Plant Tissue Analysis and Interpretation for Vegetable Crops in Florida
Introduction
Improved fertilizer management for vegetables is important in view of today's need to reduce production costs, conserve natural resources, and minimize possible negative environmental impacts. These goals can be achieved through optimum management of the fertilizer applied. Understanding the crop nutrient requirements and using soil testing to predict fertilizer needs are keys to fertilizer management efficiency.
Plant tissue testing is another tool for use in achieving a high degree of precision in fertilizer management. Timely tissue testing can help diagnose suspected nutrient problems or can simply assist in learning more about fertilizer management efficiency.
This guide is provided to assist vegetable growers, UF/IFAS Extension personnel, and consultants in conducting a meaningful plant tissue testing program. Guidelines are provided for collecting samples, proper handling of the sample, and choosing an analytical lab. Information is also presented on basic plant nutrition so that the reader understands the nutrient requirements of each vegetable crop and the process of identifying nutrient deficiencies.
The final section of the guide presents the deficiency, sufficiency, and toxicity ranges for plant nutrient concentrations. This is the interpretation portion. Values presented in the tables have been drawn from research from many areas of the country with emphasis on research conducted in Florida. Missing values in the tables indicate areas of research need. The final section of the guide also presents recommendations for nutrient deficiency correction.
Plant Nutrition
Essential Elements
Plants require light, water, minerals, oxygen, carbon dioxide, and a suitable temperature to grow. These absolute growth requirements must be available within appropriate ranges and in balance with others for optimum growth to occur.
A total of 17 elements are known to be required for plants to grow and reproduce normally. The elements are carbon (C), hydrogen (H), oxygen (O), nitrogen (N), phosphorus (P), potassium (K), calcium (Ca), magnesium (Mg), sulfur (S), iron (Fe), boron (B), manganese (Mn), copper (Cu), zinc (Zn), molybdenum (Mo), chlorine (Cl) and nickel (Ni).
The atmosphere provides C and O, and H is provided by water. Together, these three elements are combined into simple organic compounds during the process of photosynthesis. The other 14 elements are supplied mostly from the soil, including native soil fertility, residual lime and fertilizer, or from current lime and fertilizer applications. Other less important sources of plant nutrients are well water (Ca, Mg, S, Fe) and the atmospheric deposition (S and N).
The macronutrients (N, P, K, Ca, Mg, S) are those found in comparatively high concentrations in plants and are measured in percent (%). Micronutrients (Fe, B, Mn, Cu, Zn, Mo, Cl) are present in comparatively minute concentrations in plants and are measured in parts per million (ppm).
Roles of Essential Elements in Plant Growth
Each of the essential elements has at least one specifically defined role in plant growth so that plants fail to grow and reproduce normally in the absence of that element. However, most of the essential elements have several functions in the plant. A basic summary of some of these functions follows:
Carbon, from carbon dioxide (CO2) in the atmosphere, is assimilated by plants in the photosynthetic process. It is a component of organic compounds such as sugars, proteins, and organic acids. These compounds are used in structural components, enzymatic reactions, and genetic material, among others. The process of respiration degrades organic compounds to provide energy for various plant metabolic processes.
Oxygen, derived from CO2, also is a part of organic compounds such as simple sugars. Atmospheric oxygen is necessary for all oxygen-requiring reactions in plants including nutrient uptake by roots.
Hydrogen derived from water (H2O) also is incorporated into organic compounds in the photosynthetic process. Hydrogen ions are involved in electrochemical reactions and maintain electrical charge balances across all membranes.
Phosphorus is used in several energy transfer compounds in plants. A very important function for P is its role in nucleic acids, the building blocks for the genetic code material in plant cells.
Potassium plays a major role as an activator in many enzymatic reactions in the plant. Many enzymes responsible for cellular reactions require K as a co-factor. Another role for K in plants occurs in special leaf cells called guard cells found around the stomata. By regulating the turgor pressure in the guard cells, the degree of opening of the stomata is controlled and thus the level of gas and water vapor exchange through the stomata is regulated. Turgor is largely controlled by K movement in and out of guard cells.
Nitrogen is found in many compounds including chlorophyll (the green pigment in plants), amino acids, proteins, and nucleic acids. A large part of the plant body is composed of N-containing compounds.
Sulfur is a component of sulfur-containing amino acids such as methionine. Sulfur also is contained in the sulfhydryl group of certain enzymes.
Calcium is a component of calcium pectate, a constituent of cell walls. In addition, Ca is a co-factor of certain enzymatic reactions. Recently, it has been determined that Ca is involved in the intimate regulation of cell processes mediated by a molecule called calmodulin.
Magnesium plays an important role in plant cells since it appears in the center of the chlorophyll molecule. Certain enzymatic reactions require Mg as a co-factor.
Iron is used in the biochemical reactions that form chlorophyll and is a part of one of the enzymes that is responsible for the reduction of nitrate-N to ammoniacal-N. Other enzyme systems such as catalase and peroxidase also require Fe.
Boron functions in the plant are still not well understood. Boron seems to be important for normal meristem development in young plant parts, such as root tips.
Manganese functions in several enzymatic reactions that involve the energy compound adenosine triphosphate (ATP). Manganese also activates several enzymes and is involved in the processes of the electron transport system in photosynthesis.
Copper is a constituent of a protein, plastocyanin, involved in electron transport in chloroplasts, and copper is part of several enzymes, called oxidases.
Zinc is involved in the activation of several enzymes in the plant and is required for the synthesis of indoleacetic acid, a plant growth regulator.
Molybdenum is a constituent of two enzymes involved in N metabolism. The most important of these is nitrate reductase, the enzyme involved in the reduction of nitrate-N to ammoniacal-N.
Chlorine plays a possible role in photosynthesis and might function as a counter ion for K fluxes involved in cell turgor.
Nickel is now recognized by plant scientists as an essential element for plants. It is involved in the enzyme urease and is a part of several other enzymes involved in plant metabolism.
Mobility of Essential Elements within the Plant
Approximately 80% of all nutrients absorbed by roots are translocated to the shoots. When nutrient supply is abundant, they are delivered directly to the shoots often within minutes of absorption. Accordingly, plants may absorb and accumulate essential elements in far greater quantities than are necessary for immediate use. These accumulated elements are available for use later in the plant life cycle when demands are high for fruit production and/or when nutrient supply from the soil is restricted. The ability of an element to move from one plant part to another is called mobility and the process is known as retranslocation. The mobility of the essential elements in plants is shown in Table 1.
The mobility of an element influences the location where deficiency symptoms (see the following section) are likely to be observed on the plant. For example, Mg deficiency symptoms occur on the oldest, generally lower leaves, because Mg is retranslocated to the younger leaves of the plant. Conversely, Ca deficiencies occur at the growing point or in storage organs like roots and fruits because Ca, being immobile, is not retranslocated to these sites during Ca stress conditions.
Nutrient Deficiency Symptoms
Vegetable plants exhibit deficiency symptoms that are characteristic for each element, and are, therefore useful for diagnostic purposes. However, in many cases, the symptoms may be masked by symptoms of other nutritional disorders, those caused by unfavorable environment, or stress caused by plant pests. In these situations, plant tissue analysis provides useful information to complement and confirm visual diagnosis. Nutritional disorders of vegetables rarely occur in well managed crops. The general symptoms associated with deficiencies and excesses of the essential elements follow:
Nitrogen is absorbed as NH4+ and NO3 -. It is a mobile element in the plant and deficiency symptoms therefore show up first on the lower leaves. Symptoms consist of a general yellowing (chlorosis) of the leaves. On tomatoes, there might be some red coloration to the petioles and leaf veins. If the problem persists, lower leaves will drop from the plant.
Healthy plant leaves contain between 2.0 and 5.0% N on a dry weight basis. Deficiencies of N show up most often where errors are made in fertilizer management resulting in insufficient N supply to the crops. More often in commercial vegetable production, there is a problem from excess N application. Plants receiving excess N usually are lush and tender with larger and darker-green leaves. Excess N (especially in warm and sunny conditions) can lead to "bullish" tomato plants. These plants produce thick, leathery leaves that curl under in dramatic fashion producing compact growth.
Phosphorus is typically absorbed as H2PO4- by an active (energy-requiring) process. P is very mobile in the plant. Deficiencies therefore show up on the older leaves of the plant because P is translocated out of these leaves to satisfy the needs of new growth. P deficiency shows up as stunting and a reddish coloration resulting from enhanced display of anthocyanin color pigments. Deficient leaves will have only about 0.1% P in the dry matter. Normal, most-recently matured leaves of most vegetables, will contain 0.25 to 0.6% P on a dry weight basis. Excess P in the root zone can result in reduced plant growth probably as a result of P retarding the uptake of Zn, Fe, and Cu.
Potassium is absorbed in large quantities by an active uptake process. Once in the plant, K is very mobile and is transported to young tissues rapidly. Deficiency symptoms for K show up first on lower leaves as flecking or mottling on the leaf margins. Prolonged deficiency results in necrosis along the leaf margins and the plants can become slightly wilted. Deficient plant leaves usually contain less than 1.5% K. Deficiencies of K lead to blotchy ripening of tomatoes where fruits fail to produce normal red color in some areas on the fruit.
Calcium, unlike most elements, is absorbed and transported by a passive mechanism. The transpiration process of plants is important in the transport of Ca. Once in the plant, Ca moves toward areas of high transpiration rate, such as rapidly expanding leaves.
Most of the uptake of Ca occurs in a region on the root just behind the root tip. This has practical importance for vegetable culture because it means that growers must keep healthy root systems with numerous actively growing root tips. Root diseases and nematodes may severely limit Ca uptake by the plant.
Calcium is immobile in the plant, therefore, deficiency symptoms show up first on the new growth. Deficiencies of Ca cause necrosis of new leaves or lead to curled, contorted growth. Examples of this are tipburn of lettuce and cole crops. Blossom-end rot of tomato also is a calcium-deficiency related disorder. Cells of the tomato fruit deprived of Ca break down causing the well-known dark area on the tomato fruit. Sometimes this breakdown can occur just inside the skin so that small darkened hard spots form on the inside of the tomato while the outside appears normal. On other occasions, the lesion on the outside of the fruit is sunken or simply consists of a darkening of tissue around the blossom area.
Since Ca movement in the plant is related to transpiration, environmental conditions that affect transpiration also affect Ca movement. Periods of high humidity can lead to tipburn of lettuce because the leaves are not transpiring rapidly enough to move adequate Ca to the leaf extremities.
Calcium concentrations in healthy, most-recently matured leaves will be from about 0.6 to 5.0%. Deficiencies, however, can occur temporarily given certain environmental conditions as previously discussed. Therefore, it is important to consider irrigation in the overall Ca fertilization program.
Magnesium is absorbed by the plant in lower quantities than Ca. Unlike Ca, Mg is highly mobile in the plant and deficiencies first appear on the lower leaves. Deficiency symptoms consist of an interveinal chlorosis, which can lead to necrosis of the affected areas. On tomato leaves, advanced Mg deficiency leads to a mild purpling of the affected areas.
Magnesium is usually found in concentrations of 0.2 to 0.8% in normal leaves. Conditions that lead to deficiency are usually related to poorly designed fertilizer programs that supply too little Mg, or when Ca and/or K compete with Mg for uptake.
Sulfur is absorbed mainly in the form of sulfate (SO4-2) by a mechanism that is not well understood. Sulfur is somewhat mobile in the plant so deficiency symptoms are fairly evenly distributed on the plant but mostly on the upper leaves. Deficiency symptoms consist of a general yellowing of the leaves. Deficiencies of N and S appear somewhat similar but N deficiency occurs on the lower leaves whereas S deficiency occurs in the upper part of the plant.
Plant leaves usually contain between 0.2 and 0.5% S on a dry weight basis. This range is similar to that for P. Plants can generally tolerate quite high concentrations of S in the growing media. This is one reason for the wide use of S-containing materials to supply nutrients such as Mg and the micronutrients, and explains why S deficiency is not very common in vegetable crops.
Iron is absorbed by an active process as Fe2+ or as iron chelates, which are organic molecules containing iron sequestered within the molecule. Uptake of Fe is highly dependent on the Fe form and adequate uptake depends on the ability of the root to reduce the pH nearby and reduce Fe3+ to Fe2+ for uptake. Iron chelates are soluble and aid in keeping Fe in solution for uptake. The uptake of the whole chelate molecule is low and usually Fe is removed from the chelate before uptake.
Iron is not mobile in plants and symptoms appear on the new leaves first. Symptoms consist of interveinal chlorosis that may progress to a bleaching and necrosis of the affected leaves. Usually, the chlorosis begins on the lower part of the leaflets and not at the tips. Normal leaves contain 30 to 150 ppm Fe on a dry-weight basis.
Conditions that lead to Fe deficiency are inadequate concentrations of Fe in the soil solution or basic soil conditions (pH above 7.0). Fe deficiency is corrected by adding Fe to the fertilizer or by foliar sprays of Fe. Usually one or two sprays of 0.5 ppm Fe solution will correct a temporary Fe deficiency.
Manganese is absorbed as Mn2+ ions and uptake is affected by other cations such as Ca and Mg. Manganese is relatively immobile in the plant and symptoms of deficiency first appear on the upper leaves.
Deficiency of Mn resembles that of Mg, however Mn deficiency appears on the upper leaves of the plant. Manganese deficiency consists of interveinal chlorosis; however, the chlorosis is more speckled in appearance compared to Mg deficiency. Manganese deficiency also slightly resembles Fe deficiency of tomato however Mn deficiency appears as chlorotic speckling over most of the leaf while Fe deficiency usually appears first on the lower part of the leaflets.
Critical concentrations of Mn in leaves ranges from 20 to 100 ppm for most plants. High levels of Mn can be toxic to plants. Toxicity appears as marginal leaf necrosis in many plants. Concentrations of Mn on the order of 500 to 800 ppm can result in toxicity in many crops. Excess Mn in the soil solution can reduce uptake of Fe by the plant.
Situations that lead to deficiency are mostly related to inadequate Mn supply in the soil solution, from basic soil conditions, or to competition effects of other ions. Toxicity can occur from excess Mn supply especially when plants are in acidic soil. Solubility of Mn in the soil solution is increased by low pH.
Zinc uptake is thought to be by an active process and can be negatively affected by high concentrations of P in the media. Zinc is not highly mobile in plants. Deficiency of Zn results in young leaves with interveinal chlorosis. Sometimes Zn deficiency will lead to plants with shortened internodes.
Healthy leaves contain about 25 to 150 ppm Zn. High levels of Zn can lead to toxicity where root growth is reduced and leaves are small and chlorotic. Zinc deficiency may occur in cold, wet soils, or in soil with a very high pH where Zn is rendered unavailable to the plant.
Copper is absorbed by plants in very small quantities. The uptake process appears to be an active process and it is adversely affected by high Zn concentrations. Copper is not highly mobile in plants but some Cu can be translocated from older to newer leaves. The normal level of Cu in plants is on the order of 4 to 20 ppm.
Copper deficiency on young leaves leads to chlorosis and some elongation of the leaves. Excess Cu, especially in acidic soil may be toxic to plants.
Molybdenum is absorbed as molybdate (MoO4-2) and the uptake can be suppressed by sulfate. Normal tissue concentrations of Mo are usually less than 1 ppm.
A deficiency of Mo first appears on leaves that are intermediate in age and older. The leaves become chlorotic and the margins roll. Unlike other micronutrients, Mo deficiency occurs in acidic soil conditions.
Boron uptake by plants is not well understood. Boron is not mobile in the plant and seems to have many uptake and transport characteristics in common with Ca.
Boron deficiency affects the young growing points first, e.g., buds, leaf tips and margins, and root tips. Buds develop necrotic areas and leaf tips become chlorotic and eventually die. Tomato leaves and stems become brittle. Healthy leaves contain 20 to 100 ppm B; levels higher than 150 ppm may lead to toxicity. Cole crops, beets, and celery have rather high B requirements, otherwise only small amounts of B are needed by plants and supplying excessive B from fertilizer or from foliar sprays can lead to toxicity.
Chlorine is supplied for plant nutrition as the chloride ion and is required in very small amounts for normal plant growth. Chloride is involved in photosynthesis and functions as a counter-ion in maintaining turgor pressure in cells. Chlorine deficiency symptoms are not common but include wilting. The chloride ion is very common in the environment and is often found as a constituent in fertilizers; therefore, deficiency symptoms are rare. High concentrations of chloride in the nutrient solution can be toxic to plants in hydroponic culture.
Nickel is required in small amounts by plants, 0.5 to 5.0 ppm Ni. Nickel is common in soil, and truly deficient soils have not be found. Deficiency symptoms include chlorosis similar to that of iron deficiency. Nickel deficiency also can be similar to zinc deficiency. These similarities in deficiencies make it difficult to diagnose true Ni deficiency in plants. A buildup of urea in leaf tips may occur in Ni-deficient plants.
Key to Nutritional Disorders of Vegetable Crops
The key in Table 2 can be used to assist in diagnosis of visual symptoms of nutrient disorders. Color photographs, available in many books (see general reference list at the end of this publication) may be useful in conjunction with the key.
Critical Concentrations
As reported in the section on nutrient deficiency symptoms, there is a general concentration range for each essential element that results in normal plant growth. This is called the adequate or sufficient nutritional concentration range (Figure 1). Plant growth remains relatively constant within the range of concentrations found in the zone of sufficiency.
The so-called critical concentration occurs at the point where growth is reduced 10% because of a shortage of the element in question. The critical concentration is in the transition zone, which is the borderline between elemental sufficiency and deficiency. Critical concentrations for an element can be different depending on stage of growth and plant part used for the reference tissue.
The zone of sufficiency (level part of the graph) is the area where an increase in tissue nutrient concentration is not accompanied by an increase in growth (Figure 1). This is the range in nutrient concentrations in which the grower should attempt to control the fertilizer program. The objective is to maintain tissue nutrient concentrations on the lower side of the range with good fertilization techniques. Managing plant nutrient concentrations on the right of the zone indicates over fertilization and resulting luxury consumption of nutrients by the plant.
The deficient zone occurs at tissue elemental concentrations lower than those in the transition zone and is accompanied by a drastic restriction in growth. Plants show deficiency symptoms as the nutrient concentration falls within this zone. This is the vertical portion of the curve (Figure 1).
At the other end of the scale is the toxicity zone where tissue elemental concentrations are greater than those in the adequate zone. A gradual decrease in plant growth occurs in the toxicity zone. As the tissue concentration rises further, toxicity symptoms, often necrosis, begins (Figure 1).
The curve shown in Figure 1 is obtained by growing plants at a wide range of concentrations of the element being studied. Meanwhile, other nutrients and factors influencing growth are held constant so that changes in growth can be attributed solely to the nutrient being studied. Either greenhouse or field experiments may be designed to generate the data necessary to develop the relationship between plant growth and tissue concentrations of a particular element.
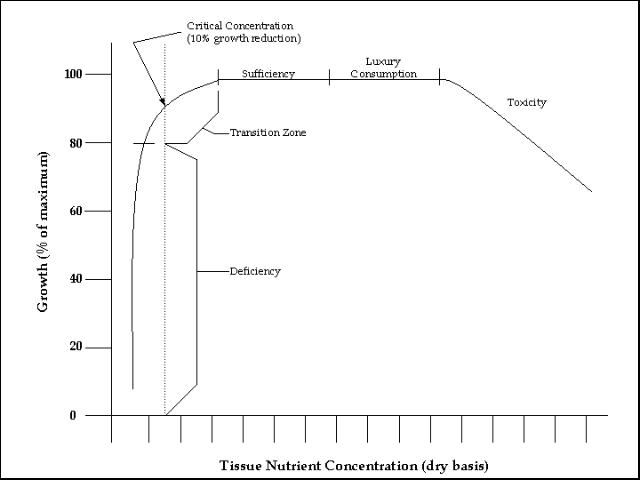
Application of Plant Analysis
Plant analysis assists in diagnosing nutritional problems or potential problems in the crop from which the samples are taken, i.e., the current crop. Potential problems can be circumvented, particularly if they are discovered early in the crop (before bloom) cycle by routine leaf analyses. For example, young cabbage plants that appear normal might have a very low N concentrations for that stage of growth. When checking fertilizer application records, it is found that an error was made, and only 1/10 of the intended rate was applied. Additional N can be applied and the crop can be saved, whereas if symptoms of N deficiency had developed before diagnosis, the crop may have been lost or there may have been a substantial yield reduction. With micro-irrigated or fertigated (drip) crops, the nutritional status of the crop can be monitored continuously, and fertigation adjustments can be made as needed.
Plant analysis results also have application for fertilizer management of the same crop grown in subsequent seasons. Fertilizer rates can be increased or decreased based on tissue test results and yields of previous crops. Given certain conditions, plant analysis results can be used to manage timing of supplemental sidedress or topdress fertilizer applications.
Results of plant tissue analysis along with results of soil analysis provide useful tools for the grower in managing the rate and timing of fertilizer applications for vegetables. However, each has limitations and they should not be used for purposes not intended.
Tissue testing is not recommended if the crop has received foliar sprays containing nutrients, especially micronutrients. There is no way to completely remove residues from leaf surfaces and these residues result in higher test results than actually in the plant tissue.
Sample Collection, Preparation, and Handling
Why Sample
There are two main reasons to test plant tissue for nutrient status. The first reason is to monitor the nutrient within the plants during the growing season. This technique is a good management strategy so long as the grower has a means of regulating nutrition in field conditions, for example, addition of nutrients through the micro irrigation system.
The second reason for tissue testing is to diagnose a suspected nutritional deficiency or toxicity. This diagnostic sampling is usually only done after a problem has been detected. In the case of deficiencies, the sampling should only be undertaken if the grower has enough time to apply extra fertilization AND the addition will actually enhance production. Too often, supplemental fertilization at the end of the season does not result in higher production, but only in greener foliage. With toxicities, information obtained on the current stressed crop can only be used to make management decisions that may benefit subsequent crops. For example, diagnosis of copper toxicity can only be treated by liming the field for the next crop.
The most frequent use of leaf tissue analysis is to diagnose a suspected nutrient deficiency. It is best to perform this analysis as soon as possible after the symptoms are evident. Once a deficiency manifests itself, the optimum yield may have already been lost. Losing the market window in shortseason crops due to a nutrient deficiency is devastating. The loss of market value due to poor leaf color in greens, for example, is also a consideration. Therefore, routine tissue sampling and analysis at the proper time(s) in the season can pay dividends for the grower.
When to Sample
A grower wishing to develop a routine program of tissue sampling to ensure proper nutrition for his or her crop throughout its growth cycle should begin shortly after the crop emerges from the soil (first true leaf) and continue at weekly or biweekly intervals. By means of a routine sampling and analysis program, the grower can fine-tune his fertilization program. Tissue analysis can serve as an indicator as to which nutrients are in adequate, deficient, or high concentrations. If a grower believes the nutritional status of his crop is satisfactory, he may benefit from a single sample taken just before fruit set and perhaps a second sample during mid-production. These samples would bracket that period when a deficiency would be most detrimental to optimum yield.
For routine sampling, a 'reference' tissue (most often leaves) is used to index plant nutritional status. Samples are collected on the basis of physiological age of the plant (not on calendar date) such as prebloom, tasseling, midgrowth, or heading.
What to Sample
There are several types of vegetable plant reference tissues including petiole, leaf, but rarely fruits. Some work has been done with vegetable plant petioles for nitrates in greenhouse crops and some field vegetable crops, but the standard vegetable reference tissue is the leaf. It is essential to use the same plant part as the one used to develop the interpretative data.
It is not practical to harvest and prepare entire plants for chemical analysis. Therefore, a plant part is used for convenience. However, it is essential that the plant part selected for chemical analysis accurately represents the nutritional status of the plant during its entire life cycle. For many vegetable crops, the most-recently-matured leaf (MRML) provides the most sensitive indicator of the nutritional status of the plant, sometimes only the petiole of this leaf is used for plant analysis. Specific plant parts for sampling each vegetable crop are specified in the section on sampling.
For most crops, and for many nutrients, mature, physiologically active leaves should be sampled. This is often referred to as "the most-recently-matured leaf" (MRML) including the blade and its petiole. The MRML is the leaf that has turned from a light-green juvenile color to a darker-green color and has reached full size. The exception to the rule of the MRML is the analysis of Ca, Cu, B, and S, which are relatively immobile in the plant. Therefore, an analysis of the mature leaves in this case may not reveal the Ca, B, Cu, or S deficiency in the younger leaves. When a nutrient deficiency of this nature is suspected, young (not fully expanded) leaf tissue is needed for analysis.
How to Sample
The sample is a whole leaf sample and it should not contain any root or stem material. For sweet corn or onions, the leaf is removed just above the attachment point to the stalk or bulb. For compound leaves (carrots, peas, tomatoes, etc.), the whole leaf includes the main petiole, all the leaflets and their petioliules. For heading vegetables, it is most practical to take the outermost whole wrapper leaf. When sampling particularly young plants, the whole above-ground portion of the plant may be sampled.
A proper leaf sample should consist of about 25 to 100 individual leaves. The same leaf (i.e., physiological age and position) should be removed from each sampled plant. Plants damaged by pests, diseases, or chemicals should be avoided when trying to monitor the nutrient status of the crop.
Individual plants, even side-by-side, may have a considerably different nutrient status. Therefore, by sampling a sufficiently large number of plants, the error due to this variability can be minimized. Figure 2 indicates the potential sampling error due to varying sample sizes. More accuracy in determining the actual nutrient status is derived from a larger sample size.
For a nutrient deficiency diagnosis, one composite tissue sample should be collected from the area exhibiting the disorder and a second sample from otherwise "normal" plants for comparison. Both samples should be of similar physiological age and from the same cultivar. The "disorder" sample and the "normal" sample must be properly separated from each other so a valid comparison can be made after analysis.
It is advisable to include a corresponding soil sample when submitting a diagnostic tissue sample. This practice is particularly important when the sample taken is from an area where a nutrient deficiency is suspected. The soil sample may indicate other factors, such as pH or nematodes, that may have a negative effect on crop growth and nutrient availability.
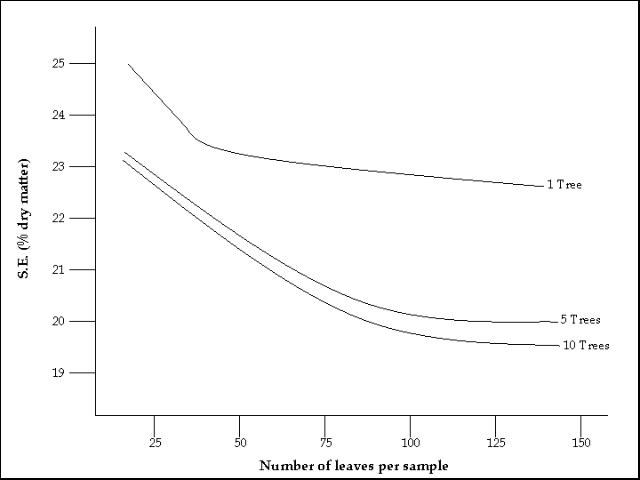
Credit: Holland et al. (1967)
Contaminants
Samples are often contaminated by fungicides, nutrient sprays, soil, or dust. Data obtained from contaminated leaf samples will be misleading. Decontamination of some dust or soil is best accomplished by quickly rinsing in a dilute non-phosphate detergent solution (2%) followed by two distilled water rinses. Tap water should not be used because it can be high in certain nutrients such as Ca, Fe, Mg, or S. Leaf samples should be washed quickly to minimize the leaching of certain nutrients (especially K) from the leaves. When testing for Fe, it is always necessary to wash the tissue as described above. It is not likely that contamination from chemical or nutrient sprays can be effectively removed from the leaf surface.
Preparation for Shipping
Following rinsing, the sample should be blotted dry with absorbent paper. The samples should be air-dried for several hours before shipment. If a plant analysis mailing kit is not available, the samples should be wrapped in fresh absorbent paper and placed in a large envelope (plastic bags must not be used). The sample should be mailed immediately to the soil and plant analysis laboratory. An air-dried sample, if loosely packed to avoid rotting, will last two to three days before decomposition begins.
If the samples must be held for any length of time before shipping, they should be dried at 150oF in a ventilated oven (leave the door ajar) until dry weight is constant. Once dried, the sample can be placed in a plant analysis mailing kit or a large envelope. This ensures the integrity of the sample until shipping is possible.
Considerations for Choosing a Laboratory
Tissue testing can be a valuable tool for monitoring nutrients within a growing crop. Tissue samples must be collected from the field, shipped to the laboratory, and analytical results with appropriate interpretations returned to the grower. Armed with this information, the grower can make a knowledgeable decision regarding possible additions of fertilizers to the crop. The time for this cycle to be completed must be held to a minimum. A reasonable time frame for this process is 3 to 5 working days for most vegetables, for diagnostic samples. For some short season crops, and for deficiency diagnosis, next-day service is needed.
Laboratory Location
Because of the need for short turnaround from sampling to receipt of the results, the best approach is to select a reliable laboratory close to the production area. However, if the producer is equipped with electronic mail or FAX instruments, delays for return of results can be greatly reduced. Priority mailing of tissue samples can further reduce the turnaround time. Thus, the need for the laboratory to be located relatively close to the production site is somewhat reduced, but the grower should still consider the physical problems of mailing as a factor in selecting a laboratory for tissue testing.
Since several tissue samples will be needed throughout the season, it is often advisable to make prior arrangements with the laboratory for all of the expected samples. Some laboratories offer a "package" for selected crops that includes a discount for a specified number of sampling dates.
The Land Grant University laboratories in the southeast region have been exchanging standardized plant samples for many years [Southern Region Information Exchange Group (SRIEG) 18 Work Group] and have found good agreement among the participating university laboratories. However, both laboratory procedures and methodology can influence tissue results, so it is usually advisable to continue testing with the same laboratory throughout the season and years to avoid possibly significant differences among laboratories.
Interpretation of Laboratory Results
While many laboratories do an excellent job of reporting the concentrations of nutrients in plant tissue, a few laboratories also provide accurate interpretations and recommendations based upon those results. That interpretations and recommendations may be provided with the report is no indication of their value for efficient crop production. Information (such as that contained in this circular) must be based upon research in local field conditions to be of use in interpreting laboratory results. Some laboratories might report the tissue results, compared with the average value for that crop and nutrient, observed by the lab in previous years. This average value might not be the critical concentration the grower is looking for because the average value includes results from crops of variable nutrient status or varieties. In other words, one needs the true critical concentration. Sometime the lab's "low", "medium", and "high" interpretation are simply a placement of the results relative to what is observed on average by the laboratory. Interpretations of this sort are misleading and of little help when making nutrient management decisions. A discussion concerning the procedure used for interpreting concentrations can assist with laboratory selection.
Diagnostic Tissue Testing
By its very nature, diagnostic tissue testing is only undertaken after a problem has been recognized. Often, the grower will see some visual clue that the crop is not as it should be. At this point, information to help make a diagnosis is needed, one component of which may be tissue analysis. Other information, such as soil testing, climatic data, pesticide, and fertilizer records, will often be needed besides nutrient status of the crop before the problem is correctly identified.
All of the considerations discussed with respect to nutrient monitoring pertaining to laboratory selection and location apply equally to diagnostic sampling. However, sample turnaround time may be the most important, since prompt reaction to some nutrient deficiencies is needed to avoid loss of yield and/or fruit quality.
Interpretations and recommendations of diagnostic samples should be a two-step process. The first interpretation should be based solely on the concentrations of nutrients found in the tissue sample. In short, do the nutrient levels represent deficiency or toxicity? The information in this circular can help with the answer to this question.
Secondly, results of samples from the affected area should be compared with those taken from an unaffected area: so-called "normal" and "disorder" areas. The samples should be taken at the same time so that a valid comparison can be made. The distance between the two composite samples should also be as small as possible.
This comparison will greatly aid in proper diagnosis. Often, a nutrient may be found to be at the lower level of the sufficiency range in the "disorder" sample, immediately making that nutrient suspect. However, comparison between "normal" and "disorder" levels may reveal that the nutrient is of similar magnitude in both samples, indicating that the symptoms may be caused by other factors.
Plant-Analysis Methods
The method used by the laboratory may greatly affect the meaning of the reported results. Many laboratory procedures, all radically different in approach, have been developed for plant analysis. For example, tests for P, K, etc., range from exotic neutron magnetic resonance (NMR) techniques to field quick-test kits. However, growers should patronize laboratories offering agricultural tests. These methods usually require destructive sampling, either by dry ashing the sample or by dissolving the sample in one or more acids. For small sample sets, some laboratories may employ microwave digestion in acids, but most laboratories will digest samples using a controlled temperature oven or heating apparatus. Testing of the resulting solutions by specific ion electrode methods is usually considered less accurate than colorimetric or spectrophotometric methods.
Methods that analyze the plant sap are usually only semiquantative measurements. Most field kits use this approach. While some of these kits are appropriate for field use by the grower for certain nutrients, the bulk of these procedures are not as precise as laboratory methods.
All reputable laboratories will monitor the accuracy and precision of test results. This process is usually referred to as a quality assurance program. It is this process that insures that numbers from the various tests are actually within acceptable accuracy ranges. A short discussion with the laboratory about their quality assurance program is good insurance against choosing the wrong laboratory. In all chemical and physical testing, it is agreed that an active quality assurance program has to be in place if any credence is to be given the results of the laboratory effort. Laboratories actively participating in the North American Proficiency Testing program meet or exceed plant tissue quality standards.
A common misconception is that two laboratories should be able to report the same, exact figures on split samples. Selection of methods and possibly different units of measure often cloud such expected agreement. For plant tissue analyses, the analytical results of split samples should be similar. For example, if one lab reports 4.8% N on one sample from a split-sample of tomato leaves, then the second lab results should be the same. In the final analysis however, the actual laboratory answer is but one step to making accurate interpretations and recommendations. It is the accuracy of the recommendation and subsequent positive crop response that is of value to the grower.
Listing of Commercial Laboratories for Agricultural Testing
The UF/IFAS Extension Soil Testing Laboratory (ESTL) offers only limited plant tissue testing to the public. Services for blueberry and pecan leaves are available. UF/IFAS Extension faculty may request diagnostic testing of other plant samples, but this service is not offered directly to the public. Therefore, a discussion with the local county extension faculty is recommended before any samples are sent to the ESTL.
The listing in Table 3 of commercial laboratories may be of use to the reader. This listing is not exhaustive. http://www.naptprogram.org/
Plant-Sap Quick Test for Nutrient Analysis
Much of the diagnostic information presented in this publication deals with analysis of dried plant material ( whole leaves, leaf blades, or petioles). The time period from sampling to recommendations for problem correction can be excessive for many situations involving deficiencies. Cost of routine sampling and analysis that involves many samples might be too high for many growers. However, the cost of tissue testing should be compared to the crop value at stake. Costs are often cited as hindrances to routine use of tissue testing in a fertilizer management program. Growers like the idea of tissue testing but may be reluctant to use it in a routine and timely fashion.
An alternative, for certain nutrients, to traditional laboratory analysis is a nutrient determination made on the fresh plant sap. Procedures for plant sap analysis have been available for years, but recently the techniques have been improved to make them more accurate and easier to use in the field. Most of these in-field plant sap "quick tests" should be used in conjunction with periodic laboratory analysis done on dried whole leaves.
Plant sap analysis kits are available in a range of sophistication from simple, hand-held "colorimeters" and ion-specific electrodes to sophisticated portable laboratory units that can test for a multitude of nutrients and chemicals. Growers interested in plant sap testing should evaluate their goals and purchase the equipment needed to meet the needs and avoid unneeded equipment. Often a $50 kit will suffice, but some growers who have the personnel, could benefit from larger, more diverse testing kits.
Plant sap kits can test for several plant nutrients but the user needs to evaluate the need for speed versus accuracy for the nutrients to be determined. For example, a sap test kit may not have the desired accuracy for certain micronutrients compared to traditional laboratory analyses using whole leaves.
Currently, plant sap test kits appear to have most utility for the mobile nutrients such as N, P, and K. These elements, particularly N and K, make up the bulk of nutrients applied as fertilizers to vegetable crops and also are the ones most often managed during the growing season, which makes plant sap testing particularly attractive for these elements. A good example is N management through the season with micro-irrigation. The routine use of a calibrated plant sap quick test could help a micro-irrigation manager make decisions regarding N scheduling for the crop. Proper management of N could reduce the overall fertilizer applications to that crop.
Recent studies in the University of Florida, Institute of Food and Agricultural Sciences (UF/IFAS), have provided calibration data for commercially available nitrate and K quick tests. The kits, described below, have been adapted to determine nitrate and K concentrations of fresh plant sap from petioles of most-recently-matured leaves. The initial work was conducted for tomato, although some work also has been done for other crops (cantaloupe, broccoli, cucumber, squash, and collards). The kits calibrated for use in Florida are described in Table 4.
Plant sap test kits are easy to use and result in rapid evaluations of plant sap for nitrate and potassium.
For sap testing, petioles collected from MRML are used for analyses. Most-recently-matured leaves (MRML) are leaves that have essentially ceased to expand and have turned from a juvenile light-green color to a darker-green color. A random sample of a minimum of 25 petioles should be collected from each "management unit" or "irrigation zone." Management units larger than 20 acres should be subdivided into 20-acre blocks. Leaves with obvious defects or with diseases should be avoided. Sampling should be done on a uniform basis for time of day (best between 10 AM and 2 PM), and for interval after rainfall or fertilization.
For tomatoes, the sample is usually the fifth or sixth leaf from the tip. Whole leaves are collected from the plant and the leaf blade tissue and leaflets are then stripped from the petiole. For tomatoes, a petiole of six to eight inches in length remains. Petioles are chopped into about one-half inch segments. If analysis is not to be conducted immediately in the field, then whole petioles should be packed with ice and analyzed within a few hours of collecting. Given more extreme environmental field conditions (high temperature and bright sun), more dependable results are obtained by making measurement in the lab or office than outdoors.
Chopped petiole pieces are mixed and a random subsample (about 1/4 cup) is crushed in a garlic press, lemon press, or hydraulic press (obtainable from HACH Co., Table 4). Expressed sap is collected in a small beaker or juice glass and stirred.
Early in the season, when sap nitrate-N concentrations are high, the sap might need to be diluted. Dilution makes it possible to read the nitrate-N levels within the scales of some test kits. Dilution also will minimize the interference of the green chlorophyll color of the sap on the reading of colorimetric testing systems. Some users have reported success with charcoal-filtered sap. This procedure is particularly good for dark sap that does not need to be diluted. Slightly different results will be obtained with filtered and unfiltered sap and users should standardize procedures with one method. With tomatoes, a dilution of 50 or 60 parts deionized or distilled water to one part sap is needed. Later in the season, a dilution of 20 to 1 will usually suffice. Diluting can be accomplished by using a laboratory pipette and graduated cylinder or less precisely, with an eyedropper. The pipette method is recommended for highest accuracy. Diluted sap is stirred completely prior to use in the test kits.
For the Quant strip test, a test strip is removed from the container (keep strips cool when not in use) and dipped for a second into the diluted sap. Following 60 seconds, the pink or purple color developed on the test pad on the end of the strip is compared to the calibrated color chart provided with the kit. Interpolation will be needed for readings between any two color blocks on the chart. An alternative is to use a newly developed strip color reader. This reflectometer provides for more quantitative evaluation of the color on the strip. Readings are made in parts per million (ppm) nitrates which can be converted into ppm nitrate-N by dividing by 4.45.
For the HACH colorimeter, two viewing tubes are filled with diluted sap. One tube is placed in its slot in the "comparator." Contents of one powder reagent pillow are emptied into the second diluted sap sample and the tube mixed for one minute. After mixing, the tube is placed in its slot in the "comparator" and left for one minute. After one minute, the colors in the viewing slots are matched by rotating the color wheel, and the resulting ppm of nitrate-N read from the dial.
For the Cardy meters, plant sap is pressed from the petioles and a drop is placed on the Cardy meter, covering both electrode spots on the meter. The meter must be calibrated with standard ion solutions before measuring ion concentration in the sap and again between every 6 or 8 measurements. There are specific meters for nitrate-N and K.
Current interpretations for these test kits for several vegetables are presented in Table 5. Work is continuing to provide data for additional crops and for other nutrients. Details on use and care of these sap measuring systems are presented in the EDIS publication Plant Petiole Sap-Testing Guide for Vegetable Crops (https://edis.ifas.ufl.edu/publication/cv004).
Correcting Nutrient Deficiencies
Nutrient deficiencies, if directly related to lack of fertilizer, must be corrected in timely fashion to avoid reduced yield and quality. It is best to avoid deficiencies by well executed soil-based nutrient programs, however, deficiencies if detected early enough can be corrected. Depending on the situation and cultural system used, several means of applying the needed fertilizer can be employed.
For open bare-ground culture, the deficient nutrient can be top dressed over the crop or banded along side of the row if the crop is not too large. Care must be taken to avoid soluble-salt damage to the crop or mechanical damage to the crop from the fertilizing equipment. For most macronutrients (N, P, K, Ca, Mg, S), a sidedressing of 30 to 40 lb. of element (P and K are in oxide form) per acre will correct a deficiency (Table 6).
Where polyethylene mulch is used, the nutrients must be applied to the root zone by manually punching holes in the mulch, with a liquid injection wheel, or through the micro-irrigation tubing, if that system is in place. Applying fertilizer in the alleys between the beds is not as effective as placing the fertilizer in the soil in the bed.
Foliar applications of macronutrients (N, P, K, Ca, Mg, or S) are not recommended due to inherent inefficiency. Too much nutrient is needed to overcome deficiencies in a short time period, which results in a high risk of foliar damage from soluble salt burn. Leaves are not well adapted for absorbing large amounts of nutrients in a short period due to the waxy cuticle and the inability to achieve uniform covering without soluble salt damage. These deficiencies are more effectively corrected by drenching or banding the needed nutrient in the root zone.
Micronutrient (Mn, Cu, Fe Zn, B, and Mo) deficiencies can be corrected by application of small amounts of the deficient nutrient (Table 6). Foliar application of the deficient micronutrient can be an effective means of correction if adequate leaf coverage is obtained. Micronutrients can be toxic in small amounts so care must be exercised to apply the recommended rates. For crops with waxy leaves, coverage can be improved by use of a spreader-sticker adjuvant in the spray tank.
Table of Deficient, Adequate, and Excessive Nutrient Concentrations for Vegetables
The following tables of nutrient concentrations were developed for vegetables from research conducted on vegetable nutrition. Tables 7 through 18 contain data for macronutrients N, P, K, Ca, Mg, and S and Tables 19 through 29 contain data on micronutrients Fe, Mn, Zn, B, Cu, and Mo. Much of these data were derived from fertilizer response research conducted in the United States with special emphasis on Florida. In these studies, researchers evaluated crop yield (and sometimes quality) response to varying rates of fertilizer nutrients on soils that contributed minimally to the crop nutrient requirement. Plant tissue nutrient concentrations from plants from those fertilizer treatments producing optimum yield and quality were selected as indicating adequate nutrition for a specific nutrient. Optimum fertilizer treatments were those fertilizer amounts above which no further increase in yields or quality resulted. Therefore, the corresponding tissue nutrient values would fall on the lower side of the sufficiency range.
Deficient nutrient values were those from fertilizer treatments that yielded significantly less than with the optimum treatments. These levels might not result in deficiency symptoms but are likely to result in reduced yields and quality.
In some situations, the dividing line between deficient and adequate values is not as clear as the table would indicate. For example, 2.0% and 2.1% might not be different from each other. For these "gray zone" values, one must use a common-sense approach to the interpretation.
The concentrations representing the adequate range (sufficiency range) are those nutrient concentrations to be found in plants that have adequate nutrients available to them. Plants with nutrient concentrations in the high range are indicative of over fertilization. Reduced yields and poor quality could result if the fertilizer rates are not reduced for these plants. For the micronutrients plant nutrient concentrations maintained in the high range could lead to phytotoxicity.
The reference tissues in Tables 5-29 are usually the MRML. This tissue is the whole leaf (blade plus petiole). This reference tissue is the most widely used plant part for most crops. However, for some crops, most of the interpretive research has been conducted for other plant parts (e.g., petioles).
General References
Burdine, H. W. 1976. Plant analysis for vegetable crops grown on Everglades organic soil. Univ. Fla. Belle Glade Agric. Res. and Ed. Ctr. Res. Report EV 7 pp.
Bould, C., E. J. Hewitt, and P. Needham. 1983. Diagnosis of mineral disorders in plants. Vol. I. Principles. Her Majesty's Stationery Office, London, Unipub, Lanham, MD, 20706.
Campbell, C. R. 2001. Reference sufficiency ranges for plant analysis in the southern region of the United States. South. Coop. Bull. 394.
Chapman, H. D. 1966. Diagnostic criteria for plants and soils. Univ. Calif. Div. Agric. Sciences. 793 pp.
Donohue, S. J. 1983. Reference soil test methods for the southern region of the United States. South. Coop. Ser. Bull. 289.
Donohue, S. J. 1992. Reference soil and media diagnostic procedures for the southern region of the United States. South. Coop. Ser. Vull. 374.
English, J. E. and D. N. Maynard. 1977. A key to nutrient disorders of vegetable plants. HortScience 13:28-29. https://doi.org/10.21273/HORTSCI.13.1.28
Epstein, E. 1972. Mineral nutrition of plants: principles and perspectives. J. Wiley and sons, Inc. New York, NY.
Glass, A. D. M. 1989. Plant nutrition. An introduction to modern concepts. Jones and Bartlett, Boston.
Hanlon, E. A. and G. J. Hochmuth. 1992. Recent changes in phosphorus and potassium fertilizer recommendations for tomato, pepper, muskmelon, watermelon, and snapbean in Florida. Commun. Soil Sci. Plant Anal., 23(17-20), 2651-2665. https://doi.org/10.1080/00103629209368763
Hanlon, E. A. 2001. Procedures used by state soil-testing laboratories in the southern region of the United States. South. Coop. Ser. Bull. 190-C.
Hartz, T. K. and G. J. Hochmuth. 1996. Fertility managments of drip-irrigated vegetables. HortTechnology 6:168-172. https://doi.org/10.21273/HORTTECH.6.3.168
Hochmuth, G. J. 2000. Management of nutrients in vegetable production systems in Florida. Soil Crop Sci. Soc. Fla Proc. 59:11-13.
Hochmuth, G. J. 1994. Efficiency ranges for nitrate-nitrogen and potassium for vegetable petiole sap quick tests. HortTechnology 4:218-222. https://doi.org/10.21273/HORTTECH.6.3.168
Hochmuth, G. J., E. A. Hanlon, B. C. Hochmuth. 1992. Responses of pepper, muskmelon, watermelon, and sweet corn to P and K fertilization at Live Oak, Fla. Suwannee Valley REC Research Report 92-28.
Hochmuth, G. J., Hanlon, E. A., and G. Kidder. 2000. Appropriate uses of soil fertility testing and the UF-IFAS standarized fertilization recommendation system: a position paper from the UF-IFAS plant nutrient oversight committee. Proc. Fla. State Hort. Soc. 113:138-140.
Hochmuth, G., E. Hanlon, B. Hochmuth, G. Kidder, and D. Hensel. 1993. Field fertility research with P and K for vegetables - interpretations and recommendations. Soil Crop Sci. Soc. Florida Proc. 52:95-101.
Hochmuth, G. J. 1992. Fertilizer managment for drip-irrigated vegetables in Florida. HortTechnology 2:27-32. https://doi.org/10.21273/HORTTECH.2.1.27
Hochmuth, G. J. 1992. Concepts and practices for improving nitrogen managment for vegetables. HortTechnology 2:121-125. https://doi.org/10.21273/HORTTECH.2.1.121
Holland, D. A., R. C. Little, M. Allen, and W. Dermott.1967. Soil and leaf sampling in apple orchards. J. Hort. Sci. 42:403-417. https://doi.org/10.1080/00221589.1967.11514224
Kidder, G. 1998. Procedures and practices followed by southern state soil testing laboratories for making liming recommendations. South. Coop. Ser. Bull. 380.
Locascio, S. J. 1987. Progress in nutrition of Florida vegetables during the past 100 years. Proc. Fla. State Hort. Soc. 100:398-405.
Locascio, S. J., and J. G. A. Fiskell. 1988. Vegetable needs for micronutrients in perspective. Soil and Crop Sci. Soc. Fla. Proc. 47:12-18.
Maynard, D. N. and G.J. Hochmuth. 1997. Knott's handbook for vegetable growers, fourth edition. Wiley, New York, NY. https://doi.org/10.1080/01904167909362696
Marschner, H. 1986. Mineral nutrition of higher plants. Academic Press, Inc. San Diego, Calif.
Maynard, D. N. 1979. Nutritional disorders of vegetable crops: A review. J. Plant Nutr. 1:1-23.
Mengel, K., and E. A. Kirkby. 1982. Principles of plant nutrition. Int'l Potash Inst., Worblaufen-Bern, Switzerland.
Mills, H. A., and J. B. Jones, Jr. 1996 Plant Nutrition Handbook II, Micro-Macro Publ, Athens, GA.
Mitchell, C. C. 1994. Research-based soil testing interpretation and fertilizer recommendations for peanuts on coastal plan soils. South. Coop. Ser. Bull. 380.
Plank, C. O. 1988. Plant analysis handbook for Georgia. Univ. Ga. Coop. Ext. Misc. Public.
Plank, C. O. 1992. Plant analysis reference procedures for the southern region of the United States. South. Coop. Ser. Bull. 368
Plucknett, D. L., and H. B. Sprague (eds.). 1989. Detecting mineral nutrient deficiencies in tropical and temperate crops. Westview Press, Inc. Boulder Colorado.
Reisenauer, H. M. (ed.). Soil and plant tissue testing in California. Univ. Calif. Div. Agr. Sci. Bull. 1879.
Reuter, D. J., and J. B. Robinson. 1986. Plant analysis, and interpretation manual. Inkata Press, Melbourne, Australia.
Scaife, A., Mary Turner, and P. Wood. 1983. Diagnosis of mineral disorders in plants. Vol. II. Vegetables. Her Majesty's Stationery Office, London, Unipub, Lanham, Md.
Sims, G. T., and C. M. Volk. 1947. Composition of Florida-grown vegetables. I. Mineral composition of commercially grown vegetables in Florida as affected by treatment, soil type, and locality. Fla. Agric. Exp. Sta. Bull 438.
Sprague, H. B. (ed.). 1964. Hunger signs in crops, third edition, David McKay Co., Inc., New York. https://doi.org/10.1097/00010694-196409000-00014
Thom, W. O., and W. Sabbe. 1994. Soil sampling procedures for the southern region of the United States. South. Coop. Ser. Bull. 377.
The North American Proficiency Testing Program (NAPT) has a Website at: http://www.naptprogram.org/
Walsh, L. M., and J. D. Beaton (eds.). 1973. Soil testing and plant analysis. Soil Sci. Amer., Inc., Madison, Wis.
Winsor, G., P. Adams, F. Fiske, and J. B. D. Robinson, 1983. Diagnosis of mineral disorders in plants. Vol. III. Glasshouse crops. Her Majesty's Stationery Office, London, Unipub, Lanham, Md, 20706.
Vavrina, C. S. 1988. Plant tissue analysis for vegetable crops. Univ. Georgia Coop. Ext. Serv. Misc. public. MP-310.
References by Crop
Broccoli
Magnifico, V., V. Lattanzio, and C. Sarli. 1979. Growth and nutrient removal by broccoli. J. Amer. Soc. Hort. Sci. 104:201-203. https://doi.org/10.21273/JASHS.104.2.201
Peck, N. H., and G. E. MacDonald. 1986. Cauliflower, broccoli, and brussels sprouts responses to concentrated superphosphate and potassium chloride fertilization. J. Amer. Soc. Hort. Sci. lll:195-201. https://doi.org/10.21273/JASHS.111.2.195
Brussels Sprouts
Peck, N. H., and G. E. MacDonald. 1986. Cauliflower, broccoli, and brussels sprouts responses to concentrated superphosphate and potassium chloride fertilization. J. Amer. Soc. Hort. Sci. lll:195-201. https://doi.org/10.21273/JASHS.111.2.195
Welch, N. C., K. B. Tyler, and D. Ririe. 1985. Nitrogen rates and nitrapyrin influence on yields of brussels sprouts, cabbage, cauliflower, and celery. HortScience 20:1110-1112. https://doi.org/10.21273/HORTSCI.20.6.1110
Cabbage
Csizinszky, A. A., and D. J. Schuster. 1985. Response of cabbage to insecticide schedule, plant spacing, and fertilizer rates. J. Amer. Soc. Hort. Sci. 110:888-893. https://doi.org/10.21273/JASHS.110.6.888
Csizinszky, A. A., and C. D. Stanley. 1984. Effect of trickle tubes per bed and N and K rates on spring broccoli and cabbage yields. Soil Crop Sci. Soc. of Fla. Proc., Vol:43:51-55.
Forbes, R. B., J. B. Sartain, and N. R. Usherwood. 1984. Optimum K fertilization schedule for maximizing yields of cabbage, sweetcorn, and soybeans grown in a multiple cropping sequence. Soil Crop Sci. Soc. Fla. Proc. 43:64-68.
Harrison, H. C., and E. L. Bergman. 1981. Calcium, magnesium, and potassium interrelationships affecting cabbage production. J. Amer. Soc. Hort. Sci. 106:500-503. https://doi.org/10.21273/JASHS.106.4.500
Knavel, D. E. and J. W. Herron. 1981. Influence of tillage system, plant spacing, and nitrogen on head weight, yield, and nutrient concentration of spring cabbage. J. Amer. Soc. Hort. Sci. 106:540-545. https://doi.org/10.21273/JASHS.106.5.540
Hochmuth, R. C., G. J. Hochmuth, and M. E. Donley. 1993. Responses of cabbage yields, head quality, and leaf nutrient status, and of second-crop squash, to poultry manure fertilization. Soil Crop Sci. Soc. Fla Proc. 52:126-130.
Peck, N. H. and J. R. Stamer. 1970. Plant response to concentrated superphosphate and potassium chloride fertilizers. N.Y. State Agr. Expt. Sta. Res. Bul. 830.
Welch, N. C., K. B. Tyler, and D. Ririe. 1985. Nitrogen rates and nitrapyrin influence on yields of brussels sprouts, cabbage, cauliflower, and celery. HortScience 20:1110-1112. https://doi.org/10.21273/HORTSCI.20.6.1110
Carrot
Burdine, H. W., and C. B. Hall. 1976. Carrot responses to fertilizer levels on Everglades organic soils. Proc. Fla. State Hort. Soc. 89:120-125.
Gupta, U. C., and J. A. Cutcliffe. 1985. Boron nutrition of carrots and table beets grown in a boron deficient soil. Commun. Soil Sci. Plant Anal. 16:509-516. https://doi.org/10.1080/00103628509367623
Hemphill, D. D., and T. L. Jackson. 1982. Effect of soil acidity and nitrogen on yield and elemental concentration of bush bean, carrot, and lettuce. J. Amer. Soc. Hort. Sci. 107(5):740-744. https://doi.org/10.21273/JASHS.107.5.740
Hipp, B. W.1978. Response by carrots to nitrogen and assessment of nitrogen status by plant analysis. HortScience 13:43-44. https://doi.org/10.21273/HORTSCI.13.1.43
Hochmuth, G. J., J. K. Brecht, and M. J. Bassett. 1999. Nitrogen fertilization to maximize carrot yield and quality on a sandy soil. HortScience 34(4):641-645. https://doi.org/10.21273/HORTSCI.34.4.641
Hochmuth, R. C., M. Burani-Arouca, and C. E. Barrett. 2021. Yield and quality of carrot cultivars with eight nitrogen rates and best management practices. HortScience 56 (10): 1199-1205. https://doi.org/10.21273/HORTSCI15983-21
Cauliflower
Peck, N. H., and G. E. MacDonald. 1986. Cauliflower, broccoli, and brussels sprouts responses to concentrated superphosphate and potassium chloride fertilization. J. Amer. Soc. Hort. Sci. 111:195-201. https://doi.org/10.21273/JASHS.111.2.195
Wall, T. E., G. J. Hochmuth, and E. A. Hanlon. 1988. Calibration of Mehlich-Iand -III extractable potassium for polyethylene drip irrigated cauliflower. Soil Crop Sci. Soc. Fla. Proc. 48:46-49.
Welch, N. C., K. B. Tyler, and D. Ririe. 1985. Nitrogen rates and nitrapyrin influence on yields of brussels sprouts, cabbage, cauliflower, and celery. HortScience 20:1110-1112. https://doi.org/10.21273/HORTSCI.20.6.1110
Celery
Burdine, H. W., and V. L. Guzman. 1965. The response of some green celery varieties to pH adjustment with sulphur on everglades organic soil. Proc. Fla. State Hort. Soc. 78:148-156.
Burdine, H. W., and V. L. Guzman. 1969. Some celery responses to fertilizer levels. Univ. Fla. Everglades Station Mimeo Report EE569-17.
Welch, N. C., K. B. Tyler, and D. Ririe. 1985. Nitrogen rates and nitrapyrin influence on yields of brussels sprouts, cabbage, cauliflower, and celery. HortScience 20:1110-1112. https://doi.org/10.21273/HORTSCI.20.6.1110
Collards
del Valle, C. C. 1971. Influence of seeding rate, source, and level of nitrogen on collards. J. Amer. Soc. Hort. Sci 96:25-26. https://doi.org/10.21273/JASHS.96.1.25
Cucumber
Cantliffe, D. J. 1977. Nitrogen fertilizer requirements of pickling cucumbers grown for once-over harvest I. Effect on yield and fresh quality. J. Amer. Soc. Hort. Sci. 102:112-114. https://doi.org/10.21273/JASHS.102.2.112
Evanylo, G. K. and D. V. Midkiff. 1987. Influence of soil fertility on slicer cucumbers. Virginia Cooperative Extension Service, The Vegetable Growers News, Vol. 42, No. 3.
Hochmuth, R. C. and G. J. Hochmuth. 1991. Nitrogen requirement for mulched slicing cucumbers. Soil Crop Sci. Soc. Fla. Proc. 50:130-133.
Navarro, A. A., and S. J. Lacascio. 1973. Cucumber response to copper rate and fertilizer placement. Proc. Fla. State Hort. Soc. 86:193-195.
Navarro, A. A., and S. J. Locascio. 1980. Copper nutrition of cucumber as influenced by fertilizer placement, phosphorus rate, and phosphorus source. Soil Crop Sci. Soc. Fla. Proc. 39:16-19.
Smith, C., G. Hochmuth, and G. Jones. 2000. Cucumber responses to soil Mg and to Mg fertilization were not predictable. Proc. Fla. State Hort. Soc. 113:261-265.
Eggplant
Hochmuth, G., R. Hochmuth, E. Hanlon, and M. Donley. 1991. Nitrogen requirements of mulched eggplant in northern Florida. Suwannee Valley REC Report #91-14.
Hochmuth, G. J., R. C. Hochmuth, E. A. Hanlon, M. E. Donley. 1992. Effect of potassium on yield and leaf-N and K concentrations of eggplant. Suwannee Valley REC Report #92-2.
Hochmuth, G. J., R. C. Hochmuth, M. E. Donley, and E. A. Hanlon. 1993. Eggplant yield in response to potassium fertilization on sandy soil. HortScience 28:1002-1005. https://doi.org/10.21273/HORTSCI.28.10.1002
Hochmuth, G., and R. Hochmuth. 1995. Effects of K amounts and proportions of K supplied from controlled-release potassium nitrate on eggplant yield. Suwannee Valley REC Report #95-6.
Ozaki, H. Y., and J. R. Iley. 1965. Magnesium, iron, manganese, and zinc ammanium phosphates as fertilizer sources for eggplant. Soil Crop Sci. Soc. Fla. Proc. 25:123-128.
Seaker, E. M., E. L. Bergman, and C. P. Romaine. 1982. Effects of magnesium on tobacco mosaic virus-infected eggplants. J. Amer. Soc. Hort. Sci. 107:162-166. https://doi.org/10.21273/JASHS.107.1.162
Lettuce
Burdine, H. W., C. B. Hall, and J. R. Hicks. 1976. Responses of some leafy vegetables to varying fertility levels on Terra Ceia muck. Soil and Crop Sci. Soc. Fla. Proc. 36:46-51.
Cantliffe, D. J., G. J. Hochmuth, Z. Karchi, and I. Secker. 1997. Nitrogen fertility requirement for iceberg lettuce grown on sandland with plastic mulch and drip irrigation. Proc. Fla. State Hort. Soc. 110:306-309.
Diaz, O. A., E. A. Hanlon, Jr., G. J. Hochmuth, and J. M. White. 1988. Phosphorus and potassium nutrition of lettuce on a Florida muck. Soil Crop Sci. Soc. Fla. Proc. 47:36-41.
Guzman, V. L., and R. E. Lucas. 1979. Preliminary investigations on the nutrient requirements of crisp-head lettuce cultivars. Belle Glade, AREC Research Report EV-1979-4.
Lucas, R. E., and V. L. Guzman. 1980. Crisphead lettuce - Plant nutrient trials. Univ. Fla. Belle Glade AREC Research Report EV-1980-9.
Pew, W. D., B. R. Gardner, and P. M. Bessey. 1983. Comparison of controlled-released nitrogen fertilizers, urea, and ammonium nitrate on yield and nitrogen uptake by fall-grown head lettuce. J. Amer. Soc. Hort. Sci. 108:448-453. https://doi.org/10.21273/JASHS.108.3.448
Sanchez, C. A., and H. W. Burdine. 1989. Soil testing and plant analysis as guides for the fertilization of escarole and endive on histosols. Soil and Crop Sci. Soc. Fla. Proc. 48:37-40.
Lima Bean
Smith, C. B. 1980. Growth responses and leaf nutrient concentrations of 'Fordhook 242' lima beans as affected by fertilizer treatment and plant stand. J. Amer. Soc. Hort. Sci. 105:472-475. https://doi.org/10.21273/JASHS.105.3.472
Muskmelon (Cantaloupe)
Bhella, H. S., and G. E. Wilcox. 1986. Yield and composition of muskmelon as influenced by preplant and trickle applied nitrogen. HortScience 21:86-88. https://doi.org/10.21273/HORTSCI.21.1.86
Bhella, H. S., and G. E. Wilcox. 1989. Lime and nitrogen influence soil acidity, nutritional status, vegetative growth, and yield of muskmelon. J. Amer. Soc. Hort. Sci. 114:606-610. https://doi.org/10.21273/JASHS.114.4.606
Brantley, B. B., and G. F. Warren. 1960. Effect of nitrogen nutrition on flowering, fruiting, and quality in the muskmelon. Proc. Amer. Soc. Hort. Sci. 77:424-431.
DeBuchananne, D. A., and H. G. Taber. 1985. Method of nitrogen application for muskmelons. J. Plant Nutr 8: 265-275. https://doi.org/10.1080/01904168509363341
Elamin, O. M., and G. E. Wilcox. 1986. Effect of magnesium and manganese nutrition on muskmelon growth and manganese toxicity. J. Amer. Soc. Hort. Sci. 111:582-587. https://doi.org/10.21273/JASHS.111.4.582
Elamin, O. M., and G. E. Wilcox. 1986. Effect of soil acidity and magnesium on muskmelon leaf composition and fruit yield. J. Amer. Soc. Hort. Sci. 111:682-685. https://doi.org/10.21273/JASHS.111.5.682
Flocker, W. J., J. C. Lingle, R. M. Davis, and R. J. Miller. 1964. Influence of irrigation and nitrogen fertilization on yield, quality, and size of cantaloupes. Proc. Amer. Soc. Hort. Sci. 86:424-431.
Gubler, W. D. 1982. Yellows of melons caused by molybdenum deficiency in acid soil. Plant Disease 66:449-451. https://doi.org/10.1094/PD-66-449
Hochmuth, G. J., E. Hanlon, and R. C. Hochmuth. 1991. Nitrogen crop nutrient requirements for muskmelons grown in various polyethylene mulch systems. Univ. Fla. Suwannee Valley REC Report #91-5.
Hochmuth, G., and R. Hochmuth. 1995. Effects of K rate and proportions of K supplied from controlled-release K on muskmelon. Univ. Fla. Suwannee Valley REC Report #95-07.
Hochmuth, G. and M. Gal. 2001. Muskmelon fruit response to K source and method of application. Proc. Fla. State Hort. Soc. 114:312-315.
Lorenz, 0. A., B. L. Weir, and J. C. Bishop. 1972. Effect of controlled-released nitrogen fertilizers on yield and nitrogen absorption by potatoes, cantaloupes, and tomatoes. J. Amer. Soc. Hort. Sci. 97:334-337. https://doi.org/10.21273/JASHS.97.3.334
Stark, F. C., and I. C. Haut. 1958. Mineral nutrient requirements of cantaloupes with reference to nitrogen, potassium, calcium, magnesium, and boron, Md. Agric. Exp. Sta. Bull. A-93. 37 pp.
Wiedenfeld, R. P. 1986. Rate, timing, and slow-release nitrogen fertilizers on bell peppers and muskmelon. HortScience 21:233-235. https://doi.org/10.21273/HORTSCI.21.2.233
Wilcox, G. E. 1973. Muskmelon response to rates and sources of nitrogen. Agron. J. 65:694-697. https://doi.org/10.2134/agronj1973.00021962006500050005x
Okra
Ahmad, N., and L. I. Tullach. 1968. Effects of fertilizer nitrogen, phosphorus, potassium, and magnesium on yield and nutrient content of okra (Hibiscus esculentus L). Agron. J. 60:353-449. https://doi.org/10.2134/agronj1968.00021962006000040006x
Asif, M. I., and J. K. Greig. 1972. Effects of N, P, and K fertilization on fruit yield, Macro- and micronutrient levels, and nitrate accumulation in okra (Abelmoschus esculentus (L.) Moench). J. Amer. Soc. Hort. Sci. 97:440-442. https://doi.org/10.21273/JASHS.97.4.440
Majanbu, Is, V. B. Ogunlela, and M. K. Ahmed. 1986. Response of two okra (Abelmoschus esculentus (L.) Moench) varieties to fertilizers: growth and nutrient concentration as influenced by nitrogen and phosphorus application. Fertilizer Research 8:297-306. https://doi.org/10.1007/BF01048632
Sutton, Paul. 1963. The response of okra to nitrogen, phosphorus and potassium fertilization. Proc. Fla. State Hort. Soc. 149-152.
Onion
Hochmuth, G. J., R. C. Hochmuth, E. A. Hanlon, and M. E. Donley. 1991. Responses of Florida sweet onions to N and K fertilization. Univ. Fla. Suwannee Valley REC Report #91-11.
Minotti, P. L., and K. W. Stone. 1988. Consequences of not fertilizing onions on organic soils with high soil test values. Commun. Soil Sci. Plant Anal 19:1887-1906. https://doi.org/10.1080/00103628809368058
Smittle, D. A. 1984. Responses of onions to sulfur and nitrogen fertilization. The University of Ga. Agric. Expt. Stat. Res. Rpt. 455.
Voss, R. E. 1979. Onion production in California. Cooperative Extension, Division of Agricultural Sciences, Univ. Ca. Pub. 4097.
Pepper
Hochmuth, G. J., K. D. Shuler, R. .L. Mitchell, P. R. Gilreath. 1987. Nitrogen crop nutrient requirement demonstrations for mulched pepper in Florida. Proc. Fla. State Hort. Soc. 100:205-209.
Hochmuth, G. J., K. D. Shuler, P. R. Gilreath, and R. L. Mitchell. 1988. Field-testing of revised Mehlich-I-predicted potassium fertilizer recommendations for mulched pepper. Soil Crop Sci. Soc. Fla. Proc. 47:30-35.
Hochmuth, G., E. Hanlon, and R. Hochmuth. 1992. Response of pepper to N fertilization in a polyethylene mulch and drip irrigation production system at Live Oak, FL, Spring 1988. Univ. Fla. Suwannee Valley REC Report #92-29.
Hochmuth, G., and R. Hochmuth. 1994. Jalapeno pepper response to N and K fertilization. Univ. Fla. Suwannee Valley REC Report #94-05.
Hochmuth, G., K. Shuler, E. Hanlon, and N. Roe. 1994. Pepper response to fertilization with soluble and controlled-release potassium fertilizaters. Proc. Fla. State Hort. Soc. 107:132-139.
Hochmuth, G., and R. Hochmuth. 1995. Effects of K rate and proportion of K supplied from controlled-release K on pepper. Univ. Fla. Suwannee Valley REC Report #95-8.
Knavel, D. E., J. Ellis, and J. Morrison. 1977. The effects of tillage systems an the performance and elemental absorption by selected vegetable crops. J. Amer. Soc. Hort. Sci. 102:323-327. https://doi.org/10.21273/JASHS.102.3.323
Locascio, S. J., and J. G. A. Fiskell. 1976. Pepper production as influenced by mulch, fertilizer placement, and nitrogen rate. Soil Crop Sci. Soc. Fla. Proc. 36:113-117.
Locascio, S. J., J. G. A. Fiskell, and F. G. Martin. 1981. Responses of bell pepper to nitrogen sources. J. Amer. Soc. Hort. Sci. 106:628-632. https://doi.org/10.21273/JASHS.106.5.628
Miller, C. H., R. E. McCollum, and S. Claimon. 1979. Relationships between growth of bell peppers (Capsicum annuum L.) and nutrient accumulation during ontogeny in field environments. J. Amer. Soc. Hort. Sci. 104:852-857. https://doi.org/10.21273/JASHS.104.6.852
Shaw, N. L., G. J. Hochmuth, and E. A. Hanlon. 1996. N fertilization management for drip irrigated bell pepper (Capsicum annuum L.). Proc. Fla. State Hort. Soc. 109:136-141.
Wiedenfeld, R. P. 1986. Rate, timing, and slow-release nitrogen fertilizers on bell peppers and muskmelon. HortScience 21:233-235. https://doi.org/10.21273/HORTSCI.21.2.233
Potato
Bundy, L. G., R. P. Walkowski, and G. G. Weis. 1986. Nitrogen source evaluation for potato production on irrigated sandy soils. Amer. Potato J. 63:385-398. https://doi.org/10.1007/BF02854101
Elkashif, M. E., and S. J. Lacascio, and D. R. Hensel. 1983. Isobutylidene diurea and sulfur-coated urea as N sources for potatoes. J. Amer. Soc. Hort. Sci. 108(4):523-526. https://doi.org/10.21273/JASHS.108.4.523
Hensel, D. R. 1962. Phosphorus fertilization of potatoes an Ona fine sand. Soil Crop Sci. Soc. Fla. Proc. 22:130-131.
Hochmuth, G., E. Hanlon, and B. Hochmuth. 1992. Foliar nutritional sprays did not improve yields or grade of watermelons or potatoes. Univ. Fla. Suwannee Valley REC Report #92-26.
Hochmuth, G., E. Hanlon, and B. Hochmuth. 1992. N and K fertilization of red potatoes at Live Oak, FL. Spring 1988. Univ. Fla. Suwannee Valley REC Report #92-30.
Hochmuth, G. J., P. Weingartner, C. Hutchinson, A. Tilton, and D. Jesseman. 2002. Potato yield and tuber quality did not respond to phosphorus fertilization of soils testing high in phosphorus content. HortTechnology 12:420-423. https://doi.org/10.21273/HORTTECH.12.3.420
Hochmuth, G., E. Hanlon, G. Kidder, D. Hensel, W. Tilton, J. Dilbeck, and D. Schrader. 1993. Fertilization demonstrations for the tri-county potato production area of northeast Florida. Proc. Fla. State Hort. Soc. 106:190-198.
Locascio, S. J., and H. L. Breland. 1963. Irish potato yield and leaf composition as affected by dolomite and phosphorus. Soil Crop Sci. Soc. Fla. Proc. 23:95-99.
Locascio, S. J., and R. D. Rhue. 1990. Phosphorus and micronutrient sources for potato. Amer. Pot. J. 67:217-266. https://doi.org/10.1007/BF02987262
Rhue, R. D., D. R. Hensel, and G. Kidder. 1986. Effect of K fertilization on yield and leaf nutrient concentrations of potatoes grown on a sandy soil. Amer. Pot. J. 63:665-681. https://doi.org/10.1007/BF02852981
Pumpkin
Swiader, J. M., J. G. Sullivan, J. A. Grunau, and F. Freiji. 1988. Nitrate monitoring for pumpkin production on dryland and irrigated soils. J. Amer. Soc. Hort. Sci. 113:684-689. https://doi.org/10.21273/JASHS.113.5.684
Radish
Burdine, H. W. 1976. Radish responses to nitrogen source. Soil Crop Sci. Soc. of Fla. Proc. 35:59-63.
Snap Bean
Hemphill, D. D. Jr., and T. L. Jackson. 1982. Effect of soil acidity on yield and elemental concentration of bush bean, carrot, and nitrogen and lettuce. J. Amer. Soc. Hort. Sci. 107:740-744. https://doi.org/10.21273/JASHS.107.5.740
Hochmuth, G., B. Hochmuth, and E. Hanlon. 1992. Comparison of various N scheduling methods for snap beans. Univ. Fla. Suwannee Valley REC Report #92-5.
Hochmuth, G., B. Hochmuth, and E. Hanlon. 1992. Response of snap bean to nitrogen fertilization on a sandy soil. Univ. Fla. Suwannee Valley REC Report #92-6.
Hochmuth, G., B. Hochmuth, and E. Hanlon. 1992. Field test of IFAS P and K recommendations for snap beans. Univ. Fla. Suwannee Valley REC Report #92-15.
Mack, H. J. 1983. Fertilizer and plant density effects on yield performance and leaf nutrient concentration of bush snap beans. J. Amer. Soc. Hort. Sci. 108:574-578. https://doi.org/10.21273/JASHS.108.4.574
Peck, N. H., G. E. MacDonald, and A. V. Gardner. 1989. Snap bean plant responses to sources and rates of nitrogen and potassium fertilizers. HortScience 24:619-623. https://doi.org/10.21273/HORTSCI.24.4.619
Palaniyandi, R., and C. B. Smith. 1978. Growth and nutrient interrelationships in snap beans as affected by several sources of potassium and magnesium. J. Amer. Soc. Hort. Sci. 103:109-113. https://doi.org/10.21273/JASHS.103.1.109
Paterson, D. R., J. D. Dolwnes, N. H. Peck, H. Ozaki, K. B. Tyler, and S. C. Wiggans. 1966. Effects of nitrogen on yield, quality, and mineral uptake of 'Harvester' snap beans. Tex. Agric. Exp. Sta. Bull. MP 11 pp.
Rhoades, F. M., E. A. Hanlon, S. M. Olson, and G. J. Hochmuth, 1990. Responses of snap beans to N- and soil P and K. Soil Crop Sci. Soc. Fla. Proc. 49.
Smith, C. B. 1977. Growth responses, nutrient leaf concentrations and interelement relationships of snap beans as affected by fertilizer treatment. J. Amer. Soc. Hort. Sci. 102:61-64. https://doi.org/10.21273/JASHS.102.1.61
Smittle, D. A. 1976. Response of snap bean to irrigation, nitrogen fertilization, and plant population. J. Amer. Soc. Hort. Sci. 101:37-40. https://doi.org/10.21273/JASHS.101.1.37
Sutton, P., E. E. Albregts, and C. M. Howard. 1972. Influence of N and K on pole bean (Phaseolus vulgaris L.) response and soil and plant analysis. Soil and Crop Sci. Soc. of Fla. Proc. 32:136-138.
Southern Pea
Hochmuth, G., and B. Hochmuth. 1992. Southern pea response to nitrogen fertilization. Univ. Fla. Suwannee Valley REC Report #92-7.
Johnson, W. A., and C. E. Evans. 1975. Nitrogen, phosphorus, and potassium fertilization of southern peas for one-time harvest on a sandy soil. J. Amer. Soc. Hort. Sci. 100(3):261-263. https://doi.org/10.21273/JASHS.100.3.261
Stewart, F. B., and M. Reed. 1969. The effect of fertilizer on yield, growth, and mineral composition of southern peas. J. Amer. Soc. Hort. Sci. 94:258-260. https://doi.org/10.21273/JASHS.94.3.258
Worley, R. E., D. A. Hegwood, and S. A. Harmon. 1971. Effects of nitrogen, phosphorus, and potassium on yield and leaf analysis of southern pea. J. Amer. Soc. Hort. Sci. 96:531-533. https://doi.org/10.21273/JASHS.96.4.531
Spinach
Brown, J. R., V. N. Lambeth, and D. G. Blevins. 1969. Nutrient interaction effects on yield and chemical composition of spinach and green beans. Mo. Agric. Exp. Sta. Res. Bull 963.
Squash
Hochmuth, G. J., and R. C. Hochmuth. 1992. Nitrogen fertilization of summer squash on a sandy soil. Univ. Fla. Suwannee Valley REC Report #92-19.
Hochmuth, R. C., G. J. Hochmuth, and M. E. Donley. 1993. Responses of cabbage yields, head quality, and leaf nutrient status, and of second-crop squash, to poultry manure fertilization. Soil Crop Sci. Soc. Fla. Proc. 52:126-130.
Strawberry
Albregts, E. E., and P. Sutton. 1971. Response of strawberry to N and K fertilization on a sandy soil. Soil Crop Sci. Soc. Fla. Proc. 31:114-116.
Albregts, E. E., C. M. Howard, and F. C. Martin. 1974. Influence of fertility level on yield response of strawberries. Soil Crop Sci. Soc. Fla. Proc. 33:215-218.
Albregts, E. E., and C. M. Howard. 1980. Accumulation of nutrients by strawberry plants and fruit grown in annual hill culture. J. Amer. Soc. Hort. Sci. 105:386-388. https://doi.org/10.21273/JASHS.105.3.386
Albregts, E. E., and C. M. Howard. 1981. N, P, K composition of and accumulation by strawberry plant organs from transplanting through fruit harvest. Soil Crop Sci. Soc. Fla. Proc. 40:30-33.
Albregts, E. E., and E. M. Howard. 1984. Strawberry Production in Florida. Fla. Agric. Exp. Sta., Bull. 841.
Albregts, E. E., and C. M. Howard. 1984. Boron application to strawberries. Soil Crop Sci. Soc. Fla. Proc. 43:11-14.
Albregts, E. E., and C. M. Howard 1984. Effect of three slow release fertilizers on fruiting strawberries. Soil and Crop Sci. Soc. Fla. Proc. 43:10-11.
Albregts, E. E., and C. M. Howard. 1986. Supplemental foliar fertilization of fruiting strawberries. Proc. Fla. State Hort. Soc. 99:329-331.
Albregts, E. E., and C. M. Howard. 1987. Fertilizer rate and method of application on fruiting strawberry. Proc. Fla. State Hort. Soc. 100:198-200.
Albregts, E. E., and C. M. Howard. 1987. Response of strawberry to foliar applications of Cu, Mn, and Zn. Soil and Crop Sci. Soc. Fla. Proc. 46:89-91.
Albregts. E. E., G. J. Hochmuth, C. K. Chandler, J. Cornell, and J. Harrison. 1996. Potassium fertigation requirements of drip-irrigated strawberry. J. Amer. Soc. Hort. Sci. 121:164-168. https://doi.org/10.21273/JASHS.121.1.164
Hall, C. B., and R. A. Dennison. 1956. Lime-induced manganese deficiency of strawberries. Proc. Fla. State Hort. Soc. 69:228-229.
Hochmuth, G. J., E. A. Albregts, C. C. Chandler, J. Cornell, and J. Harrison. 1996. Nitrogen fertilization requirements of drip-irrigated strawberries. J. Amer. Soc. Hort. Sci. 121:660-665. https://doi.org/10.21273/JASHS.121.1.164
Locascio, S. J., and G. K. Saxena. 1967. Effects of potassium source and rate and nitrogen rate on strawberry tissue composition and fruit yield. Proc. Fla. State Hort. Soc. 80:173-176.
Locascio, S. J., J. M. Myers, and F. G. Martin. 1977. Frequency and rate of fertilization with trickle irrigation for strawberries. J. Amer. Soc. Hort. Sci. 102:456-458. https://doi.org/10.21273/JASHS.102.4.456
Locascio, S. J., and F. G. Martin. 1985. Nitrogen source and application timing for trickle irrigated strawberries. J. Amer. Hort. Sci. 110:820-823. https://doi.org/10.21273/JASHS.110.6.820
Sweet Corn
Forbes, R. B., J. B. Sartain, and N. R. Usherwood. 1984. Optimum K fertilization schedule for maximizing yields of cabbage, sweetcorn, and soybeans grown in a multiple cropping sequence. Soil Crop Sci. Soc. Fla. Proc. 43: 64-68.
Hochmuth, G., B. Hochmuth, and M. Donley. 1992. Nitrogen fertilization of sweet corn on a sandy soil in northern Florida. Univ. Fla. Suwannee Valley REC Report #92-8.
Hochmuth, G. 1994. Sweet corn response to N and K fertilization and to vitamins as growth enhancers. Suwannee Valley REC Report #94-01.
Hochmuth, G. J., E. Hanlon, S. O'Hair, J. Carranza, and M. Lamberts. 1995. On-farm evaluations of University of Florida N, P, and K recommendations for sweet corn on rockdale and marl soils. Proc. Fla. State Hort. Soc. 108:184-192.
Maynard, D. N., C. L. Thomson, R. A. Damon, Jr., D. A. Marini, W. Melnick, and R. A. Miller. 1971. Sweet corn leaf composition and its relationship to soil fertility. Mass. Agri. Expt. Sta. Bull. No. 587.
Peck, N. H., G. E. MacDonald, and J. Barnard. 1988. Sweet corn seedling responses to band-applied nitrogen, phosphorus, and potassium fertilizers. J. Amer. Soc. Hort. Sci. 113:336-342. https://doi.org/10.21273/JASHS.113.3.336
Peck, N. H., and G. E. MacDonald 1988. Sweet corn plant responses to P and K in the soil and to band-applied monoammonium phosphate, potassium sulfate, and magnesium sulfate. J. Amer. Soc. Hort. Sci. 114:269-272. https://doi.org/10.21273/JASHS.114.2.269
Rudert, B. D., and S. J. Locascio. 1979. Growth and tissue composition of sweet corn as affected by nitrogen source, nitrapyrin, and season. J. Amer. Soc. Hort. Sci. 104:520-523. https://doi.org/10.21273/JASHS.104.4.520
Smith, C. B., B. B. Chubley, and D. Curwen. 1964. Yield, vigor, maturity, quality, and leaf composition of sweet corn as influenced by differential fertilizer treatments. Penn. Agri. Expt. Sta. Bull. 710.
Smith, C. B. 1984. Sweet corn growth responses and leaf concentrations as affected by lime types and fertilizer treatments in a five-year study. J. Amer. Soc. Hort. Sci. 109:572-577. https://doi.org/10.21273/JASHS.109.4.572
Smittle, D. A., E. D. Threadgill, and W. E. Seigler. 1981. Sweet corn growth, yield, and nutrient uptake responses to tillage systems. J. Amer. Soc. Hort. Sci. 106:49-53. https://doi.org/10.21273/JASHS.106.1.49
Smittle, D. A. 1985. Sweet corn response to nitrogen source, nitrogen application methods and nitrapyrin. J. Fert. Issues 2:53-57.
Swiader, J. M., and J. M. Gerber. 1984. Seedling growth and nutrition status in early sweet corn in relation to phosphorus and zinc under controlled low temperatures. J. Amer. Soc. Hort. Sci. 109:535-539. https://doi.org/10.21273/JASHS.109.2.150
Swiader, J. M., P. S. Mullen, and R. L. Becker. 1985. Nitrogen fertilization, nitrapyrin, and banded P on early sweet corn in sandy soil. Ill. Veg. Res. Rept.
Taber, H. G., and L. E. Peterson. 1979. Effect of nitrogen source and nitrapyrin on sweet corn. HortScience 14:34. https://doi.org/10.21273/HORTSCI.14.1.34
White, J. M., R. V. Tyson, E. A. Hanlon, G. J. Hochmuth, C. A. Neal. 1996. Plant petiole sap testing for nitrogen and potassium in sweet corn grown on mineral soil. Proc. Fla. State Hort. Soc. 109:149-151.
Woodruff, J. R., and H. L. Musen. 1985. Corn yield response to starter fertilizer and sulfur in a coastal plain soil. J. Fert. Issues 2:47-52.
Sweet Potato
Hammett, L. K., C. H. Miller, W. H. Swallow, and Christel Harden. 1984. Influence of N source, N rate, and K rate on the yield and mineral concentration of sweet potato. J. Amer. Soc. Hort. Sci. 109:294-298. https://doi.org/10.21273/JASHS.109.3.294
Leonard, O. A., W. S. Anderson, and M. Gieger. 1949. Field studies on the mineral nutrition of the sweet potato. Proc. Amer. Soc. Hort. Sci. 53:387-392.
Mascianica, M. P., R. R. Bellinder, B. Graves, R. D. Morse, and H. Talleyrand. 1985. Forecasting of N fertilizer requirements for sweet potatoes. J. Amer. Soc. Hort. Sci. 110:358-361. https://doi.org/10.21273/JASHS.110.3.358
Nicholaides III, J. J., H. F. Chancy, H. J. Mascagni, Jr., L. G. Wilson, and D. W. Eaddy. 1985. Sweet potato response to K and P fertilization. Agron. J. 77:466-470. https://doi.org/10.2134/agronj1985.00021962007700030024x
Spencer, J. A., and N. Ahmad. 1967. Plant nutrient deficiencies and related tissue composition of the sweet potato. Agron. J. 59:59-62 https://doi.org/10.2134/agronj1967.00021962005900010018x
Walker, D. W., and W. R. Woodson. 1987. Nitrogen rate and cultivar effects on nitrogen and nitrate concentrations of sweet potato leaf tissue. Commun. Soil Sci. Plant Anal. 18:529-541. https://doi.org/10.1080/00103628709367839
Worley, R. E., and S. A. Harmon. 1974. Effect of substituting Na for K on yield, quality, and leaf analysis of sweet potatoes grown on Tifton loamy sand. HortScience 9(6):580-581. https://doi.org/10.21273/HORTSCI.9.6.580
Table Beet
Gupta, U. C., and J. A. Cutcliffe 1985. Boron nutrition of carrots and table beets grown in a boron deficient soil. Commun. Soil Sci. Plant Anal. 16:509-516. https://doi.org/10.1080/00103628509367623
Mack, H. J. 1979. Effects of row spacings, fertilizers, and harvest dates on table beets. J. Amer. Soc. Hort. Sci. 104:717-720. https://doi.org/10.21273/JASHS.104.5.717
Mack, H. J. 1989. Effects of nitrogen, boron, and potassium on boron deficiency, leaf mineral concentrations, and yield of table beets. Commun. Soil Sci. Plant Anal. 20:291-303. https://doi.org/10.1080/00103628909368083
Peck, N. H., and G. E. MacDonald. 1972. Plant response to concentrated superphosphate and potassium chloride fertilizers IV. Table Beet (Betavulgaris L.). Search Agriculture 2 (14) N.Y. State Agri. Expt. Sta., Geneva, NY.
Tomato
Carrijo, O. A. and G. Hochmuth. 2000. Tomato responses to preplant-incorporated or fertigated phosphorus on soils varying in Mehlich-1 extractable phosphorus. HortScience 35:67-72. https://doi.org/10.21273/HORTSCI.35.1.67
Elamin, 0. M., and G. E. Wilcox. 1985. Effect of magnesium fertilization on yield and leaf composition of tomato plants. J. Plant Nutr. 8:999-1012. https://doi.org/10.1080/01904168509363402
Hochmuth, G. J., E. A. Hanlon, P. R. Gilreath, and K. D. Shuler. 1991. Effects of K rates on yield of tomato at three commercial production sites. Soil Crop Sci. Soc. Fla. Proc. 50:169-172
Hochmuth, G., O. Carrijo, and K. Shuler. 1999. Tomato yield and fruit size did not respond to P fertilization of a sandy soil testing very high in Mehlich-1 P. HortScience 34:653-656. https://doi.org/10.21273/HORTSCI.34.4.653
Jones, U. S., and T. L. Jones. 1978. Influence of polyethylene mulch and magnesium salts on tomatoes growing an loamy sand. Soil Sci. Soc. Amer. Proc. 42:918-922. https://doi.org/10.2136/sssaj1978.03615995004200060018x
Karlen, D. L., C. R. Camp, and M. L. Robbins. 1985. Fresh market tomato response to N and K fertilization and water management practices. Commun. Soil Sci. Plant Anal. 16:71-81. https://doi.org/10.1080/00103628509367588
Locascio, S. J., J. G. A. Fiskell, and F. G. Martin. 1984. Nitrogen sources and combinations for polyethylene mulched tomatoes. Proc. Fla. State Hort. Soc. 97:148-150.
Locascio, S. J., and A. G. Smajstrla. 1990. Trickle-irrigated tomato as affected by water quantity and N and K application timing. Proc. Fla. State Hort. Soc.
Locascio, S. J., G. J. Hochmuth, S. M. Olson, R. C. Hochmuth, A. Csizinszky, and K. D. Shuler. 1997. Potassium source and rate for polyethylene-mulched tomatoes. HortScience 32:1204-1207. https://doi.org/10.21273/HORTSCI.32.7.1204
Locascio, S. J., G. J. Hochmuth, F. M. Rhoads, S. M. Olson, A. G. Smajstrla, and E. A. Hanlon. 1997. Nitrogen and potassium application scheduling effects on drip-irrigated tomato yield and leaf tissue analysis. HortScience 32:230-235. https://doi.org/10.21273/HORTSCI.32.2.230
Mason, S. C., and G. E. Wilcox. 1982. Nitrogen status evaluation of tomato plants. J. Amer. Soc. Hort. Sci. 107:483-486. https://doi.org/10.21273/JASHS.107.3.483
Miller, R. J., D. E. Rolston, R. S. Rauschkolb, and D. W. Wolfe. 1981. Labeled nitrogen uptake by drip-irrigated tomatoes. Agron. J. 73:265-270. https://doi.org/10.2134/agronj1981.00021962007300020006x
Persaud, N., S. J. Locascio, and C. M. Geraldson. 1977. Influence of fertilizer rate and placement and irrigation method on plant nutrient status, soil soluble salt, and root distribution of mulched tomatoes. Soil Crop Sci. Soc. Fla Proc. 36:121-125.
Rhoads, F. M., S. M. Olson, G. J. Hochmuth, and E. A. Hanlon. 1995. Yield and petiole-sap nitrate levels of tomato with N rates applied preplant or fertigated. Soil Crop Sci. Soc. Fla Proc. 55:9-12.
Rhue, R. D., and P. H. Everett. 1987. Response of tomatoes to lime and phosphorus on a sandy soil. Agron. J. 79:71-77. https://doi.org/10.2134/agronj1987.00021962007900010015x
Shuler, K., and G. Hochmuth. 1995. Field tests of phosphorus fertilization of tomato growing in high-P soils in Palm Beach County, Florida. Proc. Fla. State Hort. Soc. 108:227-232.
Wien, H. C., and P. L. Minotti. 1987. Growth, yield, and nutrient uptake of transplanted fresh-market tomatoes as affected by plastic mulch and initial nitrogen rate. J. Amer. Soc. Hort. Sci. 112:759-763. https://doi.org/10.21273/JASHS.112.5.759
Worley, R. E. 1976. Response of tomato to pH of a coastal plain soil. J. Amer. Soc. Hort. Sci. 101:460-462. https://doi.org/10.21273/JASHS.101.4.460
Turnip Greens
Brantley, B. B. 1961. Effects of source and level of nitrogen on the yield and nitrogen content of turnip greens. Proc. Amer. Soc. Hort. Sci. 77:503-507.
Watermelon
Bhella, H. S. 1988. Effect of trickle irrigation and black mulch on growth, yield, and mineral composition of watermelon. HortScience 23:123-125. https://doi.org/10.21273/HORTSCI.23.1.123
Elamin, O. M., and G. E. Wilcox. 1986. Manganese toxicity in watermelon plants as influenced by nitrogen form. J. Amer. Soc. Hort. Sci. 111(5):765-768. https://doi.org/10.21273/JASHS.111.5.765
Elamin O. M., and G. E. Wilcox. 1986. Effect of magnesium and manganese nutrition on watermelon growth and manganese toxicity. J. Amer. Soc. Hort. Sci. 111:588-593. https://doi.org/10.21273/JASHS.111.4.588
Elmstrom, G. W., and J. G. A. Fiskell. 1974. Watermelon yield response to supplemental magnesium. Soil Crop Sci. Soc. Fla. Proc. 33:222-223.
Elmstrom, G. W., J. G. A. Fiskell, and F. G. Martin. 1973. Nutrient distribution in soil and watermelon plant uptake: effect of fertilizer timing, rate, and placement. Soil Crop Sci. Soc. Fla. Proc. 32:154-158.
Hanlon, E. A., G. J. Hochmuth, and O. A. Diaz. 1991. Mehlich-1 soil-test calibration for watermelon: Cu, Zn, and Mn. Commun. Soil Sci. Plant Anal., 22: 2077-2087 https://doi.org/10.1080/00103629109368559
Hochmuth, G. J., and E. A. Hanlon. 1988. Mehlich-I soil-test calibration for watermelons: phosphorus and potassium. Soil Crop Sci. Soc. Fla. Proc. 48:40-43.
Hochmuth, G., and B. Hochmuth. 1992. Placement of N-P-K fertilizer for mulched, drip-irrigated watermelons. Suwannee Valley REC Report #92-20.
Hochmuth, G., E. Hanlon, and B. Hochmuth. 1992. Foliar nutritional sprays did not improve yields or grade of watermelons or potatoes. Univ. Fla. Suwannee Valley REC Report #92-26.
Hochmuth, G., E. Hanlon, and B. Hochmuth. 1992. Field-testing N recommendations for icebox and standard watermelons in north central Florida. Univ. Fla. Suwannee Valley REC Report #92-27.
Hochmuth, G. J., E. A. Hanlon, and J. Cornell. 1993. Watermelon phosphorus requirements in soils with low Mechlich-1 extractable phosphorus. HortScience 28:630-632. https://doi.org/10.21273/HORTSCI.28.6.630
Hochmuth, G., B. Hochmuth, and C. Vann. 1994. Watermelon responses to N and K fertilization - yield, leaf and petiole sap nutrient concentrations. Univ. Fla. Suwannee Valley REC Report #94-06.
Hochmuth, G., R. Hochmuth. 1995. Effects of K rates and proportion of K supplied from controlled-release K on watermelon. Univ. Fla. Suwannee Valley REC Report #95-9.
Hochmuth, G., R. Hochmuth, and C. Vann. 1996. Responses of watermelon yield and whole leaf and petiole sap N seasonal profiles to N fertilization. Univ. Fla. Suwannee Valley REC Report #96-2.
Hochmuth, G., R. Hochmuth, and C. Vann. 1996. Responses of watermelon yield and whole leaf and petiole sap K seasonal profiles to K fertilization. Univ. Fla. Suwannee Valley REC Report #96-4.
Locascio, S. J. and G. J. Hochmuth. 2002. Watermelon production as influenced by lime, gypsum, and potassium. HortScience 37:322-324. https://doi.org/10.21273/HORTSCI.37.2.322
Locascio, S. J., and J.G.A. Fiskell. 1966. Copper requirements of watermelon. Proc. Amer. Soc. Hort. Sci. 90:568-575.
Locascio, S. J., P. H. Everett, and J. G. A. Fiskell. 1968. Effects of phosphorus sources and copper rates on watermelons. Proc. Amer. Soc. Hort. Sci. 92:583-589.
Locascio, S. J., J. G. A. Fiskell, and H. W. Lundy. 1973. Watermelon response to sulfur-coated urea, mulches, and nitrogen rates. Proc. Fla. State Hort. Soc. 86:201-204.
Sundstrom, F. J., R. L. Edwards, R. J. Constantin, and D. W. Wells. 1983. Influence of soil acidity on watermelon leaf tissue mineral concentration and yield. J. Amer. Soc. Hort. Sci. 108:734-736. https://doi.org/10.21273/JASHS.108.5.734
Mobility of essential elements in plants. Mobility reflects the ability of an element to be relocated within the plant under deficient supply.
Partial listing of commercial laboratories offering agricultural testing services to Florida growers. Not all laboratories offer all services. Some laboratories do not provide interpretations or recommendations with test results. Clients should contact the laboratory before submitting samples. This listing does not imply a recommendation of these laboratories by the authors or UF/IFAS.
Nitrate-nitrogen (and potassium) quick-test kits for use in petiole sap nitrate-N (and potassium) determinations.
Adequate nitrate-N and K concentrations in fresh petiole sap of most recently matured leaves for several vegetable crops at various periods in the season using the Hach or Quant-strip methods, or Cardy meter.
Critical (deficiency) values, adequate ranges, high values, and toxicity values for macronutrients for vegetables (most-recently-matured whole leaf plus petiole (MRM leaf) unless otherwise noted).
Critical (deficiency) values, adequate ranges, high values, and toxicity values for macronutrients for vegetables (most-recently-matured whole leaf plus petiole (MRM leaf) unless otherwise noted).
Critical (deficiency) values, adequate ranges, high values, and toxicity values for macronutrients for vegetables (most-recently-matured whole leaf plus petiole (MRM leaf) unless otherwise noted).
Critical (deficiency) values, adequate ranges, high values, and toxicity values for macronutrients for vegetables (most-recently-matured whole leaf plus petiole (MRM leaf) unless otherwise noted).
Critical (deficiency) values, adequate ranges, high values, and toxicity values for macronutrients for vegetables (most-recently-matured whole leaf plus petiole (MRM leaf) unless otherwise noted).
Critical (deficiency) values, adequate ranges, high values, and toxicity values for macronutrients for vegetables (most-recently-matured whole leaf plus petiole (MRM leaf) unless otherwise noted).
Critical (deficiency) values, adequate ranges, high values, and toxicity values for macronutrients for vegetables (most-recently-matured whole leaf plus petiole (MRM leaf) unless otherwise noted).
Critical (deficiency) values, adequate ranges, high values, and toxicity values for macronutrients for vegetables (most-recently-matured whole leaf plus petiole (MRM leaf) unless otherwise noted).
Critical (deficiency) values, adequate ranges, high values, and toxicity values for macronutrients for vegetables (most-recently-matured whole leaf plus petiole (MRM leaf) unless otherwise noted).
Critical (deficiency) values, adequate ranges, high values, and toxicity values for macronutrients for vegetables (most-recently-matured whole leaf plus petiole (MRM leaf) unless otherwise noted).
Critical (deficiency) values, adequate ranges, high values, and toxicity values for macronutrients for vegetables (most-recently-matured whole leaf plus petiole (MRM leaf) unless otherwise noted).
Critical (deficiency) values, adequate ranges, high values, and toxicity values for macronutrients for vegetables (most-recently-matured whole leaf plus petiole (MRM leaf) unless otherwise noted).
Critical (deficiency) values, adequate ranges, high values, and toxicity values for micronutrients for vegetables (most-recently-matured whole leaf plus petiole (MRM leaf) unless otherwise noted).
Critical (deficiency) values, adequate ranges, high values, and toxicity values for micronutrients for vegetables (most-recently-matured whole leaf plus petiole (MRM leaf) unless otherwise noted).
Critical (deficiency) values, adequate ranges, high values, and toxicity values for micronutrients for vegetables (most-recently-matured whole leaf plus petiole (MRM leaf) unless otherwise noted).
Critical (deficiency) values, adequate ranges, high values, and toxicity values for micronutrients for vegetables (most-recently-matured whole leaf plus petiole (MRM leaf) unless otherwise noted).
Critical (deficiency) values, adequate ranges, high values, and toxicity values for micronutrients for vegetables (most-recently-matured whole leaf plus petiole (MRM leaf) unless otherwise noted).
Critical (deficiency) values, adequate ranges, high values, and toxicity values for micronutrients for vegetables (most-recently-matured whole leaf plus petiole (MRM leaf) unless otherwise noted).
Critical (deficiency) values, adequate ranges, high values, and toxicity values for micronutrients for vegetables (most-recently-matured whole leaf plus petiole (MRM leaf) unless otherwise noted).
Critical (deficiency) values, adequate ranges, high values, and toxicity values for micronutrients for vegetables (most-recently-matured whole leaf plus petiole (MRM leaf) unless otherwise noted).
Critical (deficiency) values, adequate ranges, high values, and toxicity values for micronutrients for vegetables (most-recently-matured whole leaf plus petiole (MRM leaf) unless otherwise noted).
Critical (deficiency) values, adequate ranges, high values, and toxicity values for micronutrients for vegetables (most-recently-matured whole leaf plus petiole (MRM leaf) unless otherwise noted).