This publication is one in a series on ocean acidification (OA). The series introduction, Ocean Acidification: An Introduction, contains information on the causes and chemistry of OA. Because OA is large-scale and complex, each publication in the series addresses a specific aspect of this issue. Florida, with an extensive coastline and deep cultural and economic ties to marine resources, will be directly affected by changes in seawater chemistry. Thus, each topic in the series also highlights information of specific relevance to Florida. While some of the chemistry can be a bit technical, the publications in the series should be accessible to the general public as well as interested specialists.
Introduction
Approximately 30% of the carbon dioxide (CO2) released into the atmosphere has been absorbed by the world’s oceans. As CO2 emissions increase due to human activities, so does the amount absorbed by the oceans. Carbon dioxide gas lowers the pH of the ocean system. The gas chemically reacts with water (H2O) yielding carbonic acid (H2CO3). Breakdown of H2CO3 into bicarbonate ions (HCO3-) and hydrogen ions (H+) causes OA as increased H+ reduces seawater pH. A subject of particular interest is the effects of OA on marine species that are economically important (i.e., products for human use) and ecologically important (i.e., nutrient cycling, reef builders). Sponges are important in both ways. They are of some economic importance because of the sponge fishery, and they are of vital ecological importance. Sponges are stationary marine invertebrates that are essential reef-associated species. They provide shelter for fish and crustaceans in reef habitats and can also structure ecosystems through bioerosion (biological processes that break down hard substrates), water filtration, and colonization of coral reefs. This publication considers the effects of OA on marine sponges, focusing on Florida’s coral reef.
Impacts on Sponges
Ocean acidification is not likely to affect all sponges in the same way. When considering the effects of OA on sponges, a distinction can be drawn between siliceous and calcareous sponges (Figure 1). The distinguishing feature between these sponges is the internal endoskeletal material that forms their sharp spicules, the interlocking spikes within the sponge tissue providing structural support. Siliceous sponges have spicules made of silica (glass), and calcareous sponges have spicules made of calcium carbonate (CaCO3).
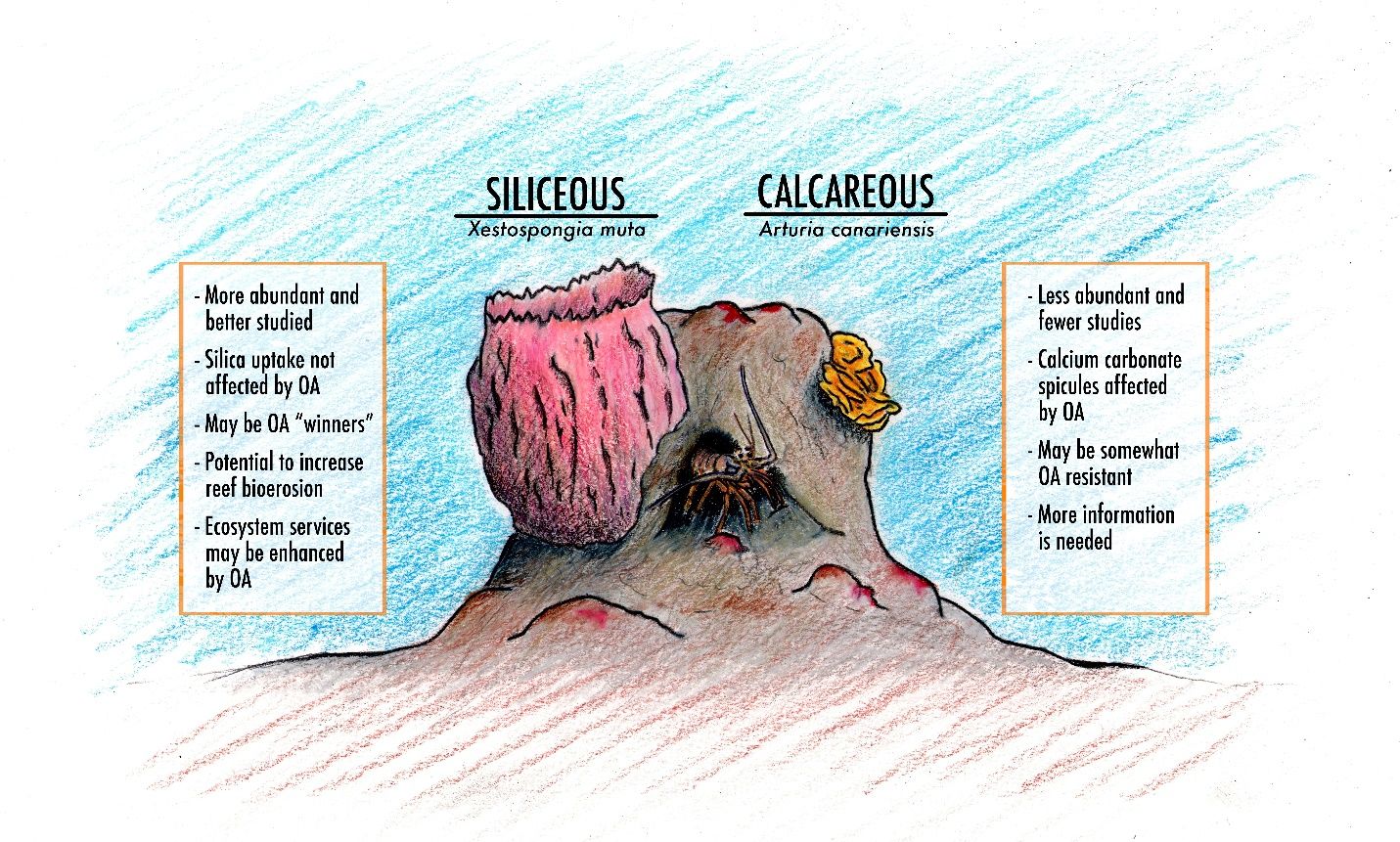
Credit: Joseph Henry
Siliceous Sponges
There are three classes of sponges, Demospongiae (demosponges), Homoscleromorpha (marine sponges), and Hexactinellida (glass sponges). The glass spicules vary in size ranging from microscopic (microscleres) to those visible to the naked eye (megascleres). Because these types of sponge are not reliant on calcification (see the Ask IFAS publication “Ocean Acidification: Calcifying Marine Organisms”), there is greater potential to resist the changing environmental conditions produced by OA (Bell et al. 2013). In fact, Bell et al. (2013) suggests that these sponges may come to dominate stony coral reef systems faced with rising CO2 levels and other climate change effects. This theory is based on the geological evidence that siliceous sponges thrived during a mass extinction event 200 million years ago when ocean waters became more acidic. Despite OA challenges, their spicule structure and other adaptations (e.g., photosynthesis of symbiotic dinoflagellates) could lead to an increased abundance of siliceous sponges.
The uptake of silica in siliceous sponges is not affected by OA, increased temperature, or the combined exposure to both stressors (Vincente et al. 2016). This means that the substance used to form their skeletons is not compromised by either of these environmental stressors. The growth rates and survival of siliceous sponges are also unaffected by OA or temperature. However, there is evidence that spicules decrease in size and weight, and therefore the total weight of spicules decreases under prolonged elevated CO2 exposure. This suggests that, while siliceous sponges may be better able to resist the effects of OA than sponges that rely on calcification, they may still be affected by these conditions. Even within demosponges, some species are probably more vulnerable than others to OA, because there are characteristics besides spicule formation (e.g., reproduction, growth rate, etc.) that are likely affected by pH (Goodwin et al. 2014). Although demosponges are predominantly siliceous sponges, there are some species in the class with calcareous elements that may increase their vulnerability.
A limited number of siliceous sponge species are important bioeroders on coral reef environments (e.g., boring sponges in the family Clionidae; Wisshak et al. 2013). These sponges have an adaptation to increase acidity in localized areas to aid in dissolving the carbonate skeleton of stony corals or marine bivalves and gastropods (Ramsby et al. 2018). As OA also increases dissolution rates of the carbonate skeletons of corals, the amount of energy needed for bioerosion to occur is reduced (Wisshak et al. 2012; Achlatis et al. 2017). These sponges can break down corals faster and exploit the new space available in the reef system because of decreased competition (Bell et al. 2013). Increased boring by clionid sponges and other climate change effects on coral abundance may have consequences for coral reef habitats.
Symbiosis is a term referring to a beneficial relationship between two species. Like corals, siliceous sponges have a symbiotic relationship with dinoflagellates (unicellular microalgae), specifically those in the genus Symbiodinium. The sponges protect their symbionts; the dinoflagellate symbiont can burrow deeper into the sponge during darkness or exposure to stressors (Schönberg and Suwa 2007). In return, the Symbiodinium provide food for the sponge, through photosynthesis (Bell et al. 2013; Wisshak et al. 2013). An increase in CO2 may lead to increased photosynthesis. The energy provided by these microalgae can exceed the requirements of the sponges, thereby increasing the overall health and fitness (Achlatis et al. 2013; Bell et al. 2013). With increased energy availability from these endosymbionts, competitive behavior, and lack of reliance on calcification, siliceous sponges may be better adapted for a more acidic environment than corals.
Bioerosion processes, plus survivability, are also affected by temperature. Increased temperature harms these siliceous sponges and can initiate stress responses in some species (Wisshak et al. 2013; Stubler et al. 2014). Consequently, even if these sponges are adapted to OA, they may face other challenges and interactive effects that require further investigation. Nevertheless, it is still possible that these sponges could outcompete and dominate coral reef systems in the future.
Calcareous Skeletons
A small percentage of marine sponge species have calcium carbonate (CaCO3) spicules. These are termed calcareous sponges. Sponges in class Calcarea have calcium carbonate, needle-like spicules that compose their skeleton (Smith et al. 2013, Figure 2). Sponges with CaCO3 skeletons account for less than 3% of all sponge species (Bell et al. 2013; Smith et al., 2013). They are generally small and cryptic, although much of their distribution occurs within coral reef zones.
Ocean acidification results in the decrease of carbonate ions, which could lead to the breakdown of these skeletons (Patterson and Krimsky 2018). Consequently, we can hypothesize that calcareous sponges will be more vulnerable to changing climate conditions. However, limited studies evaluating OA effects on calcareous sponges do not support this prediction.
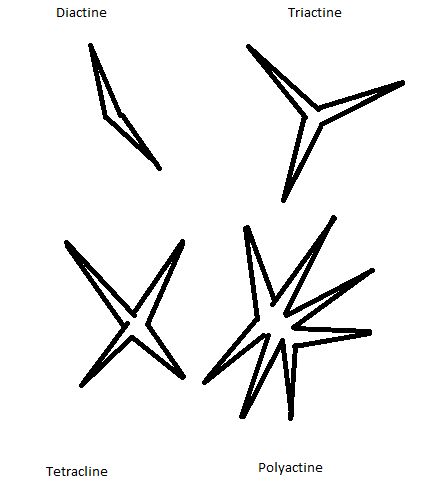
Credit: Lena Donnarumma
Understanding carbonate mineralogy in calcareous sponges and how the structure is affected by OA is vital (Smith et al. 2013). The crystal structure of the carbonate mineral may play a role in solubility, or dissolvability, in seawater, of the sponge skeleton. Calcite is a more stable form of CaCO3, and it is less soluble than another form called aragonite, which makes up coral skeletons. However, some sponges produce MgCO3 (magnesium carbonate), or high-Mg calcite, which is more soluble than aragonite (Smith et al. 2013). This distinction suggests that sponges with the MgCO3 form may experience more harmful effects of high OA due to the composition and solubility of their skeletons.
Although there has been no direct evidence to suggest calcareous sponges will succumb to OA, Peck et al. (2015) found that reduced pH over 100 days affected a marine biofouling community, including a tubeworm, sea squirts, and a calcareous sponge. Interestingly, the calcareous sponge, from the genus Leucosolenia, experienced increased abundance in low-pH conditions. Interactions of Leucosolenia with the rest of the biofouling community (e.g., reduced competition from other organisms) may have been partially responsible for this increase, suggesting that its adaptations to environmental stressors may differ from those of other sponge species.
A separate study tested the effects of both elevated temperature and decreased pH on a single calcareous sponge species, Sycettusa hastifera. Ribeiro et al. (2020) found that, overall, this species was relatively resistant to these stressors and may, in fact, have a low vulnerability to OA. However, reduced pH resulted in smaller spicules in the outermost sponge layers. Sponge-associated microbe communities were stable under both stressors, although there were increases in stress-indicator bacteria. This study suggests that calcareous sponges may be able to resist OA in short-term (9 day) exposure. Still, it does not provide data on potential long-term effects of OA.
Translating OA Effects on Sponges
More study is needed to better understand the effects of OA on calcareous sponges, especially with the prediction that they are more at risk. Ocean acidification research is a recent and rapidly evolving field, and this research will require further fine tuning and more precise methodologies to better explain the challenges faced by all organisms. However, the available information should be considered to better understand how OA might affect sponge species and coral reef ecosystems.
Relevance to Florida
Florida’s coral reef is a valuable ecological and economic resource. It provides important habitats for fishery species and shoreline protection and is essential to the ecotourism industry (Florida Museum 2023). According to the Florida Keys National Marine Sanctuary (FKNMS), coral reefs in southeast Florida have a total economic value of about $8.5 billion. The estimated commercial value of fisheries associated with coral reef systems is over $100 million.
It is imperative to consider the effects OA has on sponges and stony corals because the interactions between these two groups may be altered. Small, cryptic sponge species growing in close association with stony corals may be considered a “glue” that helps hold broken fragments, known as rubble, together. Sponges can represent more than half of the organisms growing in coral rubble, bringing stability to these areas, and ultimately facilitating coral recruitment (Wolfe et al. 2021). In a coral reef restoration experiment, Biggs (2013) observed a positive relationship between sponges and corals. When transplanting coral fragments to reef systems, the establishment of the repopulated corals depends on the substrate. The bioeroding activities of sponges produce a natural substratum that encourages the recruitment of corals. This breakdown increases the net carbonate available for coral development. Under normal oceanic conditions, sponges may facilitate coral restoration efforts (Butler et al. 2021).
However, with habitats affected by OA-accelerated bioerosion activities may alter this balance. Evidence suggests that siliceous sponges may dominate coral reefs through accelerated breakdown of corals (Page et al. 2021). Additionally, Page et al. (2021) observed that the increased presence of sponges may facilitate the growth of non-calcifying macroalgae, which can outcompete stony corals and impede their growth and reproduction. This increase would further affect the community structure, which could impact fisheries and food security, ecotourism, and other ecosystem services coral reef systems provide.
Conclusion
This publication discussed the effects of OA on different types of marine sponges. At this time, a limited amount of available data suggests that both calcareous and siliceous sponges may be OA “winners” in the sense of being resistant or at least relatively less susceptible to the stressor of decreasing pH than some competing marine organisms. Additional long-term studies would better illuminate the effects of OA on sponges, especially calcareous sponges. Slowing the progression of OA would minimize overall impacts on coral reef ecosystems to maintain the complex balance between sponges and corals and may reduce the rate at which sponges have the potential to outcompete stony corals.
References
Achlatis, M., R. M. van der Zande, C. H. L. Schönberg, J. K. H. Fang, O. Hoegh-Guldberg, and S. Dove. 2017. “Sponge Bioerosion on Changing Reefs: Ocean Warming Poses Physiological Constraints to the Photosymbiotic Excavating Sponge.” Scientific Reports 7:10705. https://doi.org/10.1038/s41598-017-10947-1
Bell, J. J., S. K. Davy, T. Jones, M. W. Taylor, and N. S. Webster. 2013. “Could some coral reefs become sponge reefs as our climate changes?” Global Change Biology 19:2613–2624. https://doi.org/10.1111/gcb.12212
Biggs, B. C. 2013. “Harnessing Natural Recovery Processes to Improve Restoration Outcomes: An Experimental Assessment of Sponge-Mediated Coral Reef Restoration.” PLoS ONE 8 (6): e64945. https://doi.org/10.1371/journal.pone.0064945
Butler, J., W. C. Sharp, J. H. Hunt, and M. J. Butler IV. 2021. “Setting the Foundation for Renewal: Restoring Sponge Communities Aids the Ecological Recovery of Florida Bay.” Ecosphere 12 (12): e03876. https://doi.org/10.1002/ecs2.3876
Goodwin, C., R. Rodolfo-Metalpa, B. Picton, and J. M. Hall-Spencer. 2014. “Effects of Ocean Acidification on Sponge Communities.” Marine Ecology 35:41–49. https://doi.org/10.1111/maec.12093
Florida Keys National Marine Sanctuary. “The Coral Reef Economy” Accessed May 23, 2023. https://oceanservice.noaa.gov/news/oct17/coral-economy.html
Florida Museum. “Importance of Coral Reefs.” Accessed May 23, 2023. https://www.floridamuseum.ufl.edu/southflorida/habitats/corals/importance/
Page, H. N., C. Hewett, H. Tompkins, and E. R. Hall. 2021. “Ocean Acidification and Direct Interactions Affect Coral, Macroalga, and Sponge Growth in the Florida Keys.” Journal of Marine Science and Engineering 9:739. https://doi.org/10.3390/jmse9070739
Patterson, J., and L. Krimsky. 2018. “Ocean Acidification: An Introduction: FA206, 7/2018”. EDIS 2018 (4). Gainesville, FL. https://doi.org/10.32473/edis-fa206-2018
Peck, L. S., M. S. Clark, D. Power, J. Reis, F. M. Batista, and E. M. Harper. 2015. “Acidification Effects on Biofouling Communities: Winners and Losers.” Global Change Biology 21:1907–1913. https://doi.org/10.1111/gcb.12841
Ramsby, B. D., M. O. Hoogenboom, H. A. Smith, S. Whalan, and N. S. Webster. 2018. “The bioeroding sponge Cliona orientalis will not tolerate future projected ocean warming.” Scientific Reports 8:8302. https://doi.org/10.1038/s41598-018-26535-w
Ribeiro, B., A. Padua, A. Barno, H. Villela, G. Duarte, A. Rossi, F. da Costa Fernandes, R. Peixoto, and M. Klautau. 2020. “Assessing Skeleton and Microbiome Responses of a Calcareous Sponge under Thermal and pH Stresses.” ICES Journal of Marine Science 78 (3): 855–866. https://doi.org/10.1093/icesjms/fsaa231
Schönberg, C. H. L., and R. Suwa. 2007. “Why Bioeroding Sponges May Be Better Hosts for Symbiotic Dinoflagellates Than Many Corals.” In Porifera Research: Biodiversity, Innovation and Sustainability, edited by M. R. Custódio, E. Hajdu, G. Lôbo-Hajdu, and G. Muricy, 569–580. Rio de Janeiro: Publication Museum Nactional.
Smith, A. M., J. Berman, M. M. Key Jr., and D. J. Winter. 2013. “Not all sponges will thrive in a high-CO2 ocean: review of mineralogy of calcifying sponges.” Palaeogeography, Palaeoclimatology, Palaeoecology, 392:463–472. https://doi.org/10.1016/j.palaeo.2013.10.004
Stubler, A. D., B. T. Furman, and B. J. Peterson. 2014. “Effects of pCO2 on the Interaction between an Excavating Sponge, Cliona varians, and A Hermatypic Coral, Porites furcata.” Marine Biology 161:1851–1859. https://doi.org/10.1007/s00227-014-2466-y
Vincente, J., N. J. Silbiger, B. A. Beckley, C. W. Raczkowski, and R. T. Hill. 2016. “Impact of High pCO2 and Warmer Temperatures on the Process of Silica Biomineralization in the Sponge Mycale grandis.” ICES Journal of Marine Science 73 (3): 704–714. https://doi.org/10.1093/icesjms/fsv235
Wisshak, M., C. H. L. Schönberg, A. Form, and A. Freiwald. 2012. “Ocean Acidification Accelerates Reef Bioerosion.” PLoS ONE 7 (9): e45124. https://doi.org/10.1371/journal.pone.0045124
Wisshak, M., C. H. L. Schönberg., A. Form, and A. Freiwald. 2013. “Effects of Ocean Acidification and Global Warming on Reef Bioerosion—Lessons from a Clionaid Sponge.” Aquatic Biology 19:111–127. https://doi.org/10.3354/ab00527
Wolfe, K., T. M. Kenyon, and P. J. Mumby. 2021. “The Biology and Ecology of Coral Rubble and Implications for the Future of Coral Reefs.” Coral Reefs 40:1769–1806. https://doi.org/10.1007/s00338-021-02185-9