Introduction
Ocean acidification (OA) refers to the ongoing decrease in ocean pH, which is caused primarily by the oceanic uptake of excess carbon dioxide (CO2) from the atmosphere. Climate change and related impacts such as sea level rise, coastal flooding, and extreme weather events often receive more attention than OA; however, the acidification of the Earth's ocean is well documented and is a major concern for the marine science community. This publication is the introduction to a series that will address ocean acidification in Florida. It is a general explanation of the changes that are occurring to the chemistry of coastal and oceanic waters because of elevated carbon dioxide levels. Additional publications will focus on potential environmental, economic, and social implications of OA for Florida.
The Cause—Carbon Dioxide Emissions
Greenhouse gases (GHGs) regulate the Earth's temperature. They do this by absorbing and releasing heat energy from the Earth's surface, atmosphere, and clouds. GHGs get their name because they warm the Earth's atmosphere in the same way air is warmed in a greenhouse building (IPCC 2007). One could also think of these gases as a blanket around the earth. Without GHGs, our planet would be inhospitably cold. However, in the past 150 years, human activities have greatly increased the levels of GHGs entering the atmosphere (Heede 2014). For the past 150 years, the use of fossil fuels has been responsible for an estimated 2/3 of the human-generated CO2 in our atmosphere. Altered land use, such as deforestation, is responsible for the other 1/3. While there are many greenhouse gases, human activities have resulted in the buildup of four long-lived ones: carbon dioxide (CO2), methane (CH4), nitrous oxide (N2O), and fluorinated gases (which include many man-made gases used in industrial applications) (Figure 1; IPCC 2014) . CO2 is important because of its long-life, warming influence and, most importantly, the large amount of it in our atmosphere.
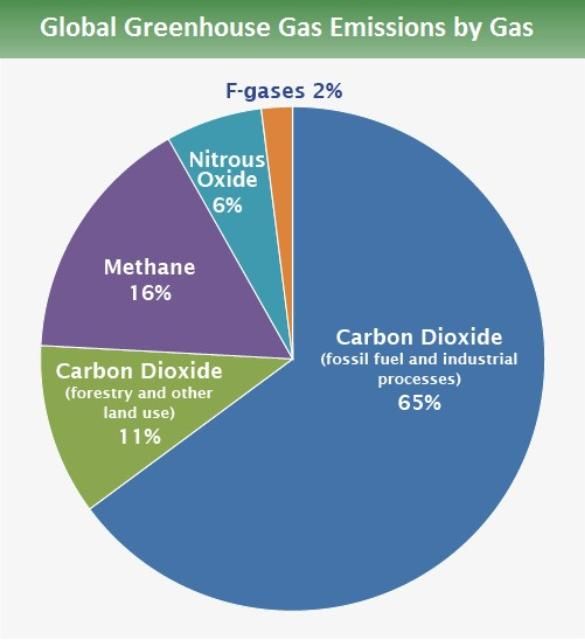
Credit: IPCC (2014)
For 650,000 years before the Industrial Revolution, atmospheric levels of CO2 fluctuated between 180 and 300 parts per million (ppm). However, as of 2017, human activities have drastically increased the amount of CO2 in our atmosphere from pre-industrial values of 280 ppm to more than 400 ppm (Tans 2017). Over time, only about half of the additional CO2 from human activity remains in the atmosphere. The other half is absorbed by the ocean (~30%) and plants on land (~20%) (Le Quéré et al. 2016). Historically, CO2 was absorbed at the ocean surface at a rate similar to that of removal. Today, the rate of human-induced CO2 additions is faster than the geological processes that remove CO2. Each day, the ocean absorbs approximately 22 million metric tons of CO2, and, over the past two centuries, more than 400 billion tons of CO2 have been absorbed by the ocean. This is good news in terms of climatic impacts because without the oceanic uptake the atmospheric concentration of CO2 would be much higher than current levels. However, this uptake is not without impact because it also changes ocean chemistry and results in the acidification of the Earth's oceans (Figure 2).
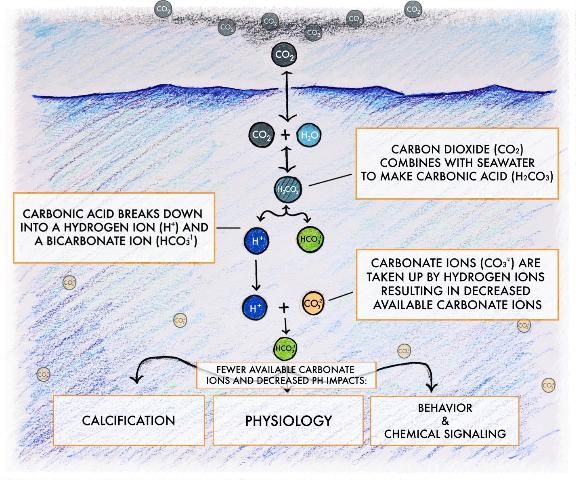
Credit: Joseph Henry, UF/IFAS
The Science of Ocean Acidification
When CO2 is dissolved in seawater, some of it will be used during photosynthesis by marine plants and phytoplankton, and some will react with the water to form carbonic acid, a weak, naturally occurring acid. This process is like that used in the creation of soda and other sparkling drinks. The addition of carbon to these beverages gives them the tiny bubbles and it's why they're described as "carbonated." When CO2 is absorbed by seawater, two things happen: 1) the seawater pH decreases (thus the term ocean acidification), and 2) the number of available carbonate ions decreases (Figure 2). Both of these occurrences will be discussed in this section.
pH is a chemistry measurement scale that expresses the acidity or alkalinity of a solution. Specifically, it is the measure of the hydrogen ion concentration of a solution. The pH scale ranges from zero to 14. A pH of seven is considered neutral. Values below seven are considered acidic. Those higher than seven are considered alkaline, or basic. The pH scale is logarithmic. Each one-unit change in the pH scale corresponds to a ten-fold change in hydrogen ion concentration.
The Earth's ocean water is naturally slightly alkaline (basic). The more carbon dioxide the ocean absorbs, the more carbonic acid is formed. Absorption of atmospheric CO2 over the past 150 years has made the ocean more acidic. According to the IPCC, the average ocean pH has decreased by about 0.1 units, from a pre-industrial revolution value of 8.2 to the current pH of 8.1 (IPCC 2014). Under present day emission conditions, average ocean pH could drop to 7.7–7.9 by the end of the century. The magnitude of a pH decrease from 8.1 to 7.7–7.9 can be misleading because the pH scale is logarithmic. Such a drop would represent a 100–150% increase in hydrogen ions (H+) in the water.
As with atmospheric CO2, variations in oceanic CO2 concentrations have occurred in the past. It is unlikely, however, that the oceans have ever experienced such a rapid and significant decrease in pH. The IPCC reports that the current rate of ocean acidification is unprecedented within the last 65 million years, and possibly as long as 300 million years (IPCC 2014). While most marine organisms have historically been able to adjust to changes in pH, the current accelerated rate of acidification may prove to be too fast for them to adapt. For other organisms, basic physiological processes may become chemically impossible.
The second chemical response to increases in CO2 is a decrease in carbonate ions (CO3-2) in seawater. When carbonic acid (H2CO3) dissolves in seawater, it breaks apart into a hydrogen ion (H+) and a bicarbonate ion (HCO3-) (Figure 2). Some of these hydrogen ions will remain in that state, thereby increasing the acidity of the water. The majority, however, will react with carbonate ions (CO3-2). This reaction creates additional bicarbonate ions and reduces the number of carbonate ions available to marine organisms. The degree to which seawater is saturated with carbonate ions is referred to as the "saturation state." A reduction in the concentration of carbonate ions will lead to a proportional decrease in the saturation state. It is estimated that by the end of this century, the concentration of carbonate ions could be reduced by half in some parts of the ocean. The reduction in carbonate ions and consequential decreased saturation state likely will have a significant impact on marine calcifying organisms such as corals, oysters, clams, and many others that build their shells through the uptake of carbonate ions from the water.
Conclusion
Further information on different aspects and potential consequences of OA will be available in additional EDIS publications in this series. The acidification of the Earth's ocean cannot be stopped entirely, but it can be slowed. The magnitude of its consequences can also be diminished. This can be done by limiting the amount of CO2 and greenhouse gas emissions that enter the atmosphere from human activities. The United States is the second leading country in carbon dioxide emissions due to the burning of fossil fuels. The United States also leads global per capita carbon emissions (tonnes of carbon per person per year), emitting more than double that of the second leading emitter, the collective member states of the European Union (Le Quéré et al. 2016).
Florida ranks 6th of the 50 states in total CO2 emissions because Florida's industrial sector consumption is relatively low. In Florida, the transportation and residential sectors are the main carbon emitters due to a large population and many visitors (United States Energy Information Administration 2017). This means that the people of Florida have the ability to greatly reduce the state's energy consumption by modifying their everyday actions. While changes are needed on a global scale, individuals can make an important difference, especially within Florida and the United States. New technologies and small changes can substantially minimize an individual's carbon footprint. To find tips and recommendations about what changes you can make in your home to help mitigate CO2 emissions, review the UF/IFAS Energy Efficient Homes series. Other individual-level strategies for reducing CO2 emissions are available in the Examples and Resources column of Table 1 in Strategies for Communicating Climate Change to Extension Audiences, https://edis.ifas.ufl.edu/publication/fr402, from the UF/IFAS Climate Change Communication series.
References
Challener, R. C., L. L. Robbins, and J. B. McClintock. 2016. "Variability of the carbonate chemistry in a shallow, seagrass-dominated ecosystem: implications for ocean acidification experiments." Marine and Freshwater Research 67: 163–172. https://doi.org/10.1071/MF14219
Fabry, V. J., B. A. Seibel, R. A. Feely, and J. C. Orr. 2008. "Impacts of ocean acidification on marine fauna and ecosystem processes." ICES Journal of Marine Science 65: 414–432. https://doi.org/10.1093/icesjms/fsn048
Gledhill, D. K., R. Wanninkhof, F. J. Millero, and M. Eakin. 2008. "Ocean acidification of the greater Caribbean region 19962006." Journal of Geophysical Research 113: C10031. https://doi.org/10.1029/2007JC004629
Heede, R. "Tracing anthropogenic carbon dioxide and methane emissions to fossil fuel and cement producers, 18542010." Climatic Change 122: 229–241.
IPCC. 2007. "Climate Change 2007: The Physical Science Basis." Contribution of Working Group I to the Fourth Assessment Report of the Intergovernmental Panel on Climate Change. Solomon, S., D. Qin, M. Manning, Z. Chen, M. Marquis, K. B. Averyt, M. Tignor, and H. L. Miller (eds.). Cambridge University Press, Cambridge, United Kingdom and New York, NY, USA: 996 pp. https://www.ipcc.ch/report/ar4/wg1/
IPCC. 2014. "Climate Change 2014: Mitigation of Climate Change." Working Group III Contribution to the Fourth Assessment Report of the Intergovernmental Panel on Climate Change. O. Edenhofer, R. Pichs-Madruga, and Y. Sokona (eds). Cambridge University Press, Cambridge, United Kingdom and New York, NY USA: 1419 pp. https://www.ipcc.ch/report/ar5/wg3/
Le Quéré, C., R. M. Andrew, J. G. Canadell, S. Stitch, J. I. Korsbakken, G. P. Peters, A. C. Manning, et al. 2016. "Global carbon budget 2016." Earth System Science Data 8: 605–649.
Royal Society. 2005. Ocean Acidification Due to Increasing Atmospheric Carbon Dioxide. Policy Document 12/05. London, England. https://royalsociety.org/~/media/Royal_Society_Content/policy/publications/2005/9634.pdf
Tans, P. 2017. Trends in Atmospheric Carbon Dioxide. NOAA/ESRL. https://gml.noaa.gov/ccgg/trends/
United States Energy Information Administration. 2017. Florida State Energy Profile Analysis. https://www.eia.gov/state/analysis.php?sid=FL
Yates, K. K., C. Dufore, N. Smiley, C. Jackson, and R. B. Halley. 2007. "Diurnal variation of oxygen and carbonate system parameters in Tampa Bay and Florida Bay." Marine Chemistry 104, 110–124. https://doi.org/10.1016/j.marchem.2006.12.008