grown with the Extension-recommended N program and one grown with the grower program. Fertilizers were applied by the growers. Potato yield and tuber specific gravity were measured. In all cases except one, t-tests showed no difference between fertilizer treatments for yield or specific gravity. At one grower site there was a slight increase in yield (P=0.08) with the commercial fertilizer rate and a significant reduction (P=0.002) in tuber specific gravity with the grower fertilizer program. Higher specific gravity is desired, so increased fertilizer at this site increased yield slightly but reduced tuber quality greatly. Average grower N rates were 247 lb/acre N (ranged from 210 to 283 lb/acre N). The recommended rate used was 195 lb/acre N.
Overall, 22 on-farm demonstration trials were conducted during 1992-1999 (Weingartner et al., 1999). Average yield with the commercial grower fertilizer program was 335 cwt/acre, and the yield with the recommended N program was 323 cwt/acre. Specific gravity with the grower program was 1.077, and it was 1.079 with the recommended program.
There was a lack of yield response to nitrification inhibitors (dicynandiamide and nitrapyrin) in experiments from 1983 through 1985 (Martin et al., 1993). For the purposes of this fertilizer summary, yield responses were averaged for N rates from treatments. Experiments were conducted in Gainesville in 1983 and Hastings in 1983 and 1985. Nitrogen from NH4NO3 at rates of 120 or 180 lb/acre was applied with 25 lb/acre P2O5, and 200 lb/acre K2O in Gainesville and Hastings (1983). Fertilizers were mixed and applied in two bands at planting. Fertilizer bands were 1.5 inches deep and 1.5 inches to each side of the seed pieces. In Hastings (1985), N rates were 60, 120, and 180 lb/acre with 75% applied as NH4-N and 25% as NO3-N. Potassium from K2SO4 was applied equally at planting and two weeks after emergence. Subsurface irrigation was applied as needed on Ellzey sand soils in Hastings. In Gainesville, Millhopper fine sand soils received sprinkler irrigation. These soils had higher CEC, organic carbon, and N concentrations, and lower sand content than the Hastings soils.
Yields of marketable tubers, grades A and B, were not affected by N rates in Gainesville (1983). Similar yields resulted with 180 lb/acre N (245 cwt/acre, 100% RY) as with 120 lb/acre N (97% RY). In Hastings (1983), marketable yields increased from 120 lb/acre (67% RY) to 180 lb/acre (195 cwt/acre, 100% RY). Yields increased quadratically in 1985 (Hastings) through 120 lb/acre N (302 cwt/acre, 98% RY) and were 2% higher with 180 lb/acre N. Potato quality was determined by changes in the specific gravity of tubers as affected by varying rates of applied N. Tubers with high specific gravities, such as 1.091, as occurred in Gainesville, would yield nearly 15% more potato chips than those with specific gravities of 1.071 (1.078 occurred in Hastings, 1985). Rates of applied N had no effect on the specific gravity of tubers grown in Gainesville in 1983, while in Hastings, the specific gravity of tubers increased slightly with N rate in 1983 up to 180 lb/acre N (1.084) and increased quadratically through 120 lb/acre N (1.079) in 1985.
The Hastings experiment summarized above resulted in higher yields than the Gainesville experiment. Researchers did not attribute the Hastings yield advantage to the 3:1 ratio of NH4 to NO3-N fertilizer applied at planting, but earlier Hastings experiments resulted in higher yields with unequal proportions of NH4 to NO3-N at planting (Hensel and Locascio, 1987). This previous experiment was conducted in the spring of 1986 on subsurface-irrigated Ellzey sands. Fertilizer was applied in a 3 x 3 x 3 factorial treatment arrangement with 100, 200, or 300 lb/acre N applied in proportions of 5, 25, and 45% from NO3-N. The total amount of N applied at planting increased from 0 to 33 to 67% with the remainder applied 33 days later.
Strong interactions resulted among all factors. A yield reduction occurred when 5% NO3-N was applied with 67% of the total N (at 300 lb/acre) applied at planting. Higher rates of ammoniacal N applied at planting resulted in reduced tuber set and lower yields. Researchers concluded that optimum yields depended on the optimum combination of NO3-N and NH4-N applied at planting and on how much N was applied at planting and later as a side-dress application. Based on data from this experiment, researchers recommended application of 25% NO3-N at planting with 67% of the total N (200 lb/acre) applied at planting and 33% applied at a later side-dress. Higher yields would not be expected at N rates greater than 200 lb/acre.
The importance of NO3-N in potato plant and tuber development was described in 1950 (Volk and Gammon, 1950). Researchers determined that a condition of potato plants characterized by leaf roll in new leaves, which becomes most pronounced at blossom time, was related to high soil NH4-N concentration. Tubers of affected plants were found to develop too closely to the stem for normal enlargement. Disease was not a factor in expression of these symptoms. Soils at the Federal Point and Bimini (Hastings area) sites were found to be very acidic and had insufficient bacterial activity to convert NH4-N to NO3-N. Field studies were conducted using fertilizers with varied ratios of NO3 to non NO3-N applied in combination with lime treatments. Potatoes were planted on plots where leaf-curl had occurred in 1949. Yields were 46% higher when fertilized with 5N-6P2O5-6K2O where 40% of the fertilizer N was from a NO3 source (241 bushels/four plots, unspecified plot size) than where NO3-N was omitted from the fertilizer mix (165 bushels/four plots). Correction of the soil pH did not eliminate leaf roll incidence. Plants grown on dolomite-treated soils (pH neutral) and fertilized with 5N-6P2O5-6K2O without NO3-N, resulted in lower yields (183 bushels per four plots). On soils where leaf roll had not previously occurred and the pH was 4.7 to 4.9, yields were also improved by application of 5N-6P2O5-6K2O, with 40% NO3-N (292 bushels per four plots). Further yield increases resulted when the fertilizer application was split, 60% at planting and 40% at 60 days (331 bushels per four plots). The difference in these two yield responses was attributed to leaching losses of NO3-N when applied entirely at planting. Application of some NO3-N was further cited for yield improvements during water stress periods when soil nitrification was reduced.
Nearly 20% of the state's potato production is in southern Florida. Experiments were conducted near Homestead, Florida, during the winter seasons of 1993-1994 (grower 1), 1994-1995, and 1995-1996 (growers 1 and 2) (Hochmuth et al., 1998). Nitrogen was applied at 0, 40, 80, 120, or 160 lb/acre at all sites except in 1994-1995 (grower 1) where 0, 50, 100, 145, or 190 lb/acre N was applied (Table 1). A grower fertilization program was included at each location and N rates with these treatments ranged from 96 to 115 lb/acre. Yields are typically lower in the Homestead area than elsewhere in Florida due to the winter production season.
Yields of size A potatoes and total marketable potatoes in most years increased with N fertilization (Table 1). Marketable potato yields increased through N rates of 190 and 160 lb/acre (198 and 141 cwt/acre, respectively, 100% RY) in two experiment seasons, decreased with N from 100% RY (83 cwt/acre) with 0 lb/acre N to 81% RY with 160 lb/acre N in a third study, were not affected by N in a fourth study, and had no discernible yield response to N in a fifth study. Leaf-tissue N concentrations were adequate or in excess of the sufficiency range in all experiments and increased or remained unchanged by N fertilization. Yields with the grower fertilization programs were not different from yields with experimental N rates.
Research was conducted from 2002 through 2004 at the UF/IFAS North Florida Research and Education Center in Live Oak, Florida, to study the effect of initial application of N made at planting (65 lb/acre) or the same amount at emergence (Hochmuth and Jones, 2004). Total N was the same (195 lb per acre) with both timing treatments. There was no significant effect on the total marketable yield due to the N timing treatment during all three years except for Size A tubers in 2002 in which the yield was greater with N application at plant emergence (268 cwt/acre) than that applied at planting (205 cwt/acre). Hence, the initial application of N can be withheld until plant emergence thereby reducing the risk of N leaching losses and still maintaining the same yield. Further studies with center-pivot irrigated potatoes in Suwannee County showed small amounts of N can be applied with the tuber at planting and that yields were similar with 210 and 240 lb N per acre (Hochmuth, 1994).
A series of experiments with Controlled Release Fertilizers (CRF) was conducted during the early 2000s in Hastings. A CRF system improved the nitrogen-use efficiency (NUE) compared to conventional fertilizer practices (Hutchinson, 2004). Fertilizer treatments consisted of CRF1 (166 kg/ha of N) and conventional fertilizer (Grower Standard Program, GSP at 207 kg/ha of N) in 2002 and CRF1 and CRF2 at 166 kg/ha and 164 kg/ha of N, respectively, in 2003. In 2002, tubers in CRF1 treatment were larger than those with the GSP; and in 2003, no significant difference between treatments was observed. The authors reported that the tubers were unmarketable due to poor quality from Internal Heat Necrosis (IHN) and N in GSP leached from the production area resulting in N stress during the active growing period for the potato.
An experiment was conducted in northeast Florida to evaluate the effects of CRFs and soluble fertilizers for subsurface irrigated Irish potato production (Hutchinson et al., 2003). Potato yield and tuber size were very high (approximately 4.5 times higher than without N) when grown with soluble and CRF fertilizers. CRF (15-9-12) resulted in the highest marketable and total yields (387 and 423 cwt/acre respectively) for N applied at the rate of 200 lb/acre. Treatments using CRFs resulted in higher yield (335 cwt/acre for 200 lb/a) with high tuber quality and specific gravity. Leaf N concentration with CRF 15-9-12 at 100 lb/A N was in the sufficiency range and significantly higher when compared to other fertilizers applied at the same rate. Though the nitrogen-use efficiency (NUE) was similar among all fertilizer sources at higher N rates, CRFs had a higher NUE than soluble fertilizer at low N rates, and CRFs had the ability to maintain a nutrient release pattern even in leaching conditions. Therefore, under leaching conditions, the NUE of CRFs is expected to be higher than for soluble sources at all N rates.
In another study conducted at the University of Florida farm in Hastings, the yields of total and marketable-size potatoes were compared for different CRFs (applied at 0, 130, and 220 lb/a) and soluble fertilizer (Pack et al., 2006). The potatoes grown with CRFs had higher yields than using soluble fertilizer or no N treatment, and there was no significant difference in the yields within each CRF product (Figure 5).
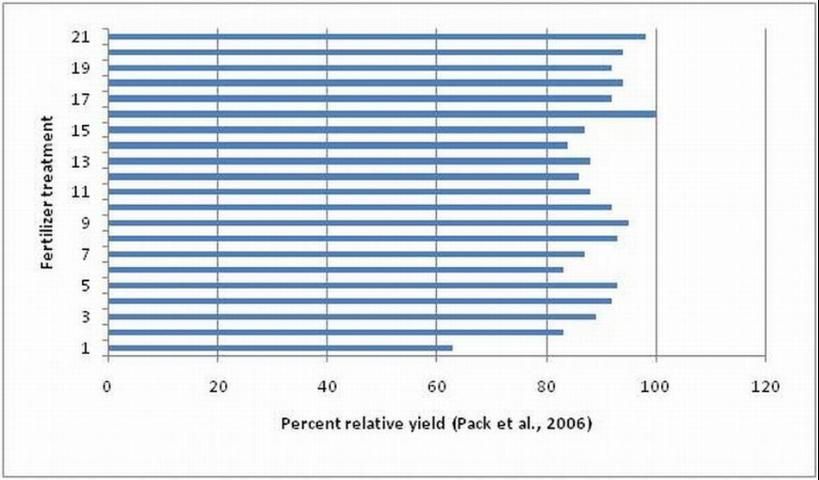
Three N CRF products (A, B, C) engineered to release completely by 120, 75, and 45 days after application were compared to no nitrogen and ammonium nitrate treatments (Figure 6). Plants in the A:B:C (33:33:33) treatment produced significantly more yield than plants with other treatments, and they exhibited 69% less Internal Heat Necrosis compared with potatoes grown with ammonium nitrate (at 200 lb N/A, BMP recommended by UF/IFAS) (Hutchinson et al., 2005). It was recommended that growers consider B:C (67:33) and A:B:C (33:33:33) treatments for commercial development, and these would reduce applied N in the Tri-County Agricultural Area (TCAA) by 25% less than the BMP rate (200 lb/acre), thereby drastically reducing N-leaching.
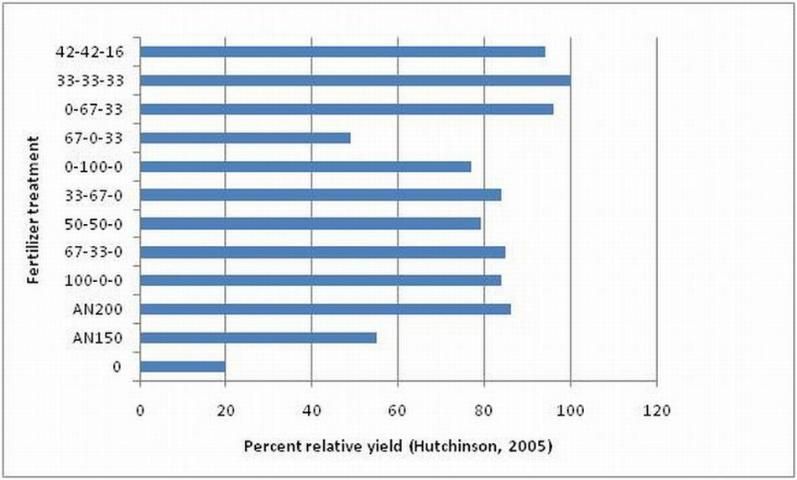
Need for inorganic fertilizer may be reduced by planting legumes (sorghum-sudan grass and cowpea) as summer cover crops and/or fall cash crops (green beans and fallow) and potatoes in spring. The experiment was conducted at the University of Florida's Plant Science Research and Education Unit in Hastings, Florida, using different treatments with 2x2x5 factorial design with four replications. Treatments of N at 100, 150, and 200 lb/acre resulted in an average of 307 cwt/acre total tuber yield, which was greater than with 250 lb/acre of N (267 cwt/acre). Though there was no significant interaction between the summer cover crops and N rate, there was a significant interaction between the fall cover crop and potato N rate. Marketable production was 89 cwt/acre with green bean-0 lb/acre of N treatment when compared to fallow-0 lb/acre; and tubers were larger than that produced in the fallow plots (Hutchinson and Mylavarapu, 2003). Readily available N will be helpful in increasing the tuber size, yield, and specific gravity and can be obtained by planting a fall crop more so than a summer legume cover crop alone.
Several fertilization programs were evaluated for their impact on yield and quality and on N-use efficiency of potato (Hutchinson et al., 2003). At least one CRF at 150 lb N per acre led to yields equal to those with soluble N at 200 lb N per acre. N-use efficiency was greatest with the CRFs at the lower N rates. Various fertilizer strategies were evaluated in 2007, including CRFs and liquid fertilizer formulations (Chen and Hutchinson, 2008). Yields were greater with 200 lb/acre N compared with the 250 lb/acre, which was closer to the commercial-grower rate. Yields with CRFs at 175 lb/acre N were similar to those with ammonium nitrate at 200 lb/acre N. N recovery efficiency was greatest with CRFs. There were no differences among the fertilizer treatments for tuber quality.
The research with CRFs summarized above showed that CRFs at 150 to 175 lb/acre N can result in potato yields equal to those with the current N recommendation of 200 lb N per acre with soluble sources (Hutchinson and Simonne, 2003). These authors summarized some costs associated with N fertilizer programs. CRFs were more expensive than all-soluble N fertilizer programs in terms of fertilizer materials costs, but these authors listed several benefits of CRFs that should be considered in designing the fertilizer program.
Many of the reviewed studies above involved only two rates of N often in factorial combination with other potato production factors. As such, some readers may not consider these studies as covering the full range of expected response. The data plotted in Figure 7 came from more recent studies where N rate was a variable in the experiment. At least three levels of N were needed to qualify the study for inclusion in the graph. Figure 7 makes it somewhat easier to visualize the average response to N rate and evaluate if the average response is close to or far from the current recommended target level of N (200 lb/acre N). Yield response was increased with added N between 0 N and 150 lb per acre N. Yield leveled off after 150 lb/acre N, and even showed a tendency to decline after 225 lb/acre N. Further analysis of the data in Figure 7 shows most of the responses to N rate at or in excess of 95% relative yield occurred with between 150 and 225 lb/acre N (Figure 8). For most studies, responses between 95 and 100% RY (highest yields) were not significantly different. If the argument is made to include occurrences between 90 and 94% RY (lower yields), then more studies show those occurrences in the higher rates of 300 lb/acre or greater. This finding indicates that yield actually declined as N rate exceeded 225 lb/acre. These recent N studies clearly support a target N rate between 150 and 225 lb/acre N, with emphasis toward the lower part of that range.
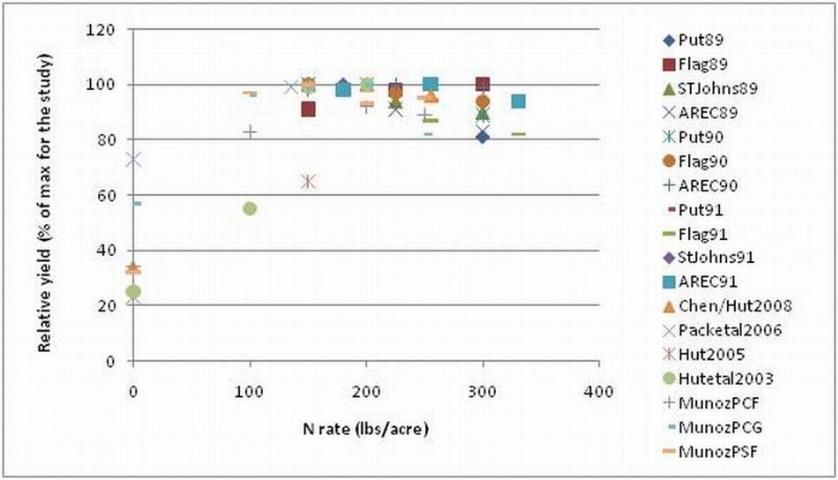
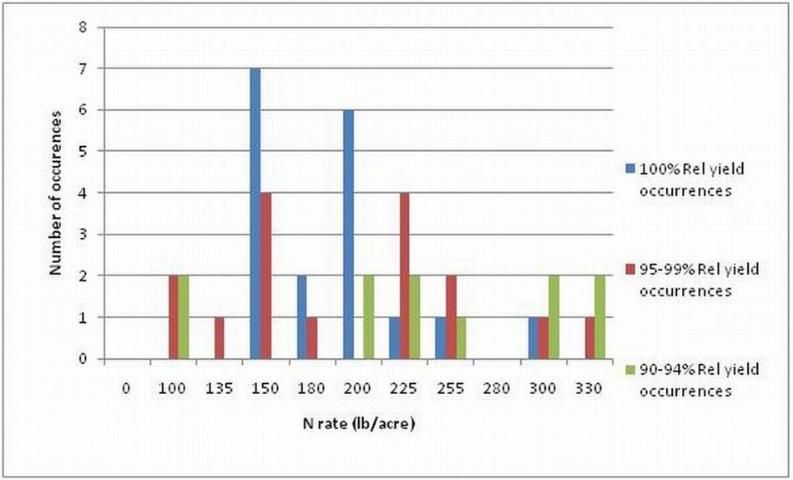
Data from these studies where at least three N rates were included can be used to illustrate the usefulness of the percent relative yield approach. In Figure 9 with the actual units of cwt/acre, there is considerable scatter and it is difficult to see the underlying trends in the data set. The presentation in Figure 7 using the converted data makes it much easier to see the underlying trend in the data set. Further, data in Figures 9 and 10 show that 1991 was a low production year, while 1990 was a higher production year. This shows that there are other factors involved in potato yield from year to year. This analysis should provide support that the percent relative yield conversion is a helpful technique to assist in visualizing response trends using several data sets over years.
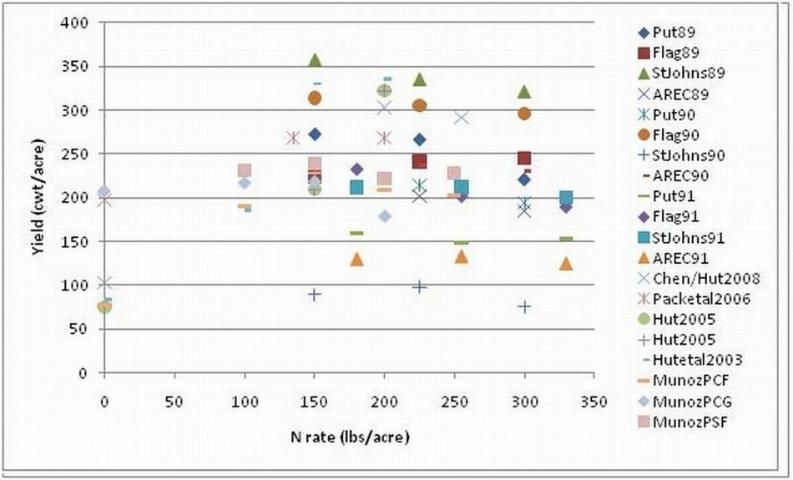
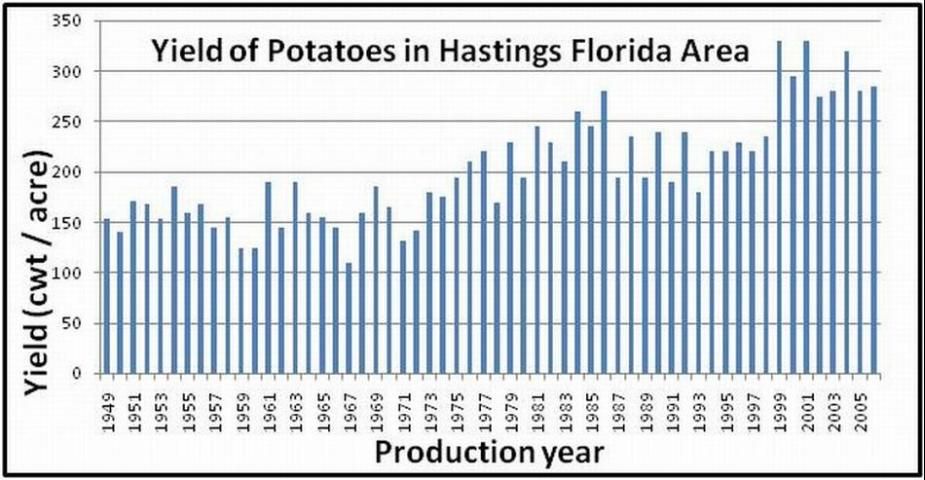
Impacts of N Fertilizer and Irrigation Management Programs on Water Quality
Irrigation and fertilizer management are linked when considering the impacts of fertilizer losses on water quality. Potatoes in most production areas of the state, including northeastern Florida and southwestern Florida, use subsurface irrigation. The crop water efficiency with this system is less than with other systems (Smajstrla et al., 2002), but there has been little research on alternate irrigation systems for potatoes. A recent review of the literature summarized the national research picture with potato fertilizer and irrigation management (Munoz et al., 2005). The fact that the potato plant has low N-use efficiency and that the crop is typically grown on sandy soils may lead to more risk to the environment from poorly managed fertilizer and irrigation programs. More research is needed on potato plant nutrient uptake patterns and nutrient mass balances in typical production systems. Research in Florida with subsurface irrigated potato showed water table depth was a good indicator of soil moisture. Early work (Hensel, 1964) showed that a water table maintained at 8.7 inches below the row alley was optimum for yield. Wetter and drier conditions led to yield reductions. Water table depth needed to be managed during the season to optimize soil moisture consistency and minimize tuber physiological disorders such as hollow-heart and brown center. Researchers noted that an irrigation schedule based on physiological age of the crop would be a preferred irrigation management strategy rather than a single water table depth employed throughout the season (Munoz-Arboleda and Hutchinson, 2006). This improved strategy would result in greater water conservation.
In a potato field near Hastings, three water furrows used for both drainage and irrigation were replaced by subsurface drains (Rogers et al., 1975). Subsurface drains were more efficient than water furrows during harvest periods when excessive rains could have delayed harvesting for several days. The water table was adequately maintained at the desired level by raising and lowering the drain outlet during periods of irrigation. Since the subsurface drains allowed planting of 18 rows between ditches, instead of the usual 16, the yield per unit area was increased significantly.
A study was conducted during 2001, 2002, and 2003 at the University of Florida Institute of Food and Agricultural Sciences to determine the amount of NO3-N fertilizer in the subsurface water table under the crop. A crop rotation plan was followed and identified as potato-sorghum-fallow (PCF), potato-sorghum-green bean (PCG), potato-cowpea-fallow (PCF) and potato-cowpea-green bean (PCG) (Munoz-Arboleda et al., 2008). Ammonium nitrate at 100 lb/acre was incorporated on the subplots and the remaining treatment amount was side-dressed two weeks after the plant emergence. No additional fertilizer was applied for sorghum or cowpea, which was planted after harvesting potato, but 125 lb/acre N was applied for green bean, which was planted after sorghum or cowpea had been harvested. The NO3-N concentration in the perched groundwater was proportional to the rainfall magnitude after potato planting (which was observed during the first and third year of the experiment). Also, the NO3-N concentration increased to more than twice that of the concentration in fallow plots due to the fertilization of green been. Sorghum was a better N scavenger as a summer cover crop as the concentration did not increase when compared to snap bean. Therefore, alternatives such as CRFs and summer cover crops with high nutrient scavenging capacity could reduce the risk of nitrate leaching during and after the potato season by synchronizing the availability of N with plant demand and by recovering any residual N from the potato season, respectively. In three years of field research, potato yield response to N rate was maximized (quadratic model maximum) at or below the recommended rate of 200 lb/acre, yet there were linear increases in N crop uptake (Munoz, 2004).
Studies were conducted to evaluate the fate and movement of N and P in Deep Creek in the lower St Johns River watershed (Worthington and Hutchinson, 2008). Total P and chlorophyll a were correlated suggesting that this creek was N-limited. Studies such as these are needed to better define the limiting nutrients in water bodies.
Nitrogen Summary
The earliest research on N fertilizer's effects on potato yield consisted of mixed-fertilizer studies beginning in the 1940s and continuing through 1967. The effects of increasing N rates, associated with the mixed-fertilizer treatments, were presented graphically against RYs in Figure 1. Yields generally increased through 175 lb/acre N, and then leveled off or declined with N rates greater than 200 lb/acre. The variation of potato yield decreased with additional N fertilizer within the range of 150 to 200 lb/acre. Among other findings during this research period, one finding was that side-dress fertilizer applications were most effective on soils with low organic matter content as long as the application was made no more than 40 days after planting. Wet soils, measured at 12% moisture content on an oven-dry basis, increased potato disease susceptibility and resulted in the lowest yields, while soils with 10% moisture content resulted in the highest yields. An 8-inch seed spacing was the most profitable plant spacing and polyethylene mulch use increased potato yields, but the authors did not evaluate the economics of mulch use on potato production.
Yields of potatoes responded positively (96 to 100% RYs) to N fertilization rates to 200 lb/acre in nearly two-thirds of experiments. Marketable yields were often reduced by N fertilization at rates greater than 225 lb/acre N. Nitrogen fertilization demonstration trials on commercial farms showed that potato yields with 225 lb/acre N were the same as yields with 300 lb/acre N in 11 out of 16 trials (Hochmuth et al., 1993). In three trials, yield was reduced when N fertilization was increased in excess of the range from 150 to 300 lb/acre N. Reports spanning more than 60 years of research with potato fertilization showed that potatoes rarely responded positively to more than 200 lb/acre N and often did not respond to more than 150 lb/acre N, especially with controlled-release fertilizers. Concentrations of N in leaf tissue generally reflected adequate N fertilization with greater than 150 lb/acre N. Maximum responses to N rate do not appear to be different across irrigation systems.
Nitrogen and K leach easily in sandy soil, therefore supplemental additions of N and K might be needed after periods of heavy rainfall. Supplemental applications of 30 lb/acre N and 20 lb/acre K2O might be needed after a rainfall of 3 inches in 3 days or 4 inches in 7 days (Kidder et al., 1992). Nitrogen efficiency was not improved by nitrification inhibitors in four out of five studies. Yields of potatoes with NH4NO3 were equal to or better than yields with early CR fertilizers or mixtures of NH4NO3 and CR fertilizers. Newer CRFs led to equal yields, increased nitrogen use efficiency, and less leaching of N, with 150 lb/acre N compared with higher rates of N.
Nitrogen application timing is critical to successful potato production. Approximately two-thirds of the N should be applied at planting or preferably at cracking. The remainder should be applied between 30 and 40 days after planting. About 25% of the total N should be supplied from NO3-N sources. BMPs should give equal consideration to the N rate and the fertilizer and irrigation management strategies.
Sixty years of intensive research with N on potato yields show yields' responses to N ranging from 100 to 400 cwt/acre, depending on the particular study. The majority of research results shows yield leveling off near 200 lb/acre N. Clearly other variables in addition to N control yield in potato. These variables might include disease incidence, insect incidence, temperature, irrigation practices, variety, timing of N application, placement of fertilizer, and many others. These variables might have as large an effect on potato yield responses as N rate, once the N rate reaches 200 lb/acre and has been managed optimally for timing, form, etc., and providing that irrigation has not been excessive. These variables have not received as much attention from researchers as N fertilizer rate.
A Suggested Nitrogen BMP for Potato Production
This BMP takes into account the cultural, economic, and environmental results from the research summarized in this document:
- Use a target seasonal N amount of 200 lb/acre to be modified if needed based on leaching rain or leaf-tissue testing before approximately 40 days after planting.
- Use a cover crop (sorghum for better nutrient scavenging) in the summer to take up residual N, and to provide organic matter for the next potato crop.
- Pre-plant nitrogen fertilizer is not needed. This recommendation was noted as early as 1955 and confirmed in the 2000s. Plant potatoes without soluble N fertilizer, or only with N that might come with the starter P (ammonium phosphates) fertilizer. Hold N to less than 20 lb/acre at planting. Using controlled-release N fertilizer mixtures with various release patterns could save 25% of the recommended N rate of 200 lb/acre. If not using CRF, make the first application of N (up to 67%) at cracking (about 14 days after planting).
- Apply remaining N within 40 days after planting.
- Apply 30 lb/acre N after any leaching rain. This rate could be added to the second N application if the leaching rain occurred just prior to the second application.
- Manage irrigation according to the recommended practice; especially avoid fluctuating water tables.
Phosphorus and Potassium Soil Testing
Knowledge of soil nutrient levels (particularly P and K) before planting is the starting point to predicting crop response and requirements for P and K fertilizer. Mehlich-1 extractant indices (expressed as ppm soil-extracted nutrient) are classified as very low, low, medium, high, and very high, and a crop specific fertilizer recommendation is made from that classification (Hochmuth and Hanlon, 2000; Mylavarapu, 2009; Rhue and Kidder, 1984). In 1979, the M-1 solution became the accepted extractant standard at the University of Florida. Previous to M-1, ammonium acetate and water extractants were used. Indices recorded from these methods cannot be directly equated with M-1 indices or fertilizer recommendation rates but review of the older research results with these extractants presents a profile of crop response to fertilizer in varying conditions. For potato, yield response did not correlate with soil K concentrations and a recommendation of 140 lb/acre K2O was made for all soil test K indices. Water management practices, fertilizer sources and application methods, and the effect of mulch in the nutrient management system are also summarized.
Phosphorus
Phosphorus experiments were conducted at Hastings field sites on previously farmed or "old" soils (cropped for the previous 60 years) and on newly cleared Ona fine sand soils (Hensel, 1962a). Other old and newly cleared sites were chosen for experiments in 1962. Fertilizer was applied in bands below and to the side of the seed pieces at rates of 150 lb/acre N, 240 lb/acre K2O, and P2O5 rates of 0 to 400 lb/acre (1960 and 1961) and in factorial combinations of three N rates, 70, 140, and 210 lb/acre with three P rates, 80, 160, and 240 lb/acre P2O5 (1962). Soil P concentrations in 1960 to 1961 were reduced from 65 to 50 ppm, measured by ammonium acetate extraction, on old soils and from 8 to 6 ppm on newly cleared soils. On old soils, potato yields were similar for plants fertilized with 0 to 400 lb/acre P2O5. Average yields were 181 and 316 cwt/acre for 1960 and 1961, respectively. Plants on newly cleared soils required 400 lb/acre P2O5 (significance not described) each year to produce optimum yields of 157 and 242 cwt/acre (100% RYs) as compared to 15% and 31% RYs with 0 lb/acre P2O5. In 1962 experiments, yields were optimized on old and new soils with 240 lb/acre P2O5 (146 and 177 cwt/acre, respectively, 100% RYs). Slightly lower RYs (98 and 97%) resulted with 160 lb/acre P2O5 applied to each soil type, and significant differences among yields were not described. Soil P concentrations (ammonium acetate) were 18 ppm (old soil) and 21 ppm (new soil). At planting, an optimum potato soil pH of 5.5 existed at all sites with 550 lb/acre of hydrated lime applied in 1960 to increase the pH on new soils.
In another experiment, potato yield response was evaluated following P applications for the three consecutive seasons, 1960, 1961, and 1962, on Ona and Kanapaha fine sands (Locascio and Breland, 1963). Researchers sought to determine the effects of different soil types on P recommendations for potato. Kanapaha soils were described as having twice the P content of the Ona soils, though soil P analytical results were not provided in the report. Concentrated superphosphate (CSP) was broadcast before a fall snap bean crop at rates of 0, 90, and 180 lb/acre P2O5. The potato crop was planted the following spring after broadcast application of 90 lb/acre N (NH4NO3) and 90 lb/acre K2O (K2SO4). An additional 30 lb/acre N and K2O were side-dressed each season. Except for potatoes grown on Kanapaha soil in 1960, potato yields increased linearly in all years on both soil types through P rates of 180 lb/acre P2O5. Averaged using all seasons, yields from Kanapaha soils were 172 cwt/acre (100% RY) with 180 lb/acre P2O5 and were 126 cwt/acre (75% RY) with 0 lb/acre P2O5. A similar average yield increase occurred on the Ona soils with applied P from 0 lb/acre P2O5 (117 cwt/acre, 72% RY) to 180 lb/acre P2O5 (161 cwt/acre, 100% RY). Although yields were similar with both soils, the leaf-tissue P concentrations were nearly double in potato plants grown on the Ona soil. Based on these leaf P concentrations and soil data (not presented), researchers concluded that more P was fixed in an unavailable form on the Kanapaha soils than in the Ona soils.
Initiation of Mehlich-1 (M-1) soil testing research in 1977 renewed interest in P fertilization of Hastings-grown potatoes where previously uncropped soils were typically low in P compared to soils in potato production for sixty to seventy years (Rhue et al., 1981a; Rhue et al., 1981b). Lime was applied in November 1977 to raise the pH of uncropped soils from 5.1 to 6.5 before seeding with 'Atlantic' potato pieces in February 1978. Applied P rates were 0, 115, 230, or 345 lb/acre P2O5 from diammonium phosphate (DAP) or liquid ammonium polyphosphate (APP). Fertilizers, including 140 lb/acre N and 180 lb/acre K2O, were applied in two bands 8 inches apart and one band 8 inches beneath the seed piece followed by side-dressing at 35 days with 50 lb/acre each of N and K2O. The water table was maintained 8 inches beneath plot alleys throughout the crop season. On these Placid sandy soils with 5 ppm M-1 soil-extracted P, significant marketable yield increases occurred between 0 lb/acre P2O5 (60 cwt/acre) and 115 lb/acre P2O5 (150 cwt/acre, 100% RY). Thereafter, P rates to 345 lb/acre P2O5 had no further effect on marketable yields. Yields of grade B potatoes were lower (1% probability) with APP than with DAP. Research with soil testing for P with M-1 is presented in Figure 9.
In 1979, M-1 soil tests revealed P concentrations had increased due to the 1978 applications of DAP or APP to 12 and 11 ppm with 115 lb/acre P2O5 of each respective P source, to 24 and 21 ppm with 230 lb/acre P2O5, and to 35 and 24 ppm with 345 lb/acre P2O5. To these residual P concentrations, half of the plots received no P and the other half received 115 lb/acre P2O5. 'Sebago' potatoes were planted and N and K were applied as in 1978. On those soils where no P was applied, yield response to increasing residual soil P concentrations was quadratic with yield leveling off with 25 to 35 ppm extracted P (308 cwt/acre; 100% RY). Potatoes receiving 115 lb/acre P2O5 produced similar yields to those that received no P. Researchers concluded that 35 ppm of M-1 soil-extracted P provided sufficient nutrient for optimum potato yields and no more than 115 lb/acre P2O5 were needed when soils tested less than 30 ppm.
In 1980, all plots received 115 lb/acre P2O5 except the 0 P control treatment. Similar pre-fertilization concentrations of M-1 soil-extracted P resulted with P sources DAP and APP. Average soil P concentrations for each rate of previously applied P were 8 ppm (0 applied P), 20 ppm (115 lb/acre P2O5), 28 ppm (230 lb/acre P2O5), and 35 ppm (345 lb/acre P2O5). Optimum yields occurred on soils testing 35 ppm P (294 cwt/acre, 100% RY). Response to the added 115 lb/acre P2O5 was more apparent this season than the previous season where yields were similar with or without the added P. Where soils were very low in M-1 P (<10 ppm) (1978) yields were significantly higher with DAP than with APP, but both P sources performed equally on soils with M-1 P concentrations greater than 10 ppm (1979 and 1980).
While these experiments were conducted, simultaneous experiments (1978 and 1980) were conducted on a nearby high-P site (237 ppm), which had been in potato production for the previous seventy years. Phosphorus rates of 0, 50, or 100 lb/acre P2O5 were applied from DAP or APP, and N and K were applied at 140 lb/acre N and 180 lb/acre K2O banded preplant with 50 lb/acre of each side-dressed 35 days later. Yields of A and B grade marketable potatoes were not different with either DAP or APP. Averaged marketable yields using both P sources did not increase compared with 0 lb/acre of P2O5 (180 and 321 cwt/acre each season,100% RY). The Placid soils tested here were typical flatwoods soils, pH 5.3, where a 1977 application of dolomitic lime at 2800 or 5600 lb/acre elevated the pH to 6.5 (a 0 lime treatment was also included). Application of lime did not interact with applied P for effects on potato yields.
The above summarized Hastings trials of 1978, 1979, and 1980 on old, high P soils were extended through 1981 and 1982 (Yuan et al., 1985a; Yuan et al., 1985b; Yuan et al., 1985c). In 1981, researchers noted that higher M-1 soil P concentrations, 340 ppm, resulted in plots where 5600 lb/acre of dolomite was applied in 1977 compared to 259 ppm P where no dolomite was applied in 1977 (Rhue et al., 1981a). They concluded that Ca from previous lime applications had acted to retain P in the soil, which would otherwise be leached from acidic soils low in Fe and Al. Phosphorus combined with Ca was held in the soil in a form available to plants. The potential water solubility of P, greater in unlimed soils than limed, was also examined in this study where P leaching was reduced by maintenance of the water table at 8 inches below the alley bottom. The authors concluded that water table maintenance and lime, applied as needed, were important to proper soil P management on long-term cropped sandy acidic soils.
By extending the experiment an additional two crop seasons, researchers also sought to observe the long-term effects of accumulated P on potato yields and determine how much soil P was removed with successive potato harvests. No additional lime or P was applied to the high-P, Ellzey sand soils (formerly termed Placid sand), and N and K applications were reduced to 125 lb/acre N and 170 lb/acre K2O. All other experimental conditions were the same as before.
Residual M-1 soil-extracted P concentrations from plots fertilized in 1980 with 0, 50, and 100 lb/acre P2O5 were 308, 272, and 292 ppm, respectively (1981) and 235, 229, and 240 ppm, respectively (1982). The reduction in soil P content was attributed to leaching and nutrient removal by the crop. Tuber yields in 1981 and 1982 were similar with all applied P rates averaging 257 and 293 cwt/acre each year. Dry weight tissue analysis revealed N and K concentrations were 10 to 100 times higher in the vegetative tissue of the potato plant than in the tuber. Phosphorus concentrations in the tuber, however, were 35% higher than P concentrations in the vegetative tissue. Early P uptake with potato is known to promote good tuber development, and ultimately most of the absorbed P is translocated to the tuber. The measured annual amount of P removed from the soil with harvest was between 30 to 40 lb/acre P2O5 in 1980 and 1981 harvests. Extraction of soil P by vegetative tissues was quadratic in 1981 and linear in 1982, but extraction of P by tubers did not differ with soil P concentrations. The authors concluded that potato tuber harvest and export would not deplete high-P soils sufficiently to require additional P application for a long time.
Spurred by the research in Hastings, a Flagler County potato experiment was conducted in 1981 to determine if the basic recommended P rate for irrigated mineral soils, 200 lb/acre P2O5 (Montelaro, 1978; Stall and Sherman, 1984), was too high (Kidder, 1982). Soil from the chosen field tested 10 ppm in M-1 soil-extracted P and had previously been cropped in cabbage, but was uncultivated before 1979. The very low soil-P concentration predicted a yield response to applied P (CSP) at the recommended rate of 200 lb/acre P2O5 or with the lower rate used earlier in Hastings, 115 lb/acre P2O5. Nitrogen (NH4NO3) was applied at 170 lb/acre N in all treatments while K (KCl) was applied at either 0 or 135 lb/acre K2O. Grower fertilizer rates of 225-60-135 lb/acre N-P2O5-K2O were applied to an adjacent commercial field area. When the potato seed pieces sprouted, fertilizers were banded 4 inches to the side of the plant row and 4 inches beneath the bed surface. Potato yields were not different with any experimental fertilizer rate, but yields were significantly lower with the grower fertilizer rates of 60 lb/acre P2O5 (260 cwt/acre) compared to yields with 115 lb/acre P2O5 (347 cwt/acre, 100% RY). Insufficient P (60 lb/acre P2O5) was cited as the limiting nutrient in the grower treatment, while 115 lb/acre P2O5 resulted in high yields on these very low P soils. The author suggested that additional research be conducted before reducing the recommended P rate from 200 lb/acre P2O5.
Researchers applied 180 lb/acre P2O5 from four P sources in experiments in 1980 and 1981 near Gainesville (Locascio and Rhue, 1990). Two liquid P sources were tested on beds of St. Johns fine sand, ammonium containing ortho-P and APP along with tests of two granular P sources, DAP and CSP. Beds were fertilized with 80 lb N (NH4NO3) and 95 lb/acre K2O (KCl and K2SO4) applied in two bands placed 2.5 inches to each side of the bed center and 2 inches below the seed. 'Atlantic' seed potatoes, planted in mid-February each year, were side-dressed six weeks later with 80 lb/acre N and 100 lb/acre K2O. Potatoes were overhead irrigated as needed.
Yields on the low P soils, 15 ppm M-1 P, in 1980 were highest with APP (154 cwt/acre), significantly lower with ortho-P and CSP (133 cwt/acre), and lowest with DAP (108 cwt/acre). These findings directly conflict with earlier P source findings (Rhue et al., 1981a; 1981b) on Placid (now Ellzey) sands (Hastings) where higher yields resulted with DAP compared to other P sources. Application of P sources APP, ortho-P, and CSP resulted in similar yields (180 cwt/acre) in 1981 on very low P soils, 8 ppm M-1 P, and lowest yields with DAP (150 cwt/acre). When measured after harvest, Mehlich-1 soil P concentrations increased 14 ppm on average with DAP and CSP compared to 9 ppm on average with liquid APP and ortho-P. Greater plant uptake of P from DAP was measured in recently matured leaves at flower formation with 0.63% P (1980) and 0.56% P (1981), but both concentrations were in the sufficient range.
Research with the Mehlich-1 extractant is summarized in Figure 11. Nearly all of the crop response to P fertilization occurs on soils with less than 40 ppm M-1 P. This soil testing research, along with the crop response results, led to a re-evaluation of the P fertilizer recommendations. By 1989, P recommendations for soils very low (<10 ppm P) and low (<15 ppm P) in M-1 soil-extracted P were revised to 120 lb/acre P2O5 based on yield responses summarized above and additional field testing (Kidder et al., 1989). Researchers in St. Johns, Putnam, and Flagler Counties, including a research center in Hastings, confirmed the 1989 P recommendation of 0 lb/acre P2O5 for soils high and very high in M-1 soil-extracted P (Hochmuth et al., 1993b) during four consecutive crop seasons from 1988 to 1991. Soils from all sites each year had high or very high P concentrations except the St. Johns site in 1991, which had medium P concentrations. Experiments were conducted to compare varying rates of N and K fertilizer; P fertilization was generally omitted from all but the grower fertilization program. No yield advantage occurred with grower P applications from 10 to 90 lb/acre P2O5 (CSP) compared to yields where no P was applied. As a result of these experiments, P recommendations were not changed in 1995 (Hochmuth and Hanlon, 2000) from the 1989 recommendations. Five on-farm experiments were conducted in the late 1990s to test the P recommendations. Soil at four sites tested high and very high in M-1 P content and one site tested low. There were no significant responses to P fertilizer (Hochmuth et al., 2002), demonstrating that P applications are not warranted on high-P testing soils. The average yield using all sites was 324 cwt/acre.
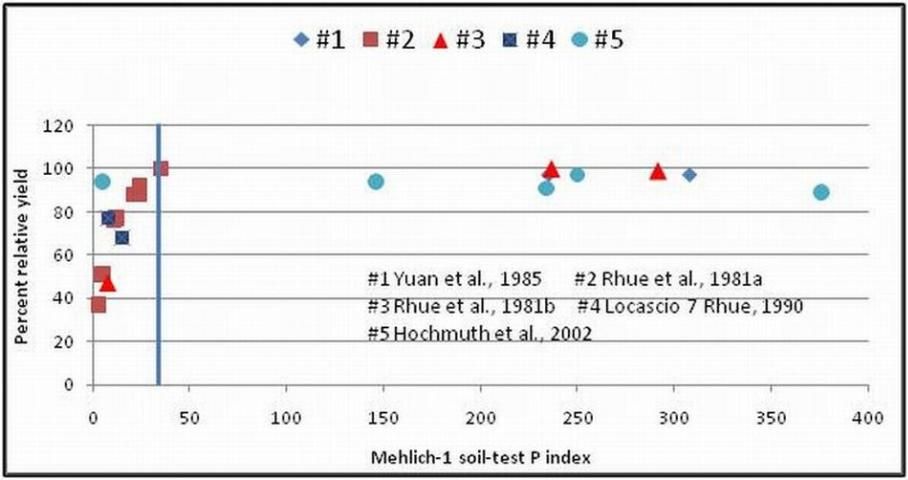
Five experiments were conducted in Homestead, Florida, among the South Florida areas where 15-20% of state potato production occurs. These experiments were conducted during the winter seasons of 1993-1994 (grower 1), and 1994-1995 and 1995-1996 (growers 1 and 2). Due to the marl and rockland soils in this area, the ammonium bicarbonate-diethylenetriamine pentacetic acid extractant, AB-DTPA, soil extractant was used. Results of this soil extractant cannot be directly related to results with the M-1 soil extractant.
Yields did not respond to P fertilization at rates greater than 100 lb/acre P2O5 in all five experimental seasons. Optimum, 100% RY was produced with 100 lb/acre P2O5 (84 cwt/acre) in one experimental season, with 0 lb/acre P2O5 in two experimental seasons (191 and 140 cwt/acre), and there were similar yield results with all P rates in two additional experimental seasons. Because yield responses to increased P fertilizer varied on sites with similar soil P concentrations, further experiments are needed to calibrate AB-DTPA soil-extracted P concentrations with yield. Where soil P concentrations were 304 ppm, high yield was produced with 0 lb/acre P2O5 (140 cwt/acre, 100% RY) and 90% RY with 200 lb/acre P2O5, while similar yields were produced with all P rates on soils with 285 ppm AB-DTPA soil-extracted P. Yield responses to P fertilizer in three experiments with 45, 70, and 60 ppm soil-extracted P were quadratic, producing a peak yield with 100 lb/acre P2O5 (84 cwt/acre, 100% RY), were reduced from 0 lb/acre P2O5 (191 cwt/acre, 100% RY) to 96% RY with 200 lb/acre P2O5, and were not affected by P rates to 200 lb/acre. Whole leaf tissue P concentrations were above the sufficiency range in all experimental seasons and were generally not affected by increased P fertilizer.
Phosphorus Summary
Yield responses to applied P rates are presented in Figure 12. Experiments where P was not applied were conducted to evaluate yield response to residual soil P concentrations. Current P recommendations for potato were based heavily on experiments in the late 1970s and early 1980s and confirmed in experiments during four seasons in a tri-county area using M-1 soil extractant data (Hochmuth et al., 1993b), with less emphasis placed on earlier work where ammonium acid extractant was used (Hensel, 1962a). Based on summarized yield responses, P fertilization of soils with high M-1 P concentrations greater than 30 ppm is not warranted.
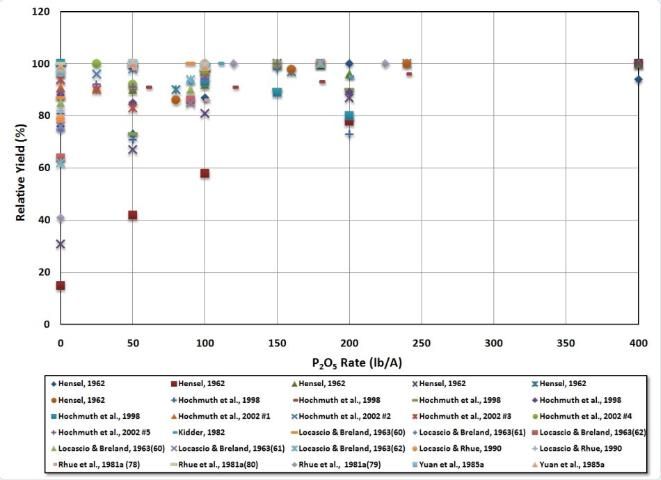
Research with P fertilizer sources provided mixed results. In early work on a Placid (Ellzey) fine sand, which does not fix large amounts of P, potato yields were reduced one year out of three with APP compared to DAP (Rhue et al., 1981a). Other work on St. Johns fine sandy soil testing low in M-1 soil-extracted P showed that yields were generally better with liquid APP, liquid ortho-P, or CSP compared to DAP (Locascio and Rhue, 1990).
The P research cited above led to the current P fertilization recommendations used by the Extension Service and summarized below with the Mehlich-1 P index, the interpretation of the index, and the lb/acre P2O5 fertilizer recommendation in parens:
<10 ppm, very low (120 lb/acre)
10 to 15 ppm, low (120 lb/acre)
16 to 30 ppm, medium (60 lb/acre)
31 to 60 ppm, high (0 lb/acre)
>60 ppm, very high (0 lb/acre)
Phosphorus BMPs Suggested by the Research
- Use the Mehlich-1 soil test to determine P needs and follow the recommendations for amounts of P fertilizer.
- High-P testing soils do not require P fertilizer.
- Given cool planting condition (January) there might be a response to a small amount of P added as a starter fertilizer to encourage young root growth. This information is provided by the footnotes in the UF/IFAS Extension soil testing report.
- Required P fertilizer should be banded near the seed piece at planting time and not broadcast before planting.
Potassium
Potassium plays a role in sugar translocation and starch synthesis in plants. Due to the high starch of the potato tuber, K is an important nutrient in tuber development (Rhue et al., 1986). Analysis of K removed from the soil by tuber and vegetative tissues revealed two-thirds of the removed K had accumulated in the tuber (Yuan et al., 1985c). Measured in crop seasons 1983 and 1984, K removed from the soil by tubers represented from 125 to 150 lb/acre K2O when 140 lb/acre K2O was applied and removal of 130 to 170 lb/acre K2O when 200 lb/acre K2O was applied in 1983 and 1984, respectively.
Researchers sought to compare the University of Florida computerized "Standard Fertilizer Recommendation System," a Mehlich-1 based fertilizer recommendation with actual yield results in a Flagler County 1981 experiment (Kidder, 1982). Potassium (KCl) was applied at 0 lb/acre K2O or the recommended rate of 135 lb/acre K2O for soils then considered medium in M-1 soil-extracted K (81 ppm). Nitrogen, as NH4NO3, was applied at 150 lb/acre and P, as CSP, was applied at 115 or 200 lb/acre P2O5. All fertilizer was applied in a band 4 inches to the side of the emerging potato and 4 inches deep. Yields with 0 or 135 lb/acre K2O were not different, averaging 344 cwt/acre of size A and B potatoes. These yields were considered high compared to the state average yield of 220 cwt/acre for that season. High yields with 0 lb/acre K2O indicated 135 lb/acre K2O, recommended from the "Standard Fertilizer Recommendation System," was excessive for soils with 81 ppm M-1 soil-extracted K.
The effects of excessive K application on soils with high M-1 soil-extracted Ca and P were evaluated in 1983 and 1984 experiments (Yuan et al., 1985c). Humaquept soils at this Hastings site were not tested for M-1 soil-extracted K in 1983 or 1984, and previous K concentrations were 55 and 37 ppm from experiments conducted there in 1981 and 1982, respectively (Yuan et al., 1985a). Following the 1982 and 1983 crop seasons, further reduction in soil K concentrations were expected and K concentrations were presumed to be less than 37 ppm. Potassium was applied at planting in rates of 0, 140, or 200 lb/acre K2O combined factorially with 100 or 150 lb/acre N. A side-dress application of 50 lb/acre N and K2O was applied five weeks after planting. The effect of applied K on yield of size-A potatoes was not significant in either year, resulting in high yields with 200 and 140 lb/acre K2O (199 and 195 cwt/acre, 100% RY) and 88% and 90% RY with 0 lb/acre K2O each year. Yields were averaged using both rates of applied N and approached the annual state average yield each year. Where no K was applied at planting, the K content measured in tuber tissue declined from 1.9% in 1983 to 1.8% in 1984 (tuber tissue is about 2% K). Depletion of soil K was suspected on these 0-K plots. Excessive K application to 200 lb/acre K2O did not reduce yields in either experiment season nor was the concentration of Ca in tuber and vegetative tissue affected by higher rates of applied K.
Researchers sought to determine critical K concentrations in leaf tissue at various sampling dates in a four-year study from 1981 to 1984 (Rhue et al., 1986). Experiments were conducted on Ellzey fine sand soil near Hastings where land had been in potato production for 70 years. Potato yield responses were evaluated with respect to applied K and soil K concentrations determined by M-1 soil analysis each season. Fertilizer application each year included 125 lb/acre N applied at planting in bands to each side of the plant row and 8 inches apart. In 1981, N and K were side-dressed 35 days from planting at 50 lb/acre N and 0, 25, 50, or 75 lb/acre K2O. Researchers expected a yield response to the side-dressed K with a M-1 soil test of 73 ppm K, recommendations of the time were for 135 lb/acre K2O (Rhue and Kidder, 1984). Yields did not change with side-dressed K rates through 75 lb/acre K2O. Optimum yields occurred with 50 lb/acre K2O (328 cwt/acre, 100% RY). Potassium concentrations in leaf tissue were greater than the sufficiency range (3.0 to 4.0%) at first blossom with 0 to 75 lb/acre of side-dressed K2O. Later tissue samples had adequate K concentrations (2.0 to 3.0%) and were unchanged by added K. Interpretation of a M-1 soil concentration of 73 ppm was changed from medium to high in 1989 (Kidder et al., 1989) and the K recommendation was reduced from 135 to 0 lb/acre K2O (Kidder et al., 1989; Rhue and Kidder, 1984).
Soils in 1982 and 1983 experiments tested low in M-1 soil-extracted K, 34 and 32 ppm, respectively. Potassium was applied at 150 or 250 lb/acre K2O in 1982 and at 50 and 250 lb/acre K2O in 1983. Potassium recommendations at the time were for 200 lb/acre K2O. Fertilizer was applied as in 1981 with 125 lb/acre N, 0 or 200 lb/acre K2O applied in two bands at planting followed by a side-dress application of 50 lb/acre N and K2O, 35 days after planting. Yield responded significantly (5% probability) to K rates in both seasons. Optimum yields occurred with 250 lb/acre K2O each year (350 and 260 cwt/acre, 100% RY, each season) and 95% and 88% RY with 150 lb/acre K2O and 50 lb/acre K2O each year, significant at 5% probability. Leaf-tissue K concentrations were within the adequate range of 3.0 to 4.0% at first flower and 2.0 to 4.0% when tubers were half grown with the lowest K application. Leaf-tissue K concentrations in excess of adequate concentrations occurred with 250 lb/acre of applied K2O each year.
In 1984, soils at a Hastings research site tested 39 ppm in M-1 soil-extracted K. Total applied K rates were 100 to 300 lb/acre K2O or rates less than or in excess of the recommended K rate. Side-dress applications of 50 or 100 lb/acre K2O were applied as part of the total K rate. Yields were not different with increasing K rates or with higher amounts of side-dressed K. High yields resulted with 300 lb/acre K2O (273 cwt/acre, 100% RY) averaged using side-dress applications of 50 or 100 lb/acre K2O. All yields exceeded the average yield for the Hastings area of 240 cwt/acre for these years. Rates of K fertilization through 250 and 300 lb/acre K2O did not result in yield reduction in any season. Leaf-tissue K concentrations increased significantly with higher K rates though tissue concentrations were adequate with the lowest rate of applied K, 100 lb/acre K2O. Researchers concluded that a critical leaf-tissue K concentration could not be determined citing annual differences in leaf-tissue K concentrations with a given K rate to seasonal changes in rainfall, temperature, and the exact time of sampling. However, excessive leaf-tissue K concentration of 7.0% at late season sampling (1984) was indicative that K recommendations of 200 lb/acre K2O were too high for soils with 39 ppm M-1 K. Application of large amounts of K to 485 lb/acre K2O had no effect on yields in three experiment seasons in Hastings from 1984 to 1986 (Locascio et al., 1991; Locascio et al., 1992). Experiments were conducted on Ellzey fine sands to test the yield response of potato to increasing rates of Ca and K and to determine the correlation of Ca and K concentrations with the severity of bacterial soft rot (discussed later). Fertilizer, including 240 or 485 lb/acre K2O (no 0 K treatment) from K2SO4, was applied factorially with 0, 400, and 800 lb/acre Ca. Nitrogen from NH4NO3 was applied at 150 lb/acre. Formulated fertilizer was applied at planting in bands 4 inches to each side of the row and 4 inches below the seed piece. Of the three crop seasons, 1984 was characterized by heavy rainfall, 1985 less than average rainfall, and 1986 was considered very dry. Yields were similar with both K treatments in all experiment seasons with slightly higher yields with 485 lb/acre K2O in 1984 (239 cwt/acre, 100% RY) and in 1985 (277 cwt/acre, 100% RY), both yields were above state average yields for these seasons. In the dry season (1986), high yields resulted with 240 lb/acre K2O (193 cwt/acre, 100% RY), which was less than the state average yield of 262 cwt/acre for 1986. With M-1 extracted soil K concentrations from 38 to 44 ppm during this period, researchers did not expect a yield response to the higher K rate; instead, the specific gravity of the tuber was reduced significantly. In spring 1992, researchers applied several K rates to Ellzey fine sand beds in Hastings including the then-recommended 70 lb/acre K2O (Kidder et al., 1989) for medium M-1 soil-extracted K soils, 46 ppm (Hensel and Locascio, 1992). High yields of size A and B potatoes occurred with 70 lb/acre K2O (267 cwt/acre, 100% RY). This yield exceeded the state average yield for 1992 of 240 cwt/acre. Yields with all K rates, 0, 50, or 70 lb/acre K2O, however, were similar. Twenty treatments were evaluated in this experiment, and five experiments were conducted with K sources, including coated KNO3, uncoated KNO3, KCl, or K2SO4, and 50% coated KCl with 50% uncoated KCl applied at 50 lb/acre K2O. An additional five treatments consisted of these same K sources at 70 lb/acre K2O. Nitrogen was applied from NH4NO3 at 150 lb/acre. The remaining treatments included six treatments with coated KNO3 in combination with 25%, 50%, or 75% coated urea applied at both K rates and two treatments of 50% coated urea combined with 100% KNO3 applied at both K rates. Fertilizer formulations were applied to the bed surface and lightly worked into the soil before planting. Researchers found that no yield variation resulted from any of the K and N source treatments.
Experimentation with K fertilization described below was conducted in a tri-county area surrounding Hastings from 1988 to 1991 (Hochmuth et al., 1993a; Hochmuth et al., 1993b). Yield responses to fertilizer rates predicted by M-1 were variable. Based on yield responses in this series of experiments, researchers concluded that M-1 soil tests on Spodosol or flatwoods soils had little predictive capability for effective K rate recommendations and reliance on soil testing for K was discontinued for potato in this area. A recommendation of 140 lb/acre K2O, irrespective of the M-1 soil test index, replaced the graduated K recommendation made from the M-1 results. From observation of yield results with increasing rates of K fertilization, including the grower fertilization practices, researchers found that potato yield and quality did not increase with K fertilization rates above the 1989 recommended rates (Kidder et al.,1989). In six of seven experiments in 1988 and 1989, yields were similar with application of 0 to 300 lb/acre K2O.
Excessive leaf-tissue K concentrations were measured at early bloom (> 5.0%, 1988) and at full bloom (> 4.0%, 1988 and 1989) with all rates of applied K (Hochmuth et al., 2009; Hochmuth et al., 1993a). In 1990, leaf-tissue K concentrations were within the sufficiency range or exceeded sufficient concentrations at pre-, early-, and full-bloom sampling with applied K rates from 70 to 140 lb/acre K2O.
In 1990 and 1991, four of the eight experiments resulted in linear or quadratic responses to applied K (Hochmuth et al., 1993a; Hochmuth et al., 1993b). Three of these experiments required greater than the 1989 recommended rates (Kidder et al., 1989) to produce optimum yields, the fourth required less than the recommended rate, and the remaining four trials resulted in similar yields with K rates from 0 to 310 lb/acre K2O. Average yield response using all four experiment seasons to K rates of 140 or 160 lb/acre K2O, from 12 of the 15 experiments where these K rates were evaluated, resulted in 97% RY. Based on this average yield response and frequently excessive leaf K concentrations with lower K rates, researchers determined 140 lb/acre K2O was sufficient for optimum yields and adjusted the K rate recommendation to 140 lb/acre K2O for all soils regardless of their concentration of M-1 extractable K (Hochmuth and Hanlon, 2005). Commercial growers surveyed in northeast Florida were found to apply an average of 280 lb/acre K2O (Kidder et al., 1992), therefore the new recommendation could save considerable amounts of K fertilizer.
In 1992 and 1993, large-scale demonstrations were conducted on sites that were a minimum of one acre in size (Hochmuth et al., 1993a; Hochmuth et al., 1993b). These demonstrations were done on commercial-size scale to field-test the new fertilizer recommendations. Experiments in 1992 were conducted with commercial K rate applications from 235 to 280 lb/acre K2O and reduced K rate applications from 135 to 180 lb/acre K2O. Yields with the lesser K rate were generally equal to yields with the higher K rate. Delayed application of the side-dressed K in one year was attributed to lower yields (74% RY) as compared to the grower yields in one of the four experiments. Yields were up to 2.4 times greater than the average Hastings area yield of 200 cwt/acre for this season. In 1993, yields with commercial K rates from 225 to 340 lb/acre K2O were generally equal to yields with reduced K rates from 85 to 190 lb/acre K2O. As with the previous season, yields were up to 1.8 times higher than the average Hastings area yield of 180 cwt/acre for that season. Both commercial and reduced K rate applications were well in excess of 1989 recommended rates based on M-1 soil test results. Poor N or K application timing was cited in two experiments, resulting in lesser yields with the reduced rate compared to the commercial rate. Adherence to the recommended application of 175 lb/acre N, one-half to two-thirds at planting with the remainder applied 35 days from planting, and application of 140 lb/acre K2O applied in equal amounts at planting and side-dress was cited for high yield results (Kidder et al., 1992).
The single K rate recommendation of 140 lb/acre K2O (Hochmuth and Hanlon, 2000) for soils with any M-1 tested K concentration, based on the above summarized tri-county research, was evaluated for potatoes grown in South Florida (Hochmuth et al., 1998). Five experiments were conducted in Homestead, Florida, in the winters of 1993-1994 (grower 1), 1994-1995, and 1995-1996 (growers 1 and 2). Potassium was applied from 0 to 200 lb/acre K2O in four of these experimental seasons and from 0 to 240 lb/acre K2O in a fifth experimental season. The soil extractant ammonium bicarbonate-diethylenetriamine pentacetic acid, AB-DTPA, was used due to the marl and rockland soils in this area to evaluate soil K concentrations. Soil nutrient concentrations determined with AB-DTPA extractant are not interpreted as soil concentrations determined with M-1 extractant.
Yields maximized with between 99 and 100% RYs in all of these experiments with K rates less than 140 lb/acre K2O (Hochmuth et al., 1998). Maximum yields occurred between 50 and 120 lb/acre K2O resulting in yields of 87, 193, 134, 239, and 146 cwt/acre. As with the tri-county experiments in northeastern Florida, yield responses to applied K fertilizer were not dependent upon soil-extracted K concentrations. A yield response to 120 lb/acre K2O resulted where the extracted soil K concentration was 60 ppm. However, no yield response resulted where the soil K concentration was 71 ppm. An increased soil-extracted K concentration of 122 ppm resulted in a quadratic yield response leveling off with 100 lb/acre K2O. Yield responses did not differ with K rates where soil-extracted K was 274 ppm and no discernible yield trend resulted to increased K rates where soil-extracted K was 787 ppm. The predictive capability of the AB-DTPA soil test, as with the M-1 soil test in North Florida, was not effective for K fertilization recommendation on marl and rockland soils and the 140 lb/acre K2O recommendation appeared sufficient for high yield on soils throughout the state. Whole leaf-tissue K concentrations were above the sufficiency range in all experiments.
Conditions Linked to Over-Fertilization with Potassium
Over-fertilization with K is cited in the literature as a factor for reduced specific gravity in potato and increased occurrence of potato soft rot in some experiments. Higher soil fertility generally results in increased water uptake by plants, which results in lower solids content and specific gravity of the tuber in potato plants (Hensel, 1967). Although potatoes with high specific gravity produce more potato chips, other quality characteristics such as crispness and color uniformity of chips, which are not affected by specific gravity, can be more important. Florida 'Sebago' potato was described as the favored chipping potato in Hastings since 1942 for these quality characteristics, despite the relatively low specific gravity (1.0696). Research on the effects of K sources K2SO4 or KCl on the specific gravity of potato was evaluated throughout six planting seasons from 1954 to1959 in Hastings (Myhre, 1959). In the last season, changes in specific gravity were evaluated using three K rates, 100, 200, or 300 lb/acre K2O applied in bands at planting or applied broadcast at 200 lb/acre K2O.
Generally potato plants fertilized with K2SO4-K resulted in tubers with higher specific gravity than those fertilized with KCl. Exceptions occurred in which higher specific gravity resulted from KCl fertilized plants, when excessive rainfall required midseason K side-dressing. Specific gravity increased through 200 lb/acre of band-applied K2O and decreased significantly with 300 lb/acre K2O. Other factors had an observed effect on specific gravity of 'Sebago' potato tubers, including soil moisture. Tubers grown further from the irrigation furrow to 55 feet had higher specific gravity than those grown 5 feet from the irrigation furrow (1.0635 to 1.0655, respectively). Broadcast fertilizer application resulted in tubers with increased specific gravity compared to tubers grown where K was applied in bands at 200 lb/acre K2O.
Specific gravity of potatoes decreased significantly (at 1% probability) in two of three seasons with increased K rate fertilization from 240 to 485 lb/acre K2O in Hastings research (Locascio et al., 1991; Locascio et al., 1992). Researchers sought to identify excess K fertilization as a factor in bacterial soft rot development in potato tubers. This disorder and other disorders of potato tubers can be worsened by Ca deficiency, which can occur in soils where K+, NH4+, and Na+ from dissolved fertilizer compete with Ca++ for uptake by the root. Florida potato production soils with typically low to moderate, 400 to 800 ppm (M-1), concentrations of Ca prompted spring 1984, 1985, and 1986 experiments with Ca and K in Hastings. Calcium was applied at rates of 0, 400, or 800 lb/acre in factorial combination with 240 or 485 lb/acre K2O. Fertilizers were formulated and applied in bands 4 inches below the bed surface and 4 inches to each side of the seed potato. Soil K concentrations extracted by M-1 soil test were medium each season.
Tuber decay responses to varying rates of K and Ca fertilizer varied with the cultivar, but rainfall had the greatest effect on tuber decay resulting in 49% disease severity in the wet 1984 season, compared to 11 and 4% in the drier seasons of 1985 and 1986, respectively. Calcium and K were moved downward through the soil during the wet season, resulting in less Ca accumulation in the tuber compared to the drier seasons where subsurface irrigation maintained soil fertility, increased tuber Ca and K accumulation, and decreased severity of bacterial soft rot. Variation among 'LaChipper', 'Superior', and 'Atlantic' in three seasons to Ca and K fertilization made a decay response difficult to assess.
Potassium Summary
The 1978 recommendation of 200 lb/acre K2O for irrigated mineral soils (Montelaro, 1978) was reduced in 1989 to 140 lb/acre for very low and low K soils, 70 lb/acre for medium soils, and 0 lb/acre for high and very high K soils based on M-1 soil test (Kidder et al., 1989). Potassium recommendations were revised further in 1995 (Hochmuth and Hanlon, 1995) to a blanket recommendation of 140 lb/acre K2O for soils testing from very low to very high in M-1 soil-extracted K in north Florida and for all soil K concentrations extracted with the AB-DTPA soil test in south Florida. Researchers cited inconsistent yield response to applied K regardless of soil K concentrations as reason for the revised K recommendation. Data on leaf-tissue K concentrations supported the revised recommendation with K concentrations often exceeding the sufficiency range with low rates of applied K. Such variability was likely due to the mobility of this nutrient in sandy soils. Yield responses to K rates greater than 140 lb/acre K2O were split. In some experiments, yields remained high through 500 lb/acre K2O, while in others, yields dropped as much as 50% with applied K rates through 300 lb/acre K2O (Figure 13). Potato yield responses to K fertilization from experiments, summarized in Figure 13, appeared to level off after 100 lb/acre K2O. Reduction in the specific gravity of potatoes, or decreased chipping quality, frequently resulted with higher rates of applied K. Favorable growing conditions expressed as the number of growing degree days (Rhue et al., 1986) often had a greater effect on potato yields than rates of applied K fertilizer.
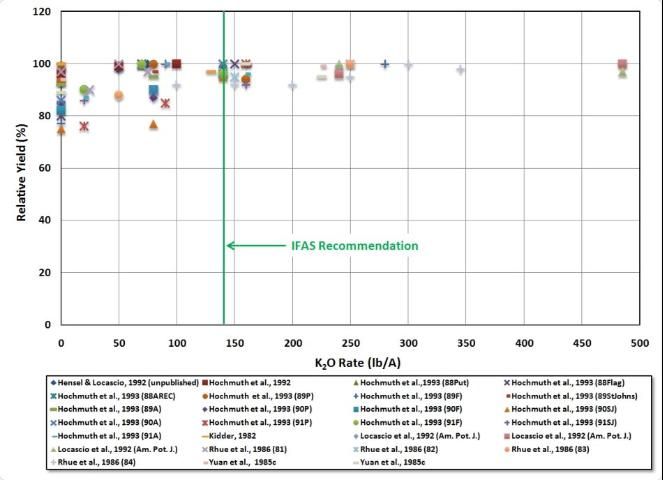
Since K leaches in sandy soils, total K application should be split into two applications with some K applied at planting or at cracking. Potato yield did not respond to K source on a soil testing medium in M-1 K. Yields were similar for soluble KNO3, KCl, and K2SO4, for a controlled-release (CR) KNO3, and for various mixtures of soluble and CR sources.
Summary
There has been a large amount of research conducted on potato nutrient and irrigation management, making potato one of the most actively researched crops in Florida for fertilizer management. Research throughout the past 70 years supports the current target recommendation of 200 lb/acre N for optimal potato yields with only sporadic yield increases slightly greater than 200 lb/acre N up to 225 lb/acre N. Excessive N sometimes reduced yield and specific gravity of potatoes. Many studies involving many varieties in several decades do not indicate any large varietal difference in N requirements. The variety 'Atlantic' has been used in the majority of fertilizer studies since the early 1980s through the present. Early research with controlled-release N sources SCU, IBDU, and a gel-infused urea-ammonium-nitrate did not increase yields compared to potatoes grown with soluble N sources. However, more recent studies with newer polymer-coated N sources showed N rates could be reduced to less than the current recommendation and that the combination of controlled-release fertilizers and reduced rates resulted in less N leaching. Studies are needed to follow N in the soil system as it relates to N and irrigation management. Additional large-scale demonstrations completed on commercial farms using recommended nutrient management programs are needed in all potato production areas. Phosphorus and K recommendations tested through experimentation appear to be on target for the needs of potato while minimizing impact on the environment. The mobility of K in the sandy Hastings-area soils and the reduced predictive capability of soil K tests, M-1 in the Hastings area and AB-DTPA in the Homestead area, led to abandonment of K testing in favor of a single target rate of K, 140 lb/acre K2O. Excessive K application often resulted in a reduction of specific gravity of potatoes. The application timing of mobile nutrients, such as N or K, was important for greatest yields. N fertilizer can be withheld until plant emergence. All N and K fertilizers need to be applied by 35 to 40 days after planting.
Literature Cited
Black, C. A. 1992. Soil fertility evaluation and control. Lewis Publishers, Boca Raton, FL. 746 pp.
Brown, J. R. 1987. Soil testing: sampling, correlation, calibration, and interpretation. SSSA Special Publ. No. 21. Soil Science Society of America, Inc. Madison, Wisc.
Chen, Z., and C. M. Hutchinson. 2008. Evaluation of alternative fertilizer programs in subsurface irrigated potato production. Proc. Fla. State Hort. Soc. 121:187–190.
Elkashif, M. E., S. J. Locascio, and D. R. Hensel. 1983. Isobutylidene diurea and sulfur-coated urea as N sources for potatoes. J. Amer. Soc. Hort. Sci. 108(4):523–526.
Florida Dept. of Agriculture and Consumer Services. 2007. Florida Agricultural Statistics - http://www.florida-agriculture.com/fass_activities.htm
Florida Dept. of Agriculture and Consumer Services. 2005. Water quality/quantity best management practices for Florida vegetable and agronomic crops. http://www.floridaagwaterpolicy.com/PDF/Bmps/Bmp_VeggieAgroCrops2005.pdf.
Florida Department of Environmental Protection. 2009. Lower St. Johns River Basin TMDL. FDEP. http://www.dep.state.fl.us/northeast/stjohns/TMDL/tmdl.htm.
Hensel, D. R. 1962a. Phosphorus fertilization of potatoes on Ona fine sand. Soil Crop Sci. Soc. Fla. Proc. 22:130–134.
Hensel, D. R. 1962b. Plastic mulching of potatoes. Soil Crop Sci. Soc. Fla. Proc. 22:232–238.
Hensel, D. R. 1964. Irrigation of potatoes at Hastings, Florida. Soil and Crop Sci. Soc. Fla. Proc. 24:105–110.
Hensel, D. R. 1967. Influence of surface mulches on growth of potatoes. Proc. Fla. State Hort. Soc. 80:186–193.
Hensel, D. R. 1968. Response of potatoes to mulching at different planting and harvesting dates. Proc. Fla. State Hort. Soc. 81:153–158.
Hensel, D. R., and S. J. Locascio. 1987. Effect of rates, form, and application date of nitrogen on growth of potatoes. Proc. Fla. State Hort. Soc. 100:203–205.
Hensel, D. R., and S. J. Locascio. 1992. Effect of potassium rate, K sources and fertilizer coatings on growth and development of potatoes. Unpublished.
Hochmuth, G. J. 1992a. Fertilizer management for drip-irrigated vegetables in Florida. HortTechnology 2:27–32.
Hochmuth, G. J. 1992b. Concepts and practices for improving nitrogen management for vegetables. HortTechnology. 2 (1):121–125.
Hochmuth, G., 1994. Yield and leaf nutrient concentrations of potato and sweet corn grown with various fertilizer programs at Suwannee farms, O'Brien, Florida. Fla. Coop. Ext. Serv. Misc. report, Suwannee Valley AREC 94–07.
Hochmuth, G. J. 2000. Management of nutrients in vegetable production systems in Florida. Soil and Crop Sci. Soc. Fla. Proc. 59:11–13.
Hochmuth, G., and K. Cordasco. 2000. Summary of N, P, and K research on potato in Florida. Fla. Coop. Ext. Serv. Fact Sheet HS 756. https://edis.ifas.ufl.edu/CV233.
Hochmuth. G. J. and E. A. Hanlon, 2000. IFAS standardized fertilization recommendations for vegetable crops. Circular 1152. University of Florida, Institute of Food and Agricultural Sciences, Gainesville, FL.
Hochmuth, G. J., E. A. Hanlon, and B. Hochmuth. 1992. N and K fertilization of red potatoes at Live Oak, FL, Spring, 1988. Research Report, Suwannee Valley AREC, 92–30, 10pp.
Hochmuth, G. J., E. A. Hanlon, S. O Hair, J. Carranza, and M. Lamberts. 1998. Report to S. Fla. Water Mgt. Dist.
Hochmuth, G. J., E. A. Hanlon, B. Hochmuth, G. Kidder, and D. R. Hensel. 1993a. Field fertility research with P and K for vegetables-interpretations and recommendations. Soil and Crop Sci. Soc. Fla. Proc. 52:95–101.
Hochmuth, G. J., E. A. Hanlon, G. Kidder, D. R. Hensel, W. A. Tilton, J. D. Dilbeck, and D. E. Schrader. 1993b. Fertilization demonstrations for the tri-county potato production area of northeast Florida. Proc. Fla. State Hort. Soc. 106:190–198.
Hochmuth, G. J., D. Maynard, C. Vavrina, E. A. Hanlon, and E. H. Simonne. 2009. Plant tissue analysis and interpretation for vegetable crops in Florida. Fla. Coop. Ext. Serv. Fact Sheet HS 964. https://edis.ifas.ufl.edu/EP081.
Hochmuth, G., and J. Jones. 2004. Timing of initial Nitrogen application did not affect potato yield. Proc. Fla. State Hort. Soc. 117:52–54.
Hochmuth, G. J., D. P. Weingartner, C. Hutchinson, W. A. Tilton, and D. Jesseman. 2002. Potato yield and tuber quality did not respond to phosphorus fertilization of soils testing high in phosphorus content. HortTechnology 12:420–423.
Hutchinson, C. M. 2004. Controlled Release Fertilizer potato production system for Florida. Proc. Fla. State Hort. Soc. 117:76–78.
Hutchinson, C. M. 2005. Influence of a Controlled Release Nitrogen Fertilizer program on potato tuber yield and quality. Proc. Meeting Physiol. Section EAPR Eds.: R. Casa and R. Viola, Acta Hort. 684.
Hutchinson, C. M., and R. S. Mylavarapu. 2003. Evaluation of Nitrogen best management practices for potato production in Northeast Florida. Proc. XXIV IHC – Potatoes – Healthy Food for Humanity. Ed. R. Y. Yada. ACTA Hortic. 619:279–283.
Hutchinson, C. M., and E. H. Simonne. 2003. Controlled-release fertilizer opportunities and costs for potato production in Florida. Fla. Coop. Ext. Serv. Fact Sheet HS941, https://edis.ifas.ufl.edu/hs187.
Hutchinson, C. M., E. H. Simonne, G. J. Hochmuth, W. M. Stall, S. M. Olson, S. E. Webb, T. G. Taylor, and S. A. Smith. 2008. Potato production in Florida. P.353–367. IN: S. M. Olson and E. H. Simonne (eds.). Vegetable production handbook for Florida, 2007-2008. Fla. Coop. Ext. Service.
Hutchinson, C. M., E. Simonne, P. Solano, J. Meldrun, and P. Livingston-Way. 2003. Testing of controlled release fertilizer programs for seep irrigated Irish potato production. Journal of Plant Nutrition, Vol. 26, No. 9, pp.1709–1723.
Kidder, G. 1982. Field testing of fertilization recommendations in 1980 and 1981. Soil Crop Sci. Soc. Fla. Proc. 41:192–195.
Kidder, G., E. A. Hanlon, and G. J. Hochmuth. 1989. IFAS standardized fertilization recommendations for vegetable crops. Fla. Coop. Ext. Serv. Spec. Ser. SS-SOS-907.
Kidder, G., G. J. Hochmuth, D. R. Hensel, E. A. Hanlon, W. A. Tilton, J. D. Dilbeck, and D. E. Schrader. 1992. Horticulturally and environmentally sound fertilization of Hastings area potatoes. Fla. Coop. Ext. Serv. Brochure.
Livingston-Way, P. 2000. Tri-County agricultural area water quality protection cost share program applicant's handbook. St. Johns River Water Management District, Palatka, FL.
Locascio, S. J., and H. L. Breland. 1963. Irish potato yield and leaf composition as affected by dolomite and phosphorus. Soil Crop Sci. Soc. Fla. Proc. 23:95–99.
Locascio, S. J., J. A. Bartz, and D. P. Weingartner. 1991. Potato yield and soft-rot potential as influenced by calcium and potassium fertilization. Proc. Fla. State Hort. Soc. 104:248–253.
Locascio, S. J., J. A. Bartz, and D. P. Weingartner. 1992. Calcium and potassium fertilization of potatoes grown in North Florida I. Effects on potato yield and tissue Ca and K concentrations. Amer. Potato J. 69:95–104.
Locascio, S. J., and D. R. Hensel. 1990. Potato yield as influenced by gelling a liquid fertilizer. Proc. Fla. State Hort. Soc.103:108–110.
Locascio, S. J., V. F. Nettles, and J. R. Neller. 1961. The effect of sawdust incorporation in a soil on growth of Irish potatoes and sweet corn. Proc. Fla. State Hort. Soc. 74:197–201.
Locascio, S. J., and R. D. Rhue. 1990. Phosphorus and micronutrient sources for potato. Am. Potato Jour. 67: 217–226.
Magley, W., and D. Joyner. 2008. Total maximum daily load for nutrients for the Lower St. Johns River. Fla. Dept. Env. Protection, Final Report, July, 2008. https://floridadep.gov/sites/default/files/lower-stjohns-nutrients.pdf. Accessed February 16, 2023.
Martin, H. W., D. A. Graetz, S. J. Locascio, and D. R. Hensel. 1993. Nitrification inhibitor influences on potato. Agron. J. 85:651–655.
McCubbin, E. N. 1956. Results of different seeding and fertilizer rates for potatoes at Hastings. Proc. Fla. State Hort. Soc. 69:259–261.
McCubbin, E. N., D. L. Myhre, and G. M. Volk. 1955. Effects of side-dressing and foliar-nutritional spray on yield of potatoes at Hastings, Florida. Proc. Fla. State Hort. Soc. 68:216–220.
Medina, S., and J. VanSickle. 1999. An economic analysis of best management practices (BMPs) for potatoes grown in the Tri-County Agricultural Area of north Florida. Report to the St. Johns Water Management District, contract #93G228.
Montelaro, J. 1978. Commercial vegetable fertilization guide. Fla. Coop. Ext. Serv. Circ. 225-B.
Munoz-Arboleda, F. 2004. Improving nitrogen management in potatoes through crop rotation and enhanced uptake. Ph.D. Diss. Univ. Fla., Gainesville, Florida.
Munoz-Arboleda, F., and C. M. Hutchinson. 2006. Soil moisture in the potato root zone under subsurface irrigation. Proc. Fla. Hort. Soc. 119:218–220.
Munoz-Arboleda, F., R. S. Mylavarapu, and C. M. Hutchinson. 2005. Environmentally responsible potato production systems: a review. Jour. Plant Nutrition. 28:1287–1309.
Munoz-Arboleda, F., R. S. Mylavarapu, and C. M. Hutchinson. 2008. Nitrate-Nitrogen concentrations in the perched ground water under subsurface-irrigated potato cropping systems. J. Environ. Qual. 37:387–394.
Myhre, D. L. 1959. Factors affecting specific gravity of potatoes. Proc. Fla. State Hort. Soc. 72:202–204.
Myhre, D. L., E. N. McCubbin, and G. M. Volk. 1955. Effects of irrigation, fertilization and seeding rates on potato yields in 1955 at Hastings, Florida. Proc. Fla. State Hort. Soc. 68:233–235.
Mylavarapu, R. S. 2009. UF/IFAS extension soil testing laboratory (ESTL) analytical procedures and training manual. Fla. Coop. Ext. Serv. Circ 1248. https://doi.org/10.32473/edis-ss312-2009. Accessed February 16, 2023.
Pack, J. M., C. M. Hutchinson and E. H. Simonne 2006. Evaluation of controlled-release fertilizers for northeast Florida chip potato production. Journal of Plant Nutrition, 29:1301–1313, 2006.
Rhue, R. D., and G. Kidder. 1984. Procedures used by the IFAS extension soil testing laboratory and interpretation of results. Fla. Coop. Ext. Serv. Circ. 596.
Rhue, R. D., D. R. Hensel, and G. Kidder. 1986. Effect of K fertilization on yield and leaf nutrient concentrations of potatoes grown on a sandy soil. Amer. Potato J. 63:665–681.
Rhue, R. D., D. R. Hensel, T. L. Yuan, and W. K. Robertson. 1981a. Ammonium orthophosphate and ammonium polyphosphate as sources of phosphorus for potatoes. Soil Sci. Soc. of Amer. J. 45(6):1229–1233.
Rhue, R. D., D. R. Hensel, T. L. Yuan, and W. K. Robertson. 1981b. Response of potatoes to soil and fertilizer phosphorus in northeast Florida. Soil Crop Sci. Soc. Fla. Proc. 40:58–61.
Rogers, J. S., D. R. Hensel, and K. L. Campbell. 1975. Subsurface drainage and irrigation for potatoes. Soil and Crop Sci. Soc. Fla. Proc. 34:16–17.
Simonne, E. H., and C. M. Hutchinson. 2005. Controlled-release fertilizers for vegetable production in the era of best management practices: Teaching new tricks to an old dog. HortTechnology 15:36–46.
Smajstrla, A. G., B. J. Boman, G. A. Clark, D. Z. Haman, D. S. Harrison, F. T. Izuno, D. J. Pitts, and F. S. Zazueta. 2002. Efficiencies of Florida agricultural irrigation systems. Fla. Coop. Ext. Serv. Bull. 247. https://ufdc.ufl.edu/IR00004382/00001/pdf. Accessed February 16, 2023.
Stall, W. M., and M. Sherman. 1984. Potato production in Florida. Circ. 118. Fla. Coop. Ext. Serv.
Volk, G. M., and N. Gammon, Jr. 1950. Effect of low nitrate nitrogen on growth of potatoes. Proc. Fla. State Hort. Soc. 63:112–115.
Weingartner, D. P., G. J. Hochmuth, W. A. Tilton, and D. Jesseman. 1999. BMP demonstration trials, 1999. Hasting AREC Research Report.
Worthington, C. M., and C. M. Hutchinson. 2008. Fate and movement of nitrogen and phosphorus in Deep Creek in the lower St. Johns River Basin of northeast Florida. Proc. Fla. State Hort. Soc. 121:183–186.
Yuan, T. L., D. R. Hensel, R. S. Mansell, and R. D. Rhue. 1985a. Yield and nutrient content of potato on a sandy Humaquept with high levels of accumulated Ca and P. Soil Crop Sci. Soc. Fla. Proc. 44:68–71.
Yuan, T. L., D. R. Hensel, R. D. Rhue, and W. K. Robertson. 1985b. Nutrient status of a sandy Humaquept under long-term potato cultivation. Soil Crop Sci. Soc. Fla. Proc. 44:93–97.
Yuan, T. L., D. R. Hensel, and R. S. Mansell. 1985c. Yield responses of potato to N and K fertilization in soil with high accumulated P. Soil Crop Sci. Soc. Fla. Proc. 44:107–110.